Gerbode defects are rare Ventricular Septal Defects (VSD) constituting approximately one percent cases of congenital heart diseases. The genetic predispositions towards the Gerbode Defect (GD) have remained an unexplored area of study till date. We investigated the genotype-phenotype correlation in patients with Gerbode VSD. Molecular genetic study on Sanger sequencing and subsequent data analysis showed that the contributing sequence variations in the NKX2-5, GATA4 and TBX5 gene lies in one of the highly conserved regions and this region is responsible for encoding a functional protein. The resulting genotype variation may be responsible for causing the diseased phenotype known as GD.
Echocardiography,Single nucleotide polymorphism,Transcriptional gene(s).
VSD is the most common defect that occurs as a primary anomaly, with or without associated major cardiac defects [1]. Gerbode defect, a rare VSD “left ventricular-to-right atrial shunt” accounts for1% in congenital heart diseases [2]. The diagnosis of an Left Ventricle-Right Atrial (LV-RA) shunt is always challenging due to the limited diagnostic accuracy of 2D Echocardiography [3]. GD results in either congenital or acquired defect where congenital GD result from an indirect shunt i.e., intravalvular via perimembranous ventricular septum and the acquired GD results from direct shunt i.e., supravalvular via membranous ventricular septum [4,5]. Acquired GD have been described as a complication related to bacterial endocarditis, chest injuries, and myocardial infarctions and Congenital GD (CGD) follows a complex developmental process and is caused by multiple factors [6]. In some individuals, smaller defects gradually close on their own by the process of an aneurysm occurring by means of hyperplasia of connective tissue covering the leaflets of tricuspid valve touching the margin of the defect [7]. In due course of time, failure in closing may lead to other complications. Despite major advancements in the field of genetic analysis, there is still a lack of consensus among investigators regarding the number of genes responsible for the rare phenotypes such as CGD.
The septum formation is an important milestone in the heart morphogenesis, which is highly orchestrated by transcriptional factor genes mainly NKX2-5, GATA4, and TBX5 [8]. Several mutations in these transcription factor genes have been reported to lead to the cardiac septal defects [9]. The specific factors leading to complex VSDs remain unidentified till date. Understanding the aetiology and risk factors behind CGD, considering its multifactorial nature, becomes very important for assessing the implications of the defect [10,11]. Various studies have shown that the VSDs could be related to the sequence variations in patients and related families [12–14]. Through the present case series, we tried to find a correlation between the sequence variations in the NKX2-5, GATA4 and TBX5 genes and the role of these variations in causing GD. For this purpose, affected individuals with cardiac septal defects were assessed using two dimensional Transoesophageal Echocardiography (2DE-TEE) with colour flow Doppler and studied the three genes using automated DNA sequencing [15], so we sequenced the exon region along with the intron-exon boundaries of the subjected genes in three cases to explore the pathogenicity of the GD.
Case 1
A 31-year-old asymptomatic male with 7 mm moderate sized perimembranous VSD showing mild Tricuspid Regurgitation and moderate Pulmonary Arterial Hypertension. The echocardiography of the patient is represented in [Table/Fig-1]. The left to right shunt was found to be directed to Right Atrium (RA) through the tricuspid valve. Also, mildly dilated cardiac chambers with a forming aneurysm were observed to direct the VSD jet into RA and Right Ventricle (RV). On comprehensive mutation screening of all the coding exons and flanking introns of NKX2-5GATA4 and TBX5, we found the changes in NKX2-5, GATA4 and TBX5 genes to be as: 1) Two single nucleotide polymorphisms c.63A>G (exon 1) and c.543G>A (exon 2) of NKX2-5 gene; 2) intronic SNP, g.79862C>T in the intron-exon boundaries of exon6 of GATA4 gene; 3) c.215C>A (exon 3) of TBX5 gene in this patient. Localization of sequence variants irrespective of conserved domains is represented in [Table/Fig-2a].
Transthoracic echocardiography of the patient (Case 1) showing Indirect Gerbode VSD: a) Parasternal long axis view showing VSD with the aneurysmal formation. b) Apical four-chamber view with colour flow mapping showing VSD jet to RA and RV.
