Utility of Transcutaneous Capnography for Optimization of Non-Invasive Ventilation Pressures
Prashant N Chhajed1, Simone Gehrer2, Kamlesh V Pandey3, Preyas J Vaidya4, Joerg D. Leuppi5, Michael Tamm6, Werner Strobel7
1 Privat Dozent, Department of Pulmonary Medicine, University Hospital Basel, Switzerland.
2 Assistant Arzt, Department of Pulmonary Medicine, University Hospital Basel, Switzerland.
3 Consultant Chest Physician, Insitute of Pulmonology, Medical Research and Development, India.
4 Consultant Chest Physician, Insitute of Pulmonology, Medical Research and Development, India.
5 Professor, Department of Pulmonary Medicine, University Hospital Basel, Switzerland.
6 Professor and Head, Department of Pulmonary Medicine, University Hospital Basel, Switzerland.
7 Oberarzt, Department of Pulmonary Medicine, University Hospital Basel, Switzerland.
NAME, ADDRESS, E-MAIL ID OF THE CORRESPONDING AUTHOR: Dr. Prashant N. Chhajed, Pulmonary Medicine, University Hospital Basel, Petersgraben 4, Basel 4031, Switzerland.
E-mail: prashant.chhajed@lungcare.in
Introduction
Nocturnal Non-invasive Positive Pressure Ventilation (NPPV) is the treatment of choice in patients with chronic hypercapnic respiratory failure due to hypoventilation. Continuous oxygen saturation measured with a pulse oximeter provides a surrogate measure of arterial oxygen saturation but does not completely reflect ventilation. Currently, Partial Pressure of Arterial (PaCO2) measured by arterial blood analysis is used for estimating the adequacy of ventilatory support and serves as the gold standard
Aim
To examine the safety, feasibility and utility of cutaneous capnography to re-titrate the non-invasive positive pressure ventilation settings in patients with chronic hypercapnic respiratory failure due to hypoventilation.
Materials and Methods
Twelve patients with chronic hypercapnic respiratory failure prospectively underwent complete polysomnography and cutaneous capnography measurement on the ear lobe. Non-invasive ventilation pressures were adjusted with the aim of normalizing cutaneous carbon dioxide or at least reducing it by 10 to 15 mmHg. Sensor drift for cutaneous carbon dioxide of 0.7 mmHg per hour was integrated in the analysis.
Results
Mean baseline cutaneous carbon dioxide was 45.4 ± 6.5 mmHg and drift corrected awake value was 45.1 ± 8.3 mmHg. The correlation of baseline cutaneous carbon dioxide and the corrected awake cutaneous carbon dioxide with arterial blood gas values were 0.91 and 0.85 respectively. Inspiratory positive airway pressures were changed in nine patients (75%) and expiratory positive airway pressures in eight patients (66%). Epworth sleepiness score before and after the study showed no change in five patients, improvement in six patients and deterioration in one patient.
Conclusion
Cutaneous capnography is feasible and permits the optimization of non-invasive ventilation pressure settings in patients with chronic hypercapnic respiratory failure due to hypoventilation. Continuous cutaneous capnography might serve as an important additional tool to complement diurnal arterial carbon dioxide tension values.
Introduction
Nocturnal non-invasive NPPV is the treatment of choice in patients with chronic hypercapnic respiratory failure due to hypoventilation [1,2]. NPPV improves partial pressure of arterial oxygen (PaO2), partial pressure of arterial carbon dioxide (PaCO2), dyspnea at rest and on exertion, quality of sleep, daytime somnolence and the quality of life in selected patients with hypercapnic respiratory failure [3]. Continuous oxygen saturation measured with a pulse oximeter provides a surrogate measure of arterial oxygen saturation but does not completely reflect ventilation [4]. Currently, PaCO2 measured by arterial blood analysis is used for estimating the adequacy of ventilatory support and serves as the gold standard.
