Introduction
It has been demonstrated earlier that exposure to ethanol and/or arsenic compounds (such as sodium arsenite) produces toxic effects as shown by both in vitro and in vivo experiments. Chronic exposure of humans to arsenic through drinking water, pesticides or consumption of alcoholic beverages has produced major health problem and concern in recent years. Water being one of the main ingredients for alcohol formation (beer fermentation process) can lead to contamination with arsenic. Thus, people consuming such alcohol are getting continuously exposed to arsenic compounds as well along with alcohol.
Aim
The present study was undertaken to investigate the effect of alcohol co-administration on arsenic induced changes in carbohydrate metabolic status in adult male albino rats.
Materials and Methods
Adult male albino rats of Wistar strain (weighing~100g) were divided into three groups (n=8 rats/group) including Control or vehicle treated (C), Arsenic treated (As) and Arsenic treated alcohol co-exposed (As+Alc). Treatment with Sodium-arsenite included intra-peritoneal injection condivutively for 14 days at a dose of 5.55 mg/kg (equivalent to 35% of LD50) per day. Absolute alcohol (15% v/v) was fed at a dose of 0.5 ml/100 g body weight per day for five condivutive days from start of the treatment schedule. Distilled water (D/W) was used as vehicle. Blood Glucose (BG) level, levels of glycogen, Pyruvic Acid (PA), Free Amino Acid Nitrogen (FAAN), total protein, Glutamate Oxalate transaminase (GOT) and Glutamate Pyruvate Transaminase (GPT) activity, and glucose-6-phosphatase (G6Pase) activity were measured in tissues including liver, kidney and muscle.
Results
Treatment with arsenic decreased the levels of BG, liver glycogen and PA, tissue protein and G6Pase activity, GOT activity in liver and muscle, and increased free amino acid content in kidney and muscle, GPT activity in liver and kidney. Alcohol administration to rats co-exposed to arsenic treatment reversed these changes.
Conclusion
Thus, it is suggested that combined administration of alcohol with arsenic can result in the suppression of the down-regulating action of arsenic on glucose homeostasis as evidenced by its hypoglycaemic effect and increased gluconeogenesis and transamination in liver.
Introduction
It is a well known fact that water is one of the major constituent of both traditional and modern beer fermentation process which may or may not be contaminated with arsenic as a result of poor quality management. Subsequently, this can cause people to be exposed to alcohol and arsenic together. Experimentally, it has been shown earlier that the chemical interaction between sodium arsenite (an arsenic compound) and ethanol is responsible for the suppression of sodium arsenite – induced DNA disruption, reduced anti-acetylcholine esterase activity in the brain, liver damage, and anemic effects [1].
In the modern time though the use of arsenic as poison or as fertilizer has been reduced, yet arsenic contamination remains an environmental problem in some areas of the world. As a common environmental pollutant, arsenic is found to produce toxic effect to many mammalian cells. Environmental sources of arsenic arise from the continued use of its compounds as pesticides, from its unintended release during mining (in the form of ores such as realgar- As4S4, orpiment-As2S3, arsenolite-As2O3, etc.,) and as contaminant from the combustion of coal. Drinking water, mostly ground water, is a major source of arsenic [2,3]. However, trace amount of this element apparently is essential to good human health and probably act as growth stimulants [4–6].
The physiological effects of arsenic in vertebrates are complicated as it is affected by several variables such as speciation of the element, the exposure route, and the susceptibility of the particular animal species. Arsenic causes severe, potentially lethal, health effects, including cancer, in people [7–13]. Arsenic related illnesses are referred to as arsenicosis. It is thought that the greater toxicity of As (III) is due to its ability to be retained in the body longer, since it becomes bound to sulfhydryl groups [14,15]. Exposure to arsenic present in the environment can cause oxidative burst in the individuals leading to tissue damage [16,17]. It has been shown earlier that depletion of glucose is an important factor in arsenic toxicity [18]. This is also supported by the fact that arsenic intoxication alters the activities of enzymes involved in cellular glucose uptake, gluconeogenesis, fatty acid oxidation and production of glutathione [19].
Alcohol abuse effects are a major medical and social problem; rather it is the growing problem for the modern day society. Alcohol alters the structure and function of all organ systems in the body, from the brain to the bone, from the heart to the liver, from the reproductive organs to the immune system [20–25]. Alcohol ingestion changes hormone levels and compromises the general well-being of the organism.
Alcohol is particularly toxic to the brain [20,26]. The pharmacology and toxicology of alcohol is different in individuals and depends mostly on the forensic toxicology method used to analyze the blood alcohol levels. Ethanol is primarily metabolized in the liver. In the liver, ethanol is broken down to acetaldehyde by alcohol dehydrogenase. Acetic acid is fed into Kreb’s cycle and is ultimately broken down into carbon dioxide and water. Several reports are available on the impact of alcohol on carbohydrate homeostasis [27]. Previously, it has been reported that alcohol inhibits lactate-stimulated gluconeogenesis in the in situ perfused liver and isolated hepatocytes when given acutely in a dose-dependent manner [28,29]. Alcohol induced alteration of hepatic glycolytic and gluconeogenic enzyme activities have also been reported [30].
Thus, it becomes clear from the above literature survey that arsenic exposure has definite role in carbohydrate metabolism and that alcohol intoxication also affects the carbohydrate homeostasis in living organisms. Not much research has been done on the effect of arsenic co-exposed with alcohol on the carbohydrate metabolic status of the living beings. It is, therefore, intended to investigate whether arsenic co-exposed with alcohol has any specific or modified effect on carbohydrate metabolism.
The present study primarily deals with the effect of alcohol on arsenic-induced alteration of glucose homeostasis and some related enzymes.