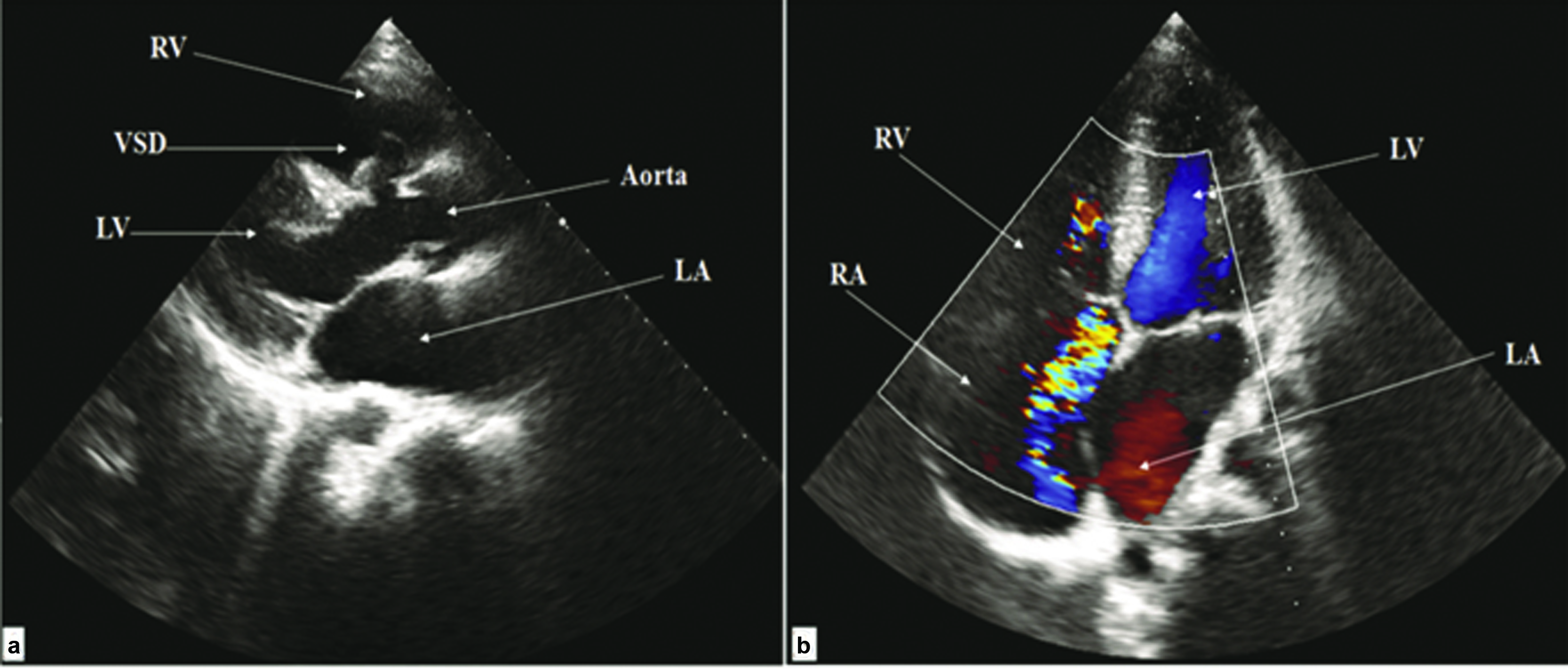
A schematic representation of NKX2-5 and GATA4 sequence variants. A) Conserved domains of NKX2-5, GATA4 and TBX5 showing the location of sequence variants; a) NKX2-5 protein indicates Tinman domain (TN), Homeobox Domain (HD) and NK domain; b) GATA4 protein indicates Transactivation Domains (TAD), N-terminal zinc finger (NZf), C-terminal zinc finger (CZf), and nuclear localization signal (NLS); c) TBX5 protein domains indicates DNA-binding domain (DNA-BD), transactivation domains (TAD and nuclear localization signal (NL) B) Sequence Chromatograms of NKX2-5, GATA4, and TBX5, arrows indicates the heterozygous nucleotides. a) c.63A>G, b) c.543G>A of NKX2-5; c) c.419C>T d) c.1138G>A e) g.79862C>T of GATA4 and f) c.215C>A g) c.837A>G of TBX5. C) ConSurf analysis representing Multiple Sequence Alignment across different species showing the conserved and variable regions of the variants; a) NKX2-5;b) GATA4 and c) TBX5.