Non invasive assessment of PaCO2 can be performed by measuring cutaneous carbon dioxide (PcCO2) or end tidal carbon dioxide (PetCO2). In intubated patients or under stable conditions without oral leakage the measurement of PetCO2 in the exhaled air shows an adequate correlation with PaCO2. However, this cannot be achieved during NPPV because of continuous flow through the mask and oral air leakage. Therefore, PcCO2 appears more appropriate for the continuous monitoring of PaCO2. This is based on the observation that carbon dioxide has high tissue solubility and diffuses rapidly through the skin. The accuracy of PcCO2 monitoring during sleep has been debated and the differences in the PcCO2 and PaCO2 have been attributed to methodology in a speculative manner [5]. PcCO2 measurement has been reportedly used during polysomnography studies including when non-invasive ventilation was performed and in critically ill patients [4,6]. Sensor drift with time is an important problem and a technically limiting factor for long term continuous measurements, which leads to discrepancy in the actual PcCO2 and PaCO2 measurement at a given point of time and hence, there is a need to calibrate the PcCO2 measuring sensor at regular intervals [4]. This problem has not been considered as the cause leading to the discrepancy between the PcCO2 and PaCO2 values and a PcCO2 measuring device without a sensor drift that does not need calibration does not exist. To the authors current knowledge there are no studies which have used cutaneous capnography at the ear lobe to specifically titrate ventilator settings in patients with chronic respiratory failure nor studies which have taken into consideration sensor drift for long term measurements.
Recently, PcCO2 measurements have been reported in patients undergoing endoscopy and in the intensive care unit using ear lobe sensors [6–8]. A good correlation between the PcCO2 and PaCO2 measurements has been described with a new digital ear lobe sensor [8,9]. We hypothesized that cutaneous capnography at the ear lobe would permit better titration of ventilator settings during NPPV compared to only diurnal arterial blood gases in patients with chronic hypercapnic respiratory failure due to hypoventilation. Using a sensor at a temperature of 42 degrees Celsius would also permit to do continuous measurements over eight hours without local thermal skin complications [10]. We would also consider correction for sensor drift to reduce the discrepancy between the PcCO2 and PcCO2 values during continuous long-term measurements. The aims of our study were to examine the safety, feasibility and utility of cutaneous capnography to re-titrate the ventilator settings during NPPV in patients with chronic hypercapnic respiratory failure due to hypoventilation and secondly to reassess the appropriateness of existing ventilator settings in patients receiving home ventilation. A re-titration study might be useful as there might be change in the ventilator settings over time since NPPV is often initiated when the patients with chronic hypercapnic respiratory failure present with an acute exacerbation and the ventilator settings at the start of the treatment are often chosen empirically.
Materials and Methods
Study approval was obtained from the ethical commission of the University Hospital Basel Switzerland. Patients gave written informed consent prior to the study. The inclusion criteria were chronic hypercapnic respiratory failure (i.e., hypoventilation syndrome), age>18 years, patients receiving NPPV for at least six months and compliance with NPPV (>5 hours/night). All patients had documented improvement of daytime blood gases, nocturnal oximetry and symptoms of hypoventilation syndrome under current treatment with NPPV. Prior to the titration study, overnight PcCO2 measurements were undertaken in another six patients to assess the extent of signal drift in the PcCO2 measurement during the recorded period. The mean signal drift for PcCO2 was –0.7mm Hg (±0.3) per hour. Therefore, a correction factor (0.7 x the hours of recording) was derived to estimate the total signal drift and was added to the awake PcCO2 value to obtain the drift corrected awake PcCO2 value.