Materials and Methods
This is a comparative study which was performed between September and October, 2014. According to the treatment protocol, it is a chronic study carried on albino rats involving fourteen consecutive days of treatment. The details of the experimental protocol are described below.
Maintenance of Animals
Twenty four adult male albino rats of Wistar strain (weighing~100 g) were used throughout the experiment. The animals were maintained under standard laboratory conditions (22-28°C, 60-70% relative humidity, 12:12 hours light/dark cycle) with 18% protein diet (containing 71% carbohydrate, 18% protein, 2% mineral, 7% fat, 1% vitamin and 1% choline chloride) and water ad libitum. Daily food intake (g/day/rat) and body weights of the rats were recorded and maintained throughout the experimental period. The experiments were carried out as per the regulation of the Institutional Animal Ethical Committee. The experiments were carried out in the Biochemistry Laboratory, Department of Physiology, University of Calcutta, Kolkata, India, with prior permission from the Head of the Department. The rats were maintained with adequate (18%) protein diet and sufficient drinking water for three days to get adapted to cage food and laboratory conditions. The dietary protocol was maintained throughout the treatment schedule.
Grouping and Treatment
On the day before treatment, adult male albino rats of Wistar strain (weighing~100 g, n=8 rats/group) were divided into three groups including Control (C), Arsenic treated (As) and Arsenic treated alcohol co-exposed (As+Alc) of equal average body weight. The animals of As and As+Alc group were injected intra-peritoneally with arsenic in the form of sodium arsenite for a period of 14 days consecutively at a dose of 5.55 mg/kg (equivalent to 35% of LD50) per day. Rats from As+Alc group were also fed with 15% (v/v) absolute alcohol at a dose of 0.5 ml/100 g body weight per day for five consecutive days of the treatment schedule. The rats of C group were injected distilled water (vehicle; D/W) intraperitoneally (0.1 ml/100 g) for 14 days consecutively and were also fed with D/W (0.5 ml/100 g body weight) for five consecutive days from beginning of treatment. Control group and As+Alc group were pair-fed with their respective As group. Everyday a measured and equal amount of food was given to all the groups. It was observed that animals undergoing treatment with arsenic were rejecting food (about 15 g). Therefore, to avoid the effect of reduced diet on glucose homeostasis, the other groups were pair-fed with the reduced amount of food. The doses of arsenic and alcohol were based on previous studies from the laboratory [19]. Body weight and food intake of all animals were recorded regularly.
Tissue Collection
After the treatment period, the rats were weighed to get the post-treatment body weight. The overnight fasted rats were sacrificed by cervical dislocation. Heparinized vials were used to collect blood samples and kept in frozen condition until analysis. The organs (liver and kidney) and leg muscle were collected, washed with ice-cold saline, blotted dry and immediately kept at -20°C until analyzed.
Homogenate Preparation
Known amounts of liver, kidney and muscle tissues were homogenized with all-glass homogenizer (approximately at 2000 rpm for two minutes) in 0.1 M phosphate buffer (pH 7.4) and 0.1M Tris-HCl buffer (pH 6.5) separately. A 10% homogenate was prepared to estimate the following parameters:
Biochemical Estimation
Estimation of blood-glucose: Protein free blood filtrate was used to determine blood glucose level by the method of Nelson-Somogyi [31].
Estimation of liver and muscle glycogen: Liver and muscle glycogen contents were estimated according to the method as suggested by Montgomery [32]. An alcoholic extract of glycogen was used from the tissues and the glycogen level was determined in terms of glucose.
Estimation of liver pyruvic acid: Liver pyruvic acid content was measured spectrophotometrically using a 10% liver homogenate in 0.1 M chilled phosphate buffer (pH 7.4) [33].
Estimation of free amino acid nitrogen: Free amino acid nitrogen contents of liver, kidney and muscle tissues were estimated spectrophotometrically using a 10% homogenate of the tissues in 0.1 M phosphate buffer (pH 7.4) [34]. Briefly, the homogenate was diluted with 0.25 M sucrose solution, and to it 2/3 (N) H2SO4 and 10% sodium tungstate was added for protein precipitation. The protein free filtrate thus obtained was finally used for estimation of free amino acid nitrogen content and expressed in terms of microgram of leucine amino acid per 100 mg tissue.
Estimation of tissue protein: Tissue protein contents of liver, kidney and muscle were estimated spectrophotometrically [35]. Bovine serum albumin was used as standard in this method.
Estimation of glutamate-oxalate transaminase (GOT) and glutamate-pyruvate transaminase (GPT) activity: A 10% tissue homogenate of liver, kidney and muscle respectively was used to estimate GOT and GPT activity spectrophotometrically [36]. Briefly, a mixture of 0.1 ml of 10% tissue homogenate and 0.16 M GOT or GPT substrate called incubation mixture was incubated at 370C for 30 minutes and the enzyme activity was expressed as micromoles of pyruvate produced per minute per 100 mg tissue.
Estimation of glucose-6-phosphatase activity in liver: A 10% homogenate of liver tissue was used to estimate Glucose-6-Phosphatase activity spectrophotometrically [37]. It was expressed in terms of microgram of phosphate liberated per minute by 100 mg tissue.
Statistical Analysis
Data so obtained from each experiment were subjected to statistical analysis using SPSS 16.0 for Windows (SPSS Inc., Chicago, USA). The significance level of the observed values from C, As and As+Alc groups of rats was computed according to two-tailed Student t-test and the probability of chance of occurrence (p) was determined accordingly.
Results
Effect of arsenic co-exposed with alcohol on body weight and organ weight: In the present investigation arsenic treatment had no significant effect on the body weight as well as liver and kidney weight in terms of both absolute and per 100 g body weight of rats with or without alcohol administration (p>0.10) [Table/Fig-1].