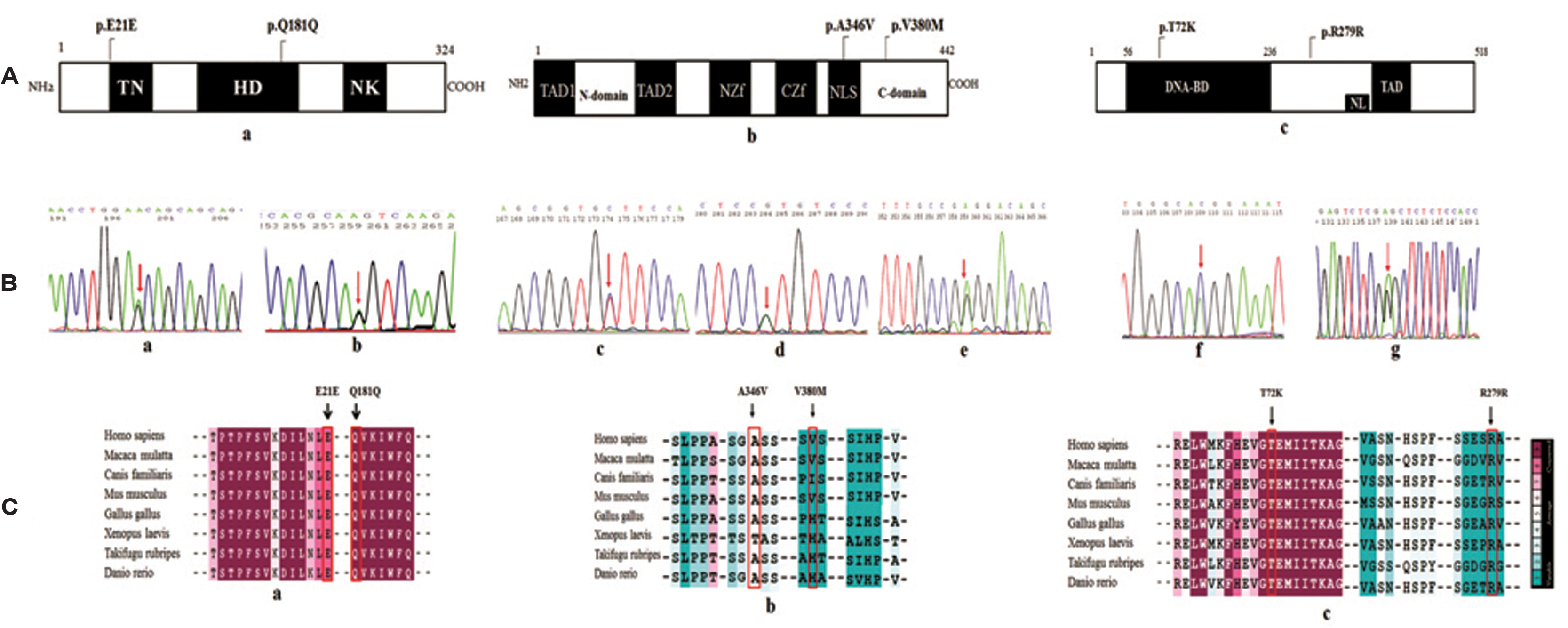
Case 2
A 27-year-old female having a complaint of murmur and 4 mm small perimembranous VSD associated right atrial dilation and mild to moderate Pulmonary Stenosis (PS). Further, it was observed that the colour flow jet was directed from RV into RA with 110 mmHg pressure gradients. Upon mutation analysis, we found the sequence variants of NKX2-5, GATA4 and TBX5 gene depicted in [Table/Fig-2b] are: 1) two synonymous SNPs in NKX2-5 (c.63A>G; c.543G>A), non-synonymous variant (c.419C>T); 2) intronic variant (g.79862C>T) in the intron-exon boundaries of exon6 of GATA4; 3) mutation c.215C>A (exon 3) and a polymorphism; c.837A>G (exon 8) in TBX5 gene, in this patient.
Case 3
A 55-year-old female having a fever with severe palpitations and with no cardiac complaints, diagnosed with 5 mm perimembranous Gerbode VSD along with moderate Pulmonary arterial hypertension. Transthoracic and Transoesophageal echocardiography further confirmed the presence of intracardiac shunt from Left Ventricle (LV) to RA. The Doppler gradient was 130 mmHg in right atrium with an aneurysmal formation which was confirmed by sonography. On mutation screening, we found the changes in NKX2-5, GATA4 and TBX5 genes to be: 1) two synonymous variants in NKX2-5 (c.63A>G; c.543G>A); 2) two non-synonymous variants (c.419C>T; c.1138G>A) and an intronic variant (g.79862C>T) in the intron-exon boundaries of exon6 of GATA4, and 3) polymorphism, c.837A>G (exon 8) in TBX5 gene, in this patient.