Complete polysomnography (Poly-MEPAL, MAP AG, Germany) was performed in all patients and in addition cutaneous carbon dioxide ear sensor (Sentec AG, Therwil, Switzerland) was placed on the ear lobe as described elsewhere [7,8]. A baseline arterial blood gas was performed, once equilibration of the PcCO2 was reached (typically 5 minutes). Similarly, another blood gas was obtained in the morning at end of the study. Data from pre-study and post study calibrations on individual patients were used to calculate the actual drift of the PcCO2 sensor during the study period. The study night was split in two parts, the first part during which the patients would try to sleep without NPPV for three hours and in the second part using the PcCO2 as a guide to titrate NPPV pressures [11]. If the patient had difficulties in sleeping without NPPV, it was offered before the end of the run in period [11]. NPPV was started with an Inspiratory Positive Airway Pressure (IPAP) of 7cmH2O, and an Expiratory Positive Airway Pressure (EPAP) of 4cmH2O. The pressures were adjusted at an interval of approximately 30 minutes with the goal of reducing the PcCO2 by 10 to 15mmHg from baseline measurement during sleep without NPPV or achieve a PcCO2 between 45 and 50mmHg, whichever occurred first [11]. This target was reached by increasing IPAP (increasing IPAP EPAP difference). EPAP was increased to prevent obstructive apneas and hypopneas as assisted by the polysomnography [12]. The physician deciding about the changes in ventilation pressures was blinded to the pre-existing ventilator settings. Epworth sleepiness score was recorded prior to the study and at follow-up. All patients charted overall satisfaction with home ventilation with regards to symptoms and use of the NPPV on a 10-centimeter visual analogue scale prior to the study and at a follow-up clinic visit six to eight weeks after the study.
Simple descriptive statistics were used [13]. Data were presented as mean (± standard deviation). Pearson’s correlation coefficient was used to assess the correlation between the PcCO2 and PaCO2.
Results
The mean age of the 12 patients was 62 years (range 42 to 73 years) and eight were males. The cause of chronic respiratory failure included different etiologies {(e.g., obesity hypoventilation syndrome, neuromuscular disease, chest wall deformities, Chronic Obstructive Pulmonary Disease (COPD)} [Table/Fig-1]. The baseline clinical characteristics of the patients prior to initiation of NPPV pressures are presented in [Table/Fig-1]. The mean baseline PcCO2 value at start of the study was 45.4 mm Hg (±6.5) and drift corrected for awake PcCO2 value was 45.1 mm Hg (±8.3). The correlation between the baseline PcCO2 at start of the study and parallel PaCO2 was R = 0.91 (95% confidence intervals 0.68 to 0.98). The correlation between the predicted awake PcCO2 and the parallel awake PaCO2 was R = 0.85 (95% confidence intervals 0.48 to 0.96). The mean sensor drift during the study period was –0.8 (± 0.8) mmHg/hour. There were no local complications such as skin redness or burns related to the use of the combined sensor.
Clinical characteristics of study population.
Patient initials | Age(Yrs) | BMI | Diagnosis | SpO2 beforeTreatment | PaCO2 beforetreatment (mmHg) | IPAP(cm H2O) | EPAPcm H2O | NPPV durationuntil study (months) |
---|
FC | 64 | 22.9 | post polio | 88% | 59 | 18 | 4 | 161 |
HE | 70 | 38.3 | OHS COPD | 77 | 78 | 17 | 7 | 13 |
BW | 57 | 40.6 | OHS | 84 | 54 | 16 | 10 | 21 |
CC | 67 | 40.3 | OHS | 84 | 61 | 15 | 5 | 35 |
HW | 65 | 29.4 | OHS COPD | 82 | 55 | 16 | 7 | 93 |
BI | 55 | 39.7 | OHS | 91 | 49 | 12 | 5 | 20 |
WA | 56 | 44.3 | OHS | 94 | 51 | 16 | 6 | 62 |
MM | 60 | 28.7 | Kyphoscoliosis | 94 | 53 | 16 | 5 | 79 |
SJ | 42 | 33.1 | OSAS, opiate therapy | 93 | 48 | 11 | 6 | 7 |
RS | 67 | 34.7 | OHS | 88 | 55 | 18 | 8 | 86 |
GT | 47 | 62.5 | OHS | 83 | 71 | 17 | 8 | 11 |
FA | 73 | 30.8 | Kyphosoliosis | 83% | 54 | 15 | 8 | 49 |
Keys: BMI = body mass index; IPAP = Inspiratory positive airway pressure; EPAP = expiratory positive airway pressure; ESS = Epworth sleepiness score; VAS = visual analogue scale; OHS = obesity hypoventilation syndrome
As a result of the titration study IPAP pressures were changed in nine patients (increased in five; decreased in four) and EPAP in eight patients (increased in four; decreased in four). The mean change in IPAP pressure after the study was 2.3 ± 2.1 cm H20 [Table/Fig-2] and EPAP pressure was 1.5 ± 1.4 cm H20 [Table/Fig-3]. At follow-up, no changes were made to the EPAP pressures; however, minor changes were made to IPAP pressures (not to the pre-study levels) on request for three patients. Patient FC felt that the IPAP pressure of 12cm H20 was too low as he was used to a pressure of 18 cm H20. However, at an IPAP of 12cm H20 nocturnal oximetry and day time blood gases were normal and not different from the results with IPAP 18cm H20. Patients CC and RS felt that the new pressures were too high for them to tolerate. The mean Epworth sleepiness score prior to the study was 8.8 (±3.8) and post study was 8.2 (±3.5) [Table/Fig-4]. The mean visual analogue score prior to the study was 7.0 (±1.5) and post study was 7.4 (±1.4) [Table/Fig-5]. The arterial blood gas values during wakefulness on NPPV treatment prior to the study and at follow-up clinic visit after the study are presented in [Table/Fig-6].