Effect of arsenic co-exposed with alcohol on body weight and organ weight. (The values are mean±SEM; n=8/group; pa= compared with control group; pb= compared with As group).
GroupsofAnimals | Bodyweight(g) | Organ weight |
---|
Liver | Kidney |
---|
(g) | (g/100gbodyweight) | (g) | (g/100gbodyweight) |
---|
Controlgroup | 116.67±3.78 | 3.45±0.15 | 2.96±0.09 | 0.81±0.06 | 0.69±0.05 |
Asgroup | 117.33±4.37pa>0.1 | 3.54±0.06pa>0.1 | 3.03±0.11pa>0.1 | 0.79±0.04pa>0.1 | 0.68±0.06pa>0.1 |
As+Alcgroup | 116.33±4.77pb>0.1 | 3.56±0.17pb>0.1 | 3.10±0.08pb>0.1 | 0.74±0.04pb>0.1 | 0.65±0.03pb>0.1 |
Effect of arsenic co-exposed with alcohol on blood glucose level: The results revealed that arsenic treatment decreased the blood glucose level significantly (p<0.02) which was restored after alcohol administration (p<0.001) [Table/Fig-2].
Effect of arsenic co-exposed with alcohol on blood glucose level and tissue glycogen content. (The values are mean±SEM; n=8/group; pa= compared with control group; pb= compared with As group).
Groupsof Animals | Blood glucose(mg/100 ml) | Glycogen (mg/100g tissue) |
---|
Liver | Muscle |
---|
Control group | 105.55±7.95 | 41.6±5.58 | 24.80±1.40 |
As group | 76.92±3.32pa<0.02 | 22.2±1.23pa<0.02 | 24.27±3.71pa>0.1 |
As+Alc group | 150.00±5.69pb<0.001 | 127.4±6.42pb<0.001 | 22.36±0.88pb>0.1 |
Effect of arsenic co-exposed with alcohol on glycogen content: The glycogen content (in terms of total, per 100 g tissue and per 100g body weight) was reduced significantly following arsenic treatment and alcohol administration appeared to have significant role in the restoration of liver glycogen level. The results also revealed that arsenic treatment do not have significant effect on muscle glycogen level of rats with or without alcohol administration [Table/Fig-2].
Effect of arsenic co-exposed with alcohol on liver pyruvic acid content: The results revealed that arsenic treatment decreased the liver pyruvate level significantly which was restored after alcohol administration [Table/Fig-3].
Effect of arsenic co-exposed with alcohol on liver pyruvate level. (The values are mean±SEM; n=8/group; pa= compared with control group; pb= compared with As group).
Groupsof Animals | Liver pyruvate content |
---|
Total (mg) | (mg/g tissue) | (mg/100g body wt) |
---|
Control group | 82.13±5.16 | 23.88±2.15 | 72.44±5.64 |
As group | 35.49±2.19pa<0.001 | 9.78±1.24pa<0.01 | 29.62±3.35pa<0.01 |
As+Alc group | 70.22±4.32pb<0.001 | 23.47±2.14pb<0.01 | 68.22±5.47pb<0.01 |
Effect of arsenic co-exposed with alcohol on free amino acid nitrogen content: Arsenic treatment increased the free amino acid nitrogen content of liver (p<0.001) by 141% and decreased that of kidney (p<0.01) by 63% and muscle (p<0.001) by 51% in terms of mg per g tissue. The levels in kidney and liver were completely restored to control level by alcohol administration. However, alcohol administration increased (p<0.001) the muscle free amino acid nitrogen level to double the control level [Table/Fig-4]. The results also revealed that arsenic treatment decreased the free amino acid nitrogen level in liver as well as kidney both in terms of total (mg) and per 100 g body weight. The levels were however restored to control level by alcohol administration [Table/Fig-5].
Effect of arsenic co-exposed with alcohol on free amino acid nitrogen. (The values are mean±SEM; n=8/group; pa= compared with control group; pb= compared with As group).
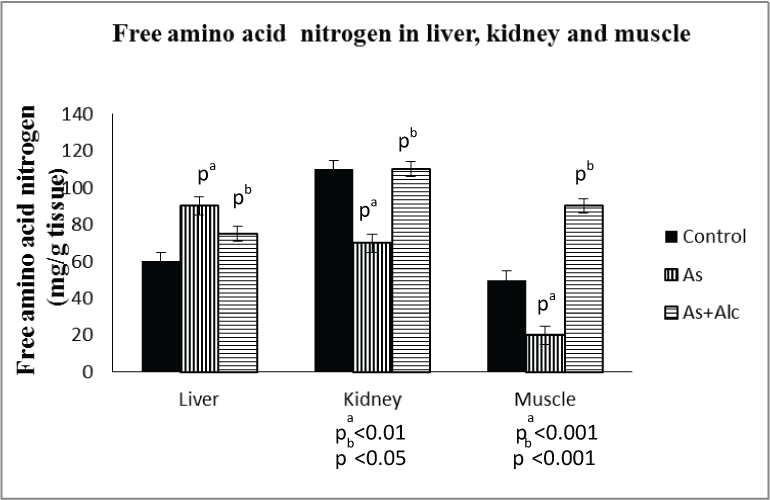
Effect of arsenic co-exposed with alcohol on free amino acid nitrogen. (The values are mean±SEM; n=8/group; pa= compared with control group; pb= compared with As group).