The cross species alignment of NKX2-5, GATA4 and TBX5 protein [Table/Fig-2c] showed that the amino acid changes belong to an evolutionarily conserved region which highlights the functional importance of the amino acid. However, the mutant residue was not observed in other homologous sequences suggesting the mutation to be potentially damaging to the protein function. The details of the exonic position, location of sequence variations in the secondary structure and prediction by in silico analysis are represented in [Table/Fig-3]. The structural analysis and protein modeling revealed that the wild type residue A346 to be located at Nuclear Localization Signal (NLS), and V380 at C-domain of GATA4. Upon mutation, both A346 and V380 are likely to make new hydrogen bond (H-bond) interaction i.e., A346 upon mutation converted to V346, makes 3 new H-bonds with Q316. This mutation has a peptide backbone with GLN316. Two H-bonds are formed between peptide backbone O of V346 with NE2 side chain and N of Q316 and third H-bond is between N of V346 and O of G316 [Table/Fig-4a]. In the proper conformation of V380, the bond is held with S379 and S381. However, upon mutation to M380, H-bond forms between N of M380 and O of S377 and between O of M380 and O side chain of S381 [Table/Fig-4b]. In the case of T72K, the wild type residue is located at T-box domain and consists of a DNA binding site. The interaction between T72 and I212 is relatively stronger than its interaction with P67 because of a H-bond. Mutation to K72 sterically hinders the K72 having a backbone of H68 and O backbone of W64, which results in structural and conformational changes in the protein [Table/Fig-4c].
Genetic variants and their location in the secondary structure of protein associated with Gerbode defect patients.
Gene Name | Chr. Loci | Exons | Genomic change | cDNA change | Allele change | Protein change | Amino acid change | RS/HGMD ID | Start | End | Helix | Strand | Coil | Case 1 | Case 2 | Case 3 |
---|
NKX2-5 | 5 | Exon1 | g.337A>G | c.63A>G | GAA ⇒ GAG | p.E21E | E [Glu] ⇒ E [Glu] | rs2277923 | 172662023 | 172662025 | + | - | - | + | + | + |
5 | Exon 2 | g.2357G>A | c.543G>A | CAG ⇒ CAA | p.Q181Q | Q[Gln] ⇒ Q[Gln] | rs72554028 | 172660003 | 172660005 | - | - | + | + | + | + |
GATA4 | 8 | Exon 6 | g.80016C>T | c.419C>T | GCT ⇒ GTT | p.A346V | A[Ala] ⇒ V [Val] | rs115372595/ CM076205 | 11614482 | 11614484 | - | - | + | - | + | + |
8 | Exon 6 | g.80117G>A | c.1138G> A | GTG ⇒ ATG | p.V380M | V [Val] ⇒ M[Met] | rs114868912 | 11614583 | 11614585 | - | - | + | - | - | + |
8 | Intron 5-6 | g.79862C>T | - | - | - | - | rs3729854 | 11614328 | 11614330 | - | - | - | + | + | + |
TBX5 | 12 | Exon3 | g.6590C>A | c.215C>A | ACG ⇒ AAG | p.T72K | T [Thr] ⇒ K [Lys] | rs863223779 | 114839657 | 114839659 | + | - | - | + | + | - |
12 | Exon 8 | g.42133A>G | c.837A>G | CGA ⇒ CGG | p.R279R | R [Arg] ⇒ R[Arg] | rs564499369 | 11436639 | 114366311 | - | - | + | - | + | + |
Footnotes: ‘+’ indicates presence; ‘-’ indicates absence.
Detailed representation of GATA4 and TBX5 missense variants. Hydrogen bonds, weak hydrogen bonds, and steric clashes are shown in green dotted lines, gray dotted lines and pink dotted lines respectively. a) A346 mutation to V (A346V). b) V380 mutation to M (V380M). c) T72 mutation to K (T72K).