Groupsof animals | Free amino acid nitrogen |
---|
Liver | Kidney |
---|
Total (mg) | (mg/100gbody wt) | Total (mg) | (mg/100gbody wt) |
---|
Control group | 208.17±12.48 | 191.56±7.99 | 87.62±4.01 | 75.35±8.31 |
As group | 112.54±6.25pa<0.001 | 98.08±3.01pa<0.001 | 53.54±3.33pa<0.001 | 38.47±3.52pa<0.001 |
As+Alcgroup | 241.22±18.89pb<0.01 | 214.87±12.06pb<0.001 | 77.70±6.15pb<0.05 | 69.52±6.59pb<0.01 |
Effect of arsenic co-exposed with alcohol on tissue protein content: Arsenic treatment decreased the protein content in liver (p<0.001) by 54% and kidney (p<0.001) by 50%, which were completely restored to control level after alcohol administration. However, arsenic treatment increased the protein content in muscle significantly (p<0.05) which was not restored to control level after alcohol administration [Table/Fig-6].
Effect of arsenic co-exposed with alcohol on tissue protein content. (The values are mean±SEM; n=8/group; pa= compared with control group; pb= compared with As group).
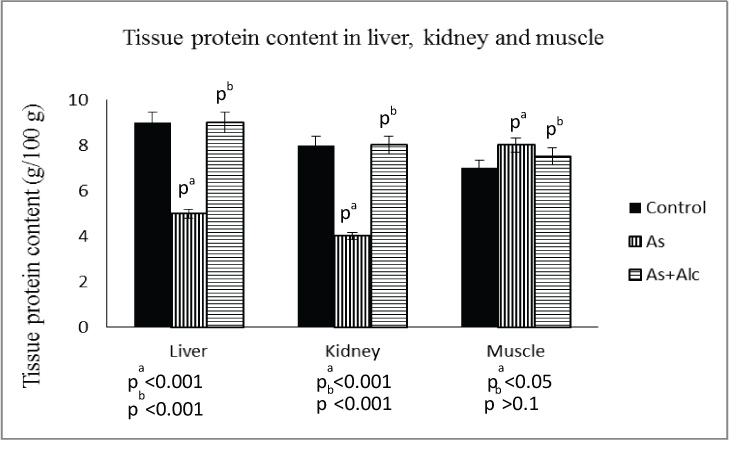
Effect of arsenic co-exposed with alcohol on GPT activity: Arsenic treatment with or without alcohol administration had no effect on GPT activity in liver, kidney and muscle in terms of per 100 mg tissue. But, in terms of per 100 mg tissue protein, arsenic treatment increased the GPT activity in both liver (p<0.01) and kidney (p<0.01), which was restored to control level on alcohol administration. However, there was no significant change in GPT activity in muscle in terms of 100 mg tissue protein after arsenic treatment both with and without alcohol administration [Table/Fig-7].
Effect of arsenic co-exposed with alcohol on glutamate pyruvate transaminase activity. (The values are mean±SEM; n=8/group; pa= compared with control group; pb= compared with As group).
Groups of Animals | Liver | Kidney | Muscle |
---|
μg pyruvate/min/100 mg tissue | μg pyruvate/min/100 mg tissue protein | μg pyruvate/min/100 mg tissue | μg pyruvate/min/100 mg tissue protein | μg pyruvate/min/100 mg tissue | μg pyruvate/min/100 mg tissue protein |
---|
Control group | 28.91±1.34 | 0.33±0.02 | 12.45±0.70 | 0.16±0.01 | 18.71±1.71 | 0.27±0.03 |
As group | 28.74±0.61pa>0.1 | 0.64±0.07pa<0.01 | 11.45±0.77pa<0.1 | 0.29±0.03pa<0.01 | 19.63±1.22pa>0.1 | 0.24±0.04pa>0.1 |
As+Alc group | 29.89±1.69pb>0.1 | 0.34±0.02pb<0.01 | 13.60±0.86pb>0.1 | 0.17±0.01pb<0.02 | 18.55±1.53pb>0.1 | 0.24±0.02pb>0.01 |
Effect of arsenic co-exposed with alcohol on GOT activity: Arsenic treatment decreased the GOT activity in liver (p<0.001) and muscle (p<0.001) in terms of per 100 mg tissue, which were restored to control level after alcohol administration. There was no significant change in GOT activity in kidney in terms of per 100 mg tissue after arsenic treatment both with and without alcohol administration. However, arsenic treatment increased the GOT activity in kidney (p<0.01) and decreased in muscle (p<0.01) in terms of per 100 mg tissue protein, both of which were restored to control level on alcohol administration. Arsenic treatment had no effect on GOT activity in liver in terms of per 100 mg tissue protein both with and without alcohol administration [Table/Fig-8].
Effect of arsenic co-exposed with alcohol on glutamate oxalate transaminase activity. (The values are mean±SEM; n=8/group; pa= compared with control group; pb= compared with As group).
Groups of Animals | Liver | Kidney | Muscle |
---|
μg pyruvate/min/100 mg tissue | μg pyruvate/min/100 mg tissue protein | μg pyruvate/min/100 mg tissue | μg pyruvate/min/100 mg tissue protein | μg pyruvate/min/100 mg tissue | μg pyruvate/min/100 mg tissue protein |
---|
Control group | 15.4±0.58 | 0.18±0.01 | 5.88±0.51 | 0.07±0.006 | 8.03±0.48 | 0.12±0.010 |
As group | 9.8±0.84pa<0.001 | 0.22±0.03pa>0.1 | 5.72±0.47pa>0.1 | 0.14±0.013pa<0.01 | 4.87±0.48pa<0.001 | 0.06±0.006pa<0.01 |
As+Alc group | 15.93±1.07pb<0.001 | 0.18±0.01pb>0.1 | 4.74±0.47pb>0.1 | 0.06±0.008pb<0.01 | 8.6±0.63pb<0.01 | 0.11±0.015pb<0.05 |
Effect of arsenic co-exposed with alcohol on glucose-6-phosphatase activity: Arsenic treatment decreased (p<0.01) the liver glucose-6-phosphatase activity, which was restored completely to control level following alcohol administration (p<0.001) both in terms of per 100 mg tissue and per 100 mg tissue protein [Table/Fig-9].