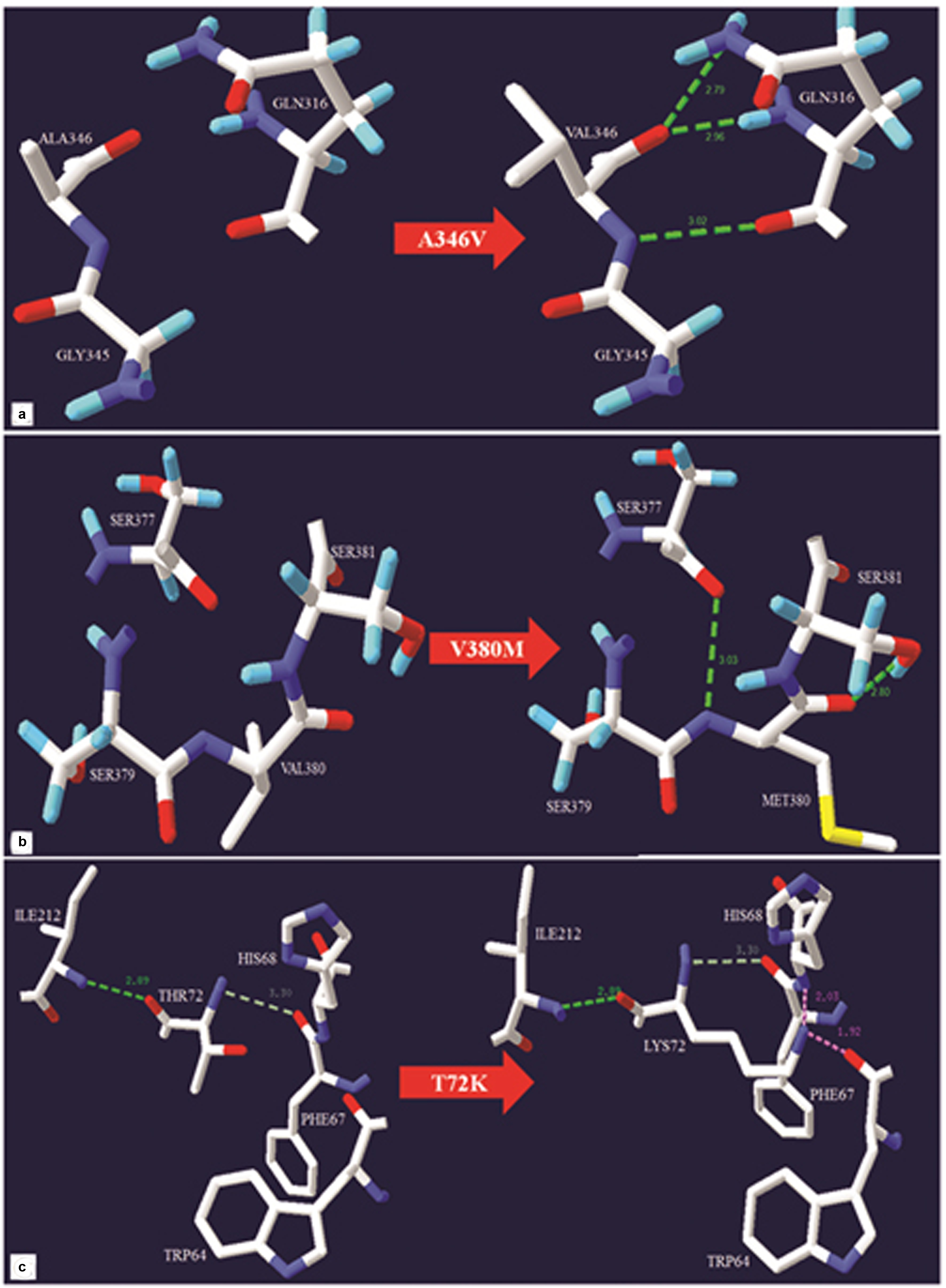
Discussion
We identified previously known genetic variants of isolated heart septal defects in NKX2-5 (c.63A>G, c.543G>A); GATA4 (c.419C>T, c.1138G>A and g.79862C>T) and TBX5 (c.215C>A, c.837A>G) in CGD patients using Sanger sequencing utilizing genomic DNA of CGD patients isolated via the standard phenol chloroform method. Period in between PCR targeted primers were designed for whole exons and partial flanking introns of GATA4, NKX2-5 and TBX5 using Primer 3 software and amplified [Table/Fig-5]. The PCR amplicons were then sequenced by the Big Dye Terminator ABI Prism sequencing kit (Applied Biosystems, Foster City, CA, USA), and run on ABI 3130 XL sequencer. DNA Sequencing was performed twice to confirm the variants using both forward and reverse primers. The acquired raw sequences were compared using NCBI BLAST and CLUSTAL W. The variations were then characterized for functional analysis using insilico tools such as SIFT, PANTHER, PolyPhen-2, Provean, I-Mutant to identify the sequence variation and its impact on the protein structure and function. Structural analysis and protein visualization for the missense variants was performed using Swiss-PDB viewer [16]. Cross species alignment of NKX2-5, GATA4, and TBX5 protein sequences showed that the affected amino acids lying in the conserved regions, suggesting that the amino acid is functionally important [11]. However, the mutated amino acid was not among the most commonly found residue types, indicating the mutation to be potentially affecting the protein conformation which may lead to the pathogenesis of Gerbode VSD in these patients. Functional characteristics of these variants through in silico analysis suggested that c.419C>T (p. A346V) and c.1138G>A (p. V380M) are benign (PolyPhen-2) and tolerated (SIFT). However, c.215C>A (p. T72K) is found to be deleterious (SIFT) and damaging (PolyPhen-2) [17,18].
The missense mutation of p.T72K has been previously reported in Saudi infant with Holt-Oram syndrome associated with congenital heart disease and found to be damaging to the TBX5 protein [19]. I-mutant analysis of these non synonymous variants showed protein destabilization. However, the reported synonymous variant c.63A>G of NKX2-5 in the present study alters the functional and conformational characteristics of the encoded protein [20]. Sequence variations in the NKX2-5 (c.63A>G, c.543G>A) and GATA4 (c.419C>T) genes have been reported to be associated with heart septal defects such as VSD and having similar implications has been observed in CGD patients in the present study [21,22]. However, the implications of missense variant c.1138G>A and the synonymous variant of TBX5, c.837A>G have been reported earlier in sporadic septal defects and non syndromic atrioventricular septal defect, respectively [23,24].
C-domain of GATA4 protein plays a major role in regulating the transcriptional activity. However, two non-synonymous variants (c.419C>T and c.1138G>A) in our case series are in the NLS and C-domain of GATA4 which may influence the transcriptional activity by altering the molecular mechanism of GATA4 [1,24]. The non-synonymous SNPs are documented to cause amino acid alterations resulting into the loss of protein structure and functions, causing altered phenotype [25]. But, in our study, the tertiary structure of the protein changes due to the alteration in the side chains of A346 and V380 of GATA4 and T72 of TBX5. The distribution of the polar and nonpolar amino acids directs the protein tertiary structure. These changes may not directly inactivate the protein but rather bring about subtle changes in the protein activity due to their interaction with the neighbouring proteins. In this study, the side chains variation and change in the 3D structure of amino acids [Table/Fig-4] suggests their potential role in non functionality of GATA4 and TBX5 proteins [25]. Our analysis suggests that the genetic variants identified in these cardiac genes may contribute to the pathogenesis of CGD by altering the gene and the protein expression.
PCR targeted primer sequences with product size for NKX2-5, GATA4 and TBX5.
Genes | Exons | Forward | Reverse | PCR product (bp) |
---|
NKX2-5 | Exon1a | 5’GCCTCTCCTGCCCCTTGTGCT3’ | 5’GGACGAAAGCGACCCAGGAG3’ | 550a |
Exon2a | 5’CCCTTACCATTACTGTGCGG3’ | 5’AGTCAGGGAGCTGTTGAGGTG3’ | 779 a |
Exon1b | 5’CCTTGTGCTCAGCGCTACCTG3’ | 5’ CGACAACACCAGGCATCTTACATT3’ | 635 |
Exon2b | 5’CTCCACGAGGATCCCTTACCATTA3’ | 5’ GAGAGTCAGGGAGCTGTTGAGGTG3’ | 794 |
GATA4 | Exon2a | 5’ GATCTTCGCGACAGTTCCTC3’ | 5’GTCCCCGGGAAGGAGAAG3’ | 458b |
Exon2b | 5’ GCTGGGCCTGTCCTACCT 3’ | 5’ AAAAACAAGAGGCCCTCGAC 3’ | 554b |
Exon3 | 5’GGGCTGAAGTCAGAGTGAGG3’ | 5’ GATGCACACCCTCAAGTTCC3’ | 437 b |
Exon4 | 5’GAGATCTCATGCAGGGTCGT3’ | 5’ GCCCCTTCCAAATCTAAGTC3’ | 390b |
Exon5 | 5’TCTTTCTCGCTGAGTTCCAG 3’ | 5’ GGGATGTCCGATGCTGTC3’ | 379b |
Exon6 | 5’GCCATCCCTGTGAGAACTGT 3’ | 5’GAGGGTAGCTCACTGCTTGC 3’ | 444b |
Exon7 | 5’AAGTGCTCCTTGGTCCCTTC3’ | 5’TTCCCCTAACCAGATTGTCG3’ | 479b |