Effect of arsenic co-exposed with alcohol on glucose-6-phosphatase activity in liver. (The values are mean±SEM; n=8/group; pa=compared with control group; pb=compared with As group).
Groups of animals | mμg/100 mg liver tissue | mμg/100 mg tissue protein |
---|
Control group | 34.43±2.018 | 532.92±59.11 |
As group | 23.83±0.445pa<0.01 | 392.60±22.13pa<0.01 |
As+Alc group | 54.12±2.486pb<0.001 | 620.09±48.02pb<0.001 |
Discussion
In the present investigation, we have evaluated the effect of alcohol co-exposure on arsenic –induced changes in carbohydrate status in adult albino rats [Table/Fig-10]. We have observed that arsenic treatment both without and with alcohol administration had no significant effect on the body weight as well as the liver and kidney weights. These findings suggest that the changes in the organ weight in relation to body weight are independent of arsenic treatment without or with alcohol administration at the present dose and duration [38]. This is in contrast to an earlier finding which showed that arsenic treatment reduced the body weight in a concentration dependent manner and that significantly increased with alcohol and arsenic treatment together [1], however, similar to the results in this study there was no effect on organ weight.
Representative picture showing the beneficial effect of alcohol administration on carbohydrate metabolism in rats altered by arsenic toxicity.
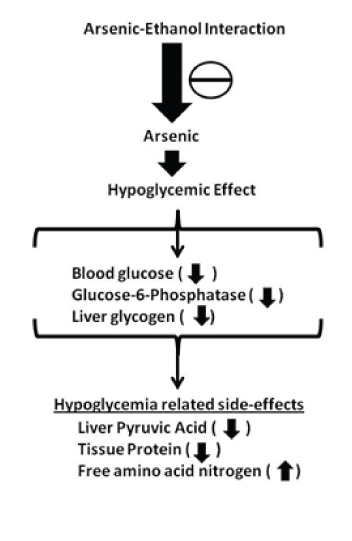
Arsenic treatment at the present dose and duration produced hypoglycaemic effect as evident by marked decrease in blood glucose level. This is in coherence with another study which showed that administration of arsenic in mice decreases glucose and glycogen contents in liver significantly [39]. In the present investigation, the decreased blood glucose level following arsenic treatment was also found to be accompanied with diminished liver glycogen content. It has been reported previously that glucose supplementation improves survival and symptoms in mice poisoned with arsenic and thus supporting the assumption that carbohydrate depletion is an important factor in arsenic toxicity [18]. The marked decrease in blood glucose level in arsenic treated rats, as observed in the present investigation, may also result from impairment of renal reabsorption of glucose leading to glycosuria. This contention is supported by the fact that most of the heavy metals including arsenic are found to be nephrotoxic and the usual site of damage is the renal tubule [40,41]. Another mechanism for reduced blood glucose level following arsenic exposure may be increased uptake of glucose by the cells. This appears likely as arsenic treatment like other cellular stress causes shifting of glucose transporter from intracellular site to the plasma membrane, and the degree of such translocation correlates approximately with the increase in glucose uptake. However, arsenic treatment in the present dose and duration has no significant effect on muscle glycogen store.
Arsenic is a trivalent element having higher affinity to bind with protein thiols, thereby suppressing Kreb’s cycle by inhibiting the actions of pyruvate and α-ketoglutarate dehydrogenase [42,43]. This reduces the production of energy rich phosphates and also causes depletion of liver glycogen and hypoglycaemia [44]. Current study revealed that liver pyruvic acid level decreased markedly following arsenic treatment and this may be due to immediate release of liver glucose produced by glycogenolysis to replenish the loss of glucose resulting from arsenic treatment causing diminutions in the accumulation of pyruvic acid in liver by retarding glycolytic reaction. The reduced glycolytic activity in liver may be due to hypoglycaemia induced by arsenic treatment. This is in coherence with earlier study showing that arsenic treatment impairs hepatic glycolysis as evident by reduced formation of glycolytic intermediates [45].
The enzymes GPT or GOT are presently known as Alanine Transaminase (ALT) [46]. These enzymes catalyze reversible transamination reactions to form pyruvate and glutamate by transferring an amino group from alanine (amino acid) to α-ketoglutarate (keto acid). Thus, ALT converts amino acid to a keto acid and requires pyridoxal phosphate for this. The evaluation of ALT (GOT or GPT) in liver is considered as a marker for hepatocellular injury [47,48]. In the present study, arsenic treatment significantly decreased the tissue protein content and increased the free amino acid nitrogen content of liver with significant rise in GPT activity and decreased GOT activity in liver, suggesting that there has been an overall small increase in the rate of transamination from amino acids to produce the intermediates of the gluconeogenic pathway. Arsenic induced decrease in tissue protein content and free amino acid nitrogen content and increase in transaminase (GPT and GOT) activity in kidney may result from the mobilization of amino acid nitrogen from kidney to liver to provide substrate for gluconeogenesis to release glucose to blood. In spite of increased free amino acid nitrogen and tissue protein content in muscle, GPT activity remains unaltered and GOT activity are diminished significantly following arsenic treatment. This diminished transaminase activity in muscle may be attributed to the inhibitory effects of the accumulated intermediate products of TCA cycle. It is also possible that arsenic induced hypoglycaemia causes increased secretion of adrenal corticoids, resulting in mobilization of free amino acids from peripheral tissues including kidney and muscle to liver [49].