TBX5 | Exon1a | 5’GGTATTCATTTGCCCAGAGC3’ | 5’CCCAGTAAAATAAAGAGGCAACC3’ | 478c |
Exon1b | 5’CCAGCCAAACGTGACAGC3’ | 5’GCCAAGTGCAAAGAGAAACC3’ | 390c |
Exon2 | 5’TTTCTCTCGTTCTCTCTCTGTCC3’ | 5’CAGACTCTGACTTTGATCTCTGC3’ | 297c |
Exon3 | 5’GTGTTTTGGGGGAGTTTGG3’ | 5’GCCACCTTTTCTTCTTCACC3’ | 243c |
Exon4 | 5’GAGGCTGCCTTAAAATACTGG3’ | 5’AACTTTTTGGGAGAAGGTTCC3’ | 248c |
Exon5 | 5’CTGGTGCGTGAACTGAAGC3’ | 5’GAGGACAAGAGGGAGACAAGG3’ | 282c |
Exon6 | 5’GGGAGCAGGGTTTTATCTGG3’ | 5’TGCAAAAGAAAGAGCAGACG3’ | 280c |
Exon7 | 5’TGGCTTAATTTGCTTCTTTTGG3’ | 5’GGTTGCTGCTGGCTTACC3’ | 294c |
Exon8 | 5’TCTCTCACACCTGGTTCAGC3’ | 5’ATACTCCTCACCCCCTCACC3’ | 390c |
Exon9a | 5’TTGGCCAAATAACTGTCTCC3’ | 5’GCTGGAACATTCCCTCTCC3’ | 465c |
Exon9b | 5’ACTTCTCCGCTCACTTCACC3’ | 5’TTTTTAAAATTGTGGTTTCAAGC3’ | 474c |
a Pang et al., 2012; b Yang et al., 2012; c Granados-Riveron et al., 2012.
Conclusion
We hereby report three cases with rare congenital GD with abnormalities such as tricuspid regurgitation, intracardiac shunt, and pulmonary artery hypertension. Molecular analysis may help to enrich the understanding of pathogenesis involved in the GD. To the best of our knowledge, this study is the first to investigate and detect gene sequence variants in CGD cases. Further investigations of genetic variations in confirmed CGD will strengthen these findings and enrich our understanding of cardiac specific transcription factors and their role in organogenesis.
Footnotes: ‘+’ indicates presence; ‘-’ indicates absence.
a Pang et al., 2012;
b Yang et al., 2012;
c Granados-Riveron et al., 2012.
[1]. Wang J, Fang M, Liu XY, Xin YF, Liu ZM, Chen XZ, A novel GATA4 mutation responsible for congenital ventricular septal defects Int J Mol Med 2011 28(4):557-64. [Google Scholar]
[2]. Yuan SM, Acquired left ventricle-to-right atrium shunt: Clinical implications and diagnostic dilemmas Wien Klin Wochenschr 2015 127(21-22):884-92. [Google Scholar]
[3]. Yuan SM, Left ventricular to right atrial shunt (Gerbode defect): Congenital versus acquired Postepy Kardiol Interwencyjnej 2014 10(3):185-94. [Google Scholar]
[4]. Demirkol S, GurkanYesil F, Bozlar U, Balta S, Sahin MA, Guler A, Multimodality imaging of a congenital Gerbode defect Kardiol Pol 2013 71(1):104 [Google Scholar]
[5]. Panduranga P, Mukhaini M, A rare type of Gerbode defect Echocardiography 2011 28(6):E118-120. [Google Scholar]
[6]. Prifti E, Ademaj F, Baboci A, Demiraj A, Acquired Gerbode defect following endocarditis of the tricuspid valve: A case report and literature review J Cardiothorac Surg 2015 10:115 [Google Scholar]
[7]. Gosciniak P, Larysz B, Baraniak J, Jozwa R, Zych A, Mokrzycki K, The Gerbode defect- A frequent echocardiographic pitfall Kardiologia Polska 2012 70(11):1191-93. [Google Scholar]
[8]. McCulley DJ, Black BL, Transcription factor pathway and congenital heart disease Curr Top Dev Biol 2012 100:253-77. [Google Scholar]
[9]. Granados-Riveron JT, Pope M, Bu’Lock FA, Thornborough C, Eason J, Setchfield K, Combined mutation screening of NKX2-5, GATA4, and TBX5 in congenital heart disease: Multiple heterozygosity and novel mutations Congenit Heart Dis 2012 7(2):151-59. [Google Scholar]