The enzyme G6Pase is considered as a key enzyme in the homeostatic regulation of blood glucose levels as it regulates the first step in gluconeogenesis and glycogenolysis [50]. G6Pase hydrolyzes glucose-6-phospahte to free glucose with the release of a phosphate group. Arsenic treatment at the present dose and duration caused a marked decrease in glucose-6-phosphatase activity in the liver that may be due to decreased accumulation of glucose-6-phosphate and other glycolytic intermediates [39,45]. This arsenic induced effect on G6Pase may be due to alteration of its synthesis at transcriptional (DNA) or translational (mRNA) level [51].
The present study further reveals that alcohol administration appreciably counteracts the arsenic induced reduction of blood glucose level, liver glycogen and pyruvic acid level. In contrast, it has been shown that continued consumption of alcohol can be a risk factor for hypoglycaemia in individuals with Type 1-diabetes [52]. Thus, it appears that carbohydrate-depleting effect of arsenic may be prevented by alcohol administration. This is supported by the fact that there is increase in the protein expression of enzymes involved in liver gluconeogenesis (G6Pase) and glycogen synthesis in rats exposed to alcohol [53]. It has also been suggested that ethanol induces decrease in lactate/pyruvate excretion and decreases the glycolytic activity in liver [54]. However, acute alcohol administration under hypoxia dramatically accelerates the intracellular acidosis and the simultaneous ATP decrease [55].
In the present investigation, alcohol administration reversed the arsenic induced changes in free amino acid nitrogen concentration in liver, kidney and muscle. Alcohol administration also counteracts the arsenic induced decreased tissue protein content in liver and kidney and it seems to have no effect on muscles, suggesting reduced gluconeogenesis. This is in contrast to another study which reported that ethanol consumption directly inhibits gluconeogenesis [56]. Thus, this study supports the contention that a chemical interaction between alcohol and arsenic is producing a reversed effect. It has been shown earlier that alcohol causes hepatomegaly and hepatic protein accumulation due to ethanol-elicited deceleration of protein catabolism [57]. This suggests that free amino acids are mobilized from kidney to liver to provide substrate for increased gluconeogenesis due to arsenic treatment is counteracted by alcohol administration.
It has been shown earlier that exposure to sodium arsenite caused an increase in ALP activity in serum, thus clearly indicating an induction of hepatotoxicity and oxidative stress in the hepatocytes, and that the co-administration of alcohol reduced such activity of ALP in a concentration dependent manner [1,58]. In coherence, our study also shows that alcohol administration decreased the arsenic induced increased GPT activity in liver and kidney and decreased GOT activity in kidney. This may be ascribed to the restoration of free amino acid nitrogen concentration in the liver and kidney of arsenic treated animals by alcohol administration. The increased GOT activity in liver and muscle following alcohol administration in spite of increased free amino acid nitrogen content in arsenic treated animals may be attributed to the cellular toxicity caused by alcohol administration, as has been reported earlier that chronic alcohol abuse causes cellular toxicity [59], increases liver enzymes and causes pancreatitis (elevated serum lipase and amylase).
Alcohol administration has been found to be effective in the restoration of glucose-6-phosphatase in liver. Alcohol opposes the carbohydrate depleting effect of arsenic in liver and leads to enhanced accumulation of glycogen and pyruvic acid, thus contributing to the accumulation of glycolytic intermediates in liver and increasing the glucose-6-phosphatase activity to provide more glucose to the blood in order to replenish the lost blood glucose due to arsenic treatment.
Limitation
In this study we evaluated the effect of arsenic and alcohol together on carbohydrate status. However, further studies in this field are needed to identify the complete relationship of arsenic and alcohol with the gluconeogenic, glycolytic and other enzymes of carbohydrate metabolism, and the involvement of other probable factors, and their mechanism of action.
Conclusion
Thus it may be possible to sum up the total process by saying that an excess arsenic intake has significant impact on glucose homeostasis and that can be reverted back with alcohol administration. Accordingly, it is suggested that alcohol exerts protective effect against arsenic-induced alteration of glucose homeostasis and other related metabolic changes. Arsenic in spite of being toxic to the body has insulin like action leading to hypoglycaemia but further studies are required to ascertain whether hypoglycaemic effect of arsenic is still maintained after withdrawal of arsenic. Alcohol can be administered to recover from this hypoglycaemic condition. Alcohol triggers the glycogenolytic activity to increase the supply of glucose following increased demand by the body. It also affects gluconeogenetic as well as glycolytic pathways.
[1]. Aliyu M, Odunola O, Owumi S, Habila N, Aimola I, Erukainure O, Ethanol suppresses the effects of sodium arsenite in male wister albino rats Scientific reports 2012 1:1-6. [Google Scholar]
[2]. Pierce P, Argos M, Chen Y, Melkonian S, Parvez F, Islam T, Arsenic exposure, dietary patterns, and skin lesion risk in Bangladesh: A prospective study Am J Epidemiol 2011 173:345-54. [Google Scholar]
[3]. Smith A, Lingas E, Rahman M, Contamination of drinking water by arsenic in Bangladesh: A public health emergency Bull World Health Organ 2000 78:1093-103. [Google Scholar]
[4]. Ding W, Zhang L, Kim S, Tian W, Tong Y, Liu J, Arsenic sulfide as a potential anti cancer drug Molecular Medicine Reports 2015 11:968-74. [Google Scholar]
[5]. Nakagawa Y, Akao Y, Morikawa H, Hirata I, Katsu K, Naoe T, Arsenic trioxide-induced apoptosis through oxidative stress in cells of colon cancer cell lines Life Sci 2002 70:2253-69. [Google Scholar]
[6]. Cheremisinoff NP, Arsenic in Handbook of Pollution Prevention Practices 2001 CRC Press Edn:38-40. [Google Scholar]
[7]. Celik I, Gallicchio L, Boyd K, Lam TK, Matanoski G, Tao X, Arsenic in drinking water and lung cancer: A systematic review Environ Res 2008 108:48-55. [Google Scholar]
[8]. Mink PJ, Alexander DD, Barraj LM, Kelsh MA, Tsuji JS, Low-level arsenic exposure in drinking water and bladder cancer: A review and meta-analysis Regul Toxicol Pharmacol 2008 52:299-310. [Google Scholar]
[9]. Liu J, Waalkes MP, Liver is a target of arsenic carcinogenesis Toxicol Sci 2008 105:24-32. [Google Scholar]
[10]. Yu HS, Liao WT, Chai CY, Arsenic carcinogenesis in the skin J Biomed Sci 2006 13:657-66. [Google Scholar]
[11]. Chen CJ, Chen CW, Wu MM, Kuo TL, Cancer potential in liver, lung, bladder and kidney due to ingested inorganic arsenic in drinking water Br J Cancer 1992 66:888-92. [Google Scholar]
[12]. Yuan Y, Marshall G, Ferreccio C, Steinmaus C, Liaw J, Bates M, Kidney cancer mortality: Fifty-year latency patterns related to arsenic exposure Epidemiology 2010 21:103-08. [Google Scholar]
[13]. Brinkel J, Khan MH, Kraemer A, A systematic review of arsenic exposure and its social and mental health effects with special reference to Bangladesh Int J Environ Res Public Health 2009 6:1609-19. [Google Scholar]
[14]. Kotyzova D, Bludovska M, Eybl V, Differential influences of various arsenic compounds on antioxidant defense system in liver and kidney of rats Environ Toxicol Pharm 2013 36:1015-21. [Google Scholar]
[15]. Hughes MF, Arsenic toxicity and potential mechanisms of action Toxicol Lett 2002 133:1-16. [Google Scholar]
[16]. Das S, Upadhaya P, Giri S, Arsenic and smokeless tobacco induce genotoxicity, sperm abnormality as well as oxidative stress in mice invivo Genes and Environment 2016 38:1-8. [Google Scholar]
[17]. Flora SJS, Arsenic-induced oxidative stress and its reversibility Free Radic Biol Med 2011 51:257-81. [Google Scholar]
[18]. Reichl FX, Kreppel H, Sziniccz L, Forth W, Effect of glucose treatment on carbohydrate content in various organs in mice after acute As2O3 poisoning Vet Hum Toxicol 1991 33:230-35. [Google Scholar]
[19]. Pal S, Chatterjee AK, Protective effect of methionine supplementation on arsenic-induced alteration of glucose homeostasis Food and Chemical Toxicology 2004 42:737-42. [Google Scholar]
[20]. Hayes DM, Deeny MA, Shaner CA, Nixon K, Determining the threshold for alcohol-induced brain damage: New evidence with gliosis markers Alcohol Clin Exp Res 2013 37:425-34. [Google Scholar]
[21]. Garcia JA, Souza AL, Cruz LH, Marques PP, Camilli JA, Nakagaki WR, Effects of ethanol consumption and alcohol detoxification on the biomechanics and morphology the bone in rat femurs Braz J Biol 2015 75:983-88. [Google Scholar]
[22]. Ikehara S, Iso H, Toyoshima H, Date C, Yamamoto A, Kikuchi S, Japan collaborative cohort study group: Alcohol consumption and mortality from stroke and coronary heart disease among Japanese men and women: The Japan collaborative cohort study Stroke 2008 39:2936-42. [Google Scholar]
[23]. Gao B, Bataller R, Alcoholic liver disease: Pathogenesis and new therapeutic targets Gastroenterology 2011 141:1572-85. [Google Scholar]
[24]. La Vignera S, Condorelli RA, Balercia G, Vicari E, Calogero AE, Does alcohol have any effect on male reproductive function? A review of literature Asian Journal of Andrology 2013 15:221-25. [Google Scholar]
[25]. Sarkar D, Jung K, Wang J, Alcohol and the immune system Alcohol Res 2015 37:153-55. [Google Scholar]
[26]. Pla A, Pascual M, Renau-Piqueras J, Guerri C, TLR4 mediates the impairment of ubiquitin-proteasome and autophagy-lysosome pathways induced by ethanol treatment in brain Cell Death Dis 2014 5:e1066 [Google Scholar]
[27]. Steiner JL, Crowell KT, Lang CH, Impact of alcohol on glycaemic control and insulin action Biomolecules 2015 5:2223-46. [Google Scholar]
[28]. Krebs HA, The effects of ethanol on the metabolic activities of the liver Adv Enzyme Regul 1968 6:467-80. [Google Scholar]
[29]. Baranyai JM, Blum JJ, Quantitative analysis of intermediary metabolism in rat hepatocytes incubated in the presence and absence of ethanol with a substrate mixture including ketoleucine Biochem J 1989 258:121-40. [Google Scholar]