[10]. Blue GM, Kirk EP, Sholler GF, Harvey RP, Sinlaw DS, Congenital heart disease: Current knowledge about causes and inheritance Med J Aust 2012 197(3):155-59. [Google Scholar]
[11]. Winston JB, Schulkey CE, Chen IBD, Regmi SD, Efimova M, Erlich JM, Complex Trait Analysis of Ventricular Septal Defects Caused by Nkx2-5 Mutation Circ Cardiovasc Genet 2012 5(3):293-300. [Google Scholar]
[12]. An Y, Duan W, Huang G, Chen X, Li L, Nie C, Genome-Wide copy number variant analysis for congenital ventricular septal defects in Chinese Han population BMC Med Genomics 2016 9:2 [Google Scholar]
[13]. Pang S, Shan J, Qiao Y, Ma L, Qin X, Wanyan H, Genetic and functional analysis of the nkx2–5 gene promoter in patients with ventricular septal defects Pediatr Cardiol 2012 33(8):1355-61. [Google Scholar]
[14]. Yang YQ, Wang J, Liu XY, Chen XZ, Zhang W, Wang XZ, Novel GATA4 mutations in patients with congenital ventricular septal defects Med Sci Monit 2012 18:CR344-50. [Google Scholar]
[15]. Sanger F, Coulson AR, A rapid method for determining sequences in DNA by primed synthesis with DNA polymerase J Mol Biol 1975 94(3):441-48. [Google Scholar]
[16]. Guex N, Peitsch MC, SWISS-MODEL and the Swiss-Pdb Viewer: An environment for comparative protein modelling Electrophoresis 1997 18(15):2714-23. [Google Scholar]
[17]. Adzhubei IA, Schmidt S, Peshkin L, Ramensky VE, Gerasimova A, Bork P, A method and server for predicting damaging missense mutations Nat Methods 2010 7(4):248-49. [Google Scholar]
[18]. Kumar P, Henikoff S, Ng PC, Predicting the effects of coding non-synonymous variants on protein function using the SIFT algorithm Nat Protoc 2009 4(7):1073-81. [Google Scholar]
[19]. Al-Qattan MM, Abou Al-Shaar H, A novel missense mutation in the TBX5 gene in a Saudi infant with Holt-Oram syndrome Saudi Med J 2015 36(8):980-82. [Google Scholar]
[20]. Sauna ZE, Kimchi-Sarfaty C, Understanding the contribution of synonymous mutations to human disease Nat Rev Genet 2011 12(10):683-91. [Google Scholar]
[21]. Yashvanthi B, Ranjan KS, Krishnananda K, Rajasekhar M, Molecular genetic study on heart septal defects Journal of Global Biosciences 2016 5(1):3475-82. [Google Scholar]
[22]. Wang Z, Zou L, Zhong R, Zhu B, Chen W, Shen N, Associations between two genetic variants in NKX2-5 and risk of congenital heart disease in Chinese population: A meta-analysis PLoS One 2013 8(8):e70979 [Google Scholar]
[23]. Schluterman MK, Krysiak AE, Kathiriya IS, Abate N, Chandalia M, Srivastava D, Screening and biochemical analysis of GATA4 sequence variations identified in patients with congenital heart disease Am J Med Genet A 2007 143A(8):817-23. [Google Scholar]
[24]. Reamon-Buettner SM, Borlak J, TBX5 mutations in non-Holt-Oram syndrome (HOS) malformed hearts Hum Mutat 2004 24(1):104 [Google Scholar]
[25]. Manjegowda DS, Karunakar P, Ramachandra NB, Effect of structural changes in proteins derived from GATA4 nonsynonymous single nucleotide polymorphisms in congenital heart diseases Indian J Pharm Sci 2015 77(6):735-41. [Google Scholar]