[30]. Duruibe V, Tejwani GA, The effect of ethanol on the activities of the key gluconeogenic and glycolytic enzymes of rat liver Mol Pharmacol 1981 20:621-30. [Google Scholar]
[31]. Oser BL, Hawk’s Physiological Chemistry 1976 14LondonMcGraw Hill Book Company:1054-1055. [Google Scholar]
[32]. Oser BL, Hawk’s Physiological Chemistry 1976 14LondonMcGraw Hill Book Company:1102-03. [Google Scholar]
[33]. Stroev EA, Makarova VG, Laboratory Manual in Biochemistry 1989 MoscowMir Publisher:147-48. [Google Scholar]
[34]. Rosen HA, A modified ninhydrin colorimetric analysis for amino acids Arch Biochem Biophys 1957 67:10-15. [Google Scholar]
[35]. Lowry OH, Rosenbrough NJ, Farr AL, Randall RJ, Protein measurement from phenol reagent J Biol Chem 1951 193:265-75. [Google Scholar]
[36]. Reiman S, Frankel S, Determination of SGPT and SGOT Am J Clin Pathol 1957 28:50-60. [Google Scholar]
[37]. Plummer DT, An Introduction to Practical Biochemistry 1988 New DelhiTata McGraw Hill:273 [Google Scholar]
[38]. Pal S, Chatterjee AK, Prospective protective role of melatonin against arsenic-induced metabolic toxicity in Wistar rats Toxicology 20005 208:25-33. [Google Scholar]
[39]. Reichl FX, Sziniccz L, Kreppel H, Fischtl B, Forth B, Forth W, Effect of glucose in mice after acute experimental poisoning with arsenic trioxide Arch Toxicol 1990 64:336-38. [Google Scholar]
[40]. Robles-Osorio ML, Sabath-Silva E, Sabath E, Arsenic-mediated nephrotoxicity Ren Fail 2015 37:542-47. [Google Scholar]
[41]. Peraza MA, Cromey DW, Carolus B, Carter DE, Gandolfi AJ, Morphological and functional alterations in human proximal tubular cell line induced by low level inorganic arsenic: Evidence for targeting of mitochondria and initiated apoptosis J Appl Toxicol 2006 26:356-67. [Google Scholar]
[42]. Winski SL, Carter DE, Interactions of rat red blood cell sulfhydryls with arsenate and arsenite J Toxicol Environ Health 1995 46:379-97. [Google Scholar]
[43]. Hu Y, Su L, Snow ET, Arsenic toxicity is enzyme specific and its effects on ligation are not caused by the direct inhibition of DNA repair enzymes Mutat Res 1998 408:203-18. [Google Scholar]
[44]. Lenartowicz E, A complex effect of arsenite on the formation of α-ketoglutarate in rat liver mitochondria Arch Biochem Biophys 1990 283:388-96. [Google Scholar]
[45]. Reichl FX, Szinicz L, Kreppel H, Forth W, Effect of arsenic on carbohydrate metabolism after single or repeated injection in guinea pigs Arch Toxicol 1988 62:473-75. [Google Scholar]
[46]. Karmen A, Wróblewski F, LaDue JS, Transaminase activity in human blood J Clin Invest 1955 34:126-33. [Google Scholar]
[47]. Wang CS, Chang TT, Yao WJ, Wang ST, Chou P, Impact of increasing alanine aminotransferase levels within normal range on incident diabetes J Formos Med Assoc 2012 111:201-08. [Google Scholar]
[48]. Ghouri N, Preiss D, Sattar N, Liver enzymes, nonalcoholic fatty liver disease, and incident cardiovascular disease: A narrative review and clinical perspective of prospective data Hepatology 2010 52:1156-61. [Google Scholar]
[49]. Schimmer BP, Parker KL, Adrenocorticotropic hormone; adrenocortical steroids and their synthetic analogues; inhibitors of the synthesis and actions of adrenocortical hormones. Cited in: Hardman JG, Limbird LE (Eds) Goodman and Gilman’s the pharmacological basis of therapeutics New York: LondonMcGraw-Hill:1649-1677. [Google Scholar]
[50]. Ghosh A, Shieh JJ, Pan CJ, Sun MS, Chou JY, The catalytic center of glucose-6-phosphatase. HIS176 is the nucleophile forming the phosphohistidine-enzyme intermediate during catalysis The Journal of Biological Chemistry 2002 277:32837-42. [Google Scholar]
[51]. Pal S, Chatterjee AK, Protective effect of N-acetylcysteine against arsenic-induced depletion invivo of carbohydrate Drug Chem. Toxicol 2004 27:179-89. [Google Scholar]
[52]. Turner B, Jenkins E, Kerr D, Sherwin R, Cavan D, The effect of evening alcohol consumption on next-morning glucose control in type 1 diabetes Diabetes Care 2001 24:1888-93. [Google Scholar]
[53]. Meng Z, Bao X, Zhang M, Wei S, Chang W, Li J, Alteration of 11β-hydroxysteroid dehydrogenase type 1 and glucocorticoid receptor by ethanol in rat liver and mouse hepatoma cells J Diabetes Res 2013 2013:218102 [Google Scholar]
[54]. Yuki T, Thurman RG, The swift in alcohol metabolism: Time course for the increase in hepatic oxygen uptake and the involvement of glycolysis Biochem J 1980 186:119-26. [Google Scholar]
[55]. Stephanie M. Wang, Rongqian Wu, The double danger of ethanol and hypoxia: Their effects on a hepatoma cell line Int J Clin Exp Pathol 2009 2:182-89. [Google Scholar]
[56]. Siler S, Neese R, Christiansen M, Hellerstein M, The inhibition of gluconeogenesis following alcohol in humans Am J Physiol Endocrinol Metab 1998 275:E897-907. [Google Scholar]
[57]. Donohue TM Jr, McVicker DL, Kharbanda KK, Chaisson ML, Zetterman RK, Ethanol administration alters the proteolytic activity of hepatic lysosomes Alcohol Clin Exp Res 1994 18:536-41. [Google Scholar]
[58]. Albano E, Alcohol, oxidative stress and free radical damage Proc Nutr Soc 2006 65:278-90. [Google Scholar]
[59]. Rauchenzauner M, Kourtcher J, Ulmer H, Pechalner C, Bellmann R, Wiederman CJ, Disturbances of electrolytes and blood chemistry in acute alcohol intoxication Wier Klin Wochenscher 2005 117:83-91. [Google Scholar]