Comparison of inspiratory positive airway pressure before and after study.
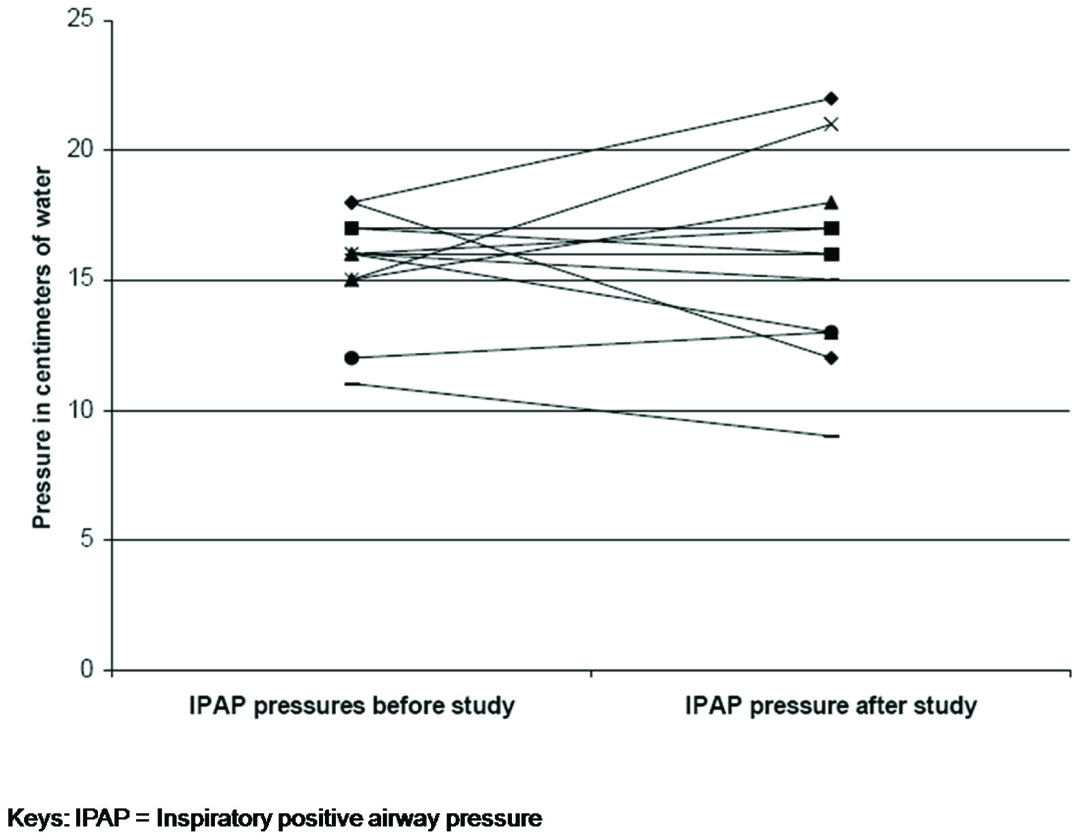
Comparison of expiratory positive airway pressure before and after study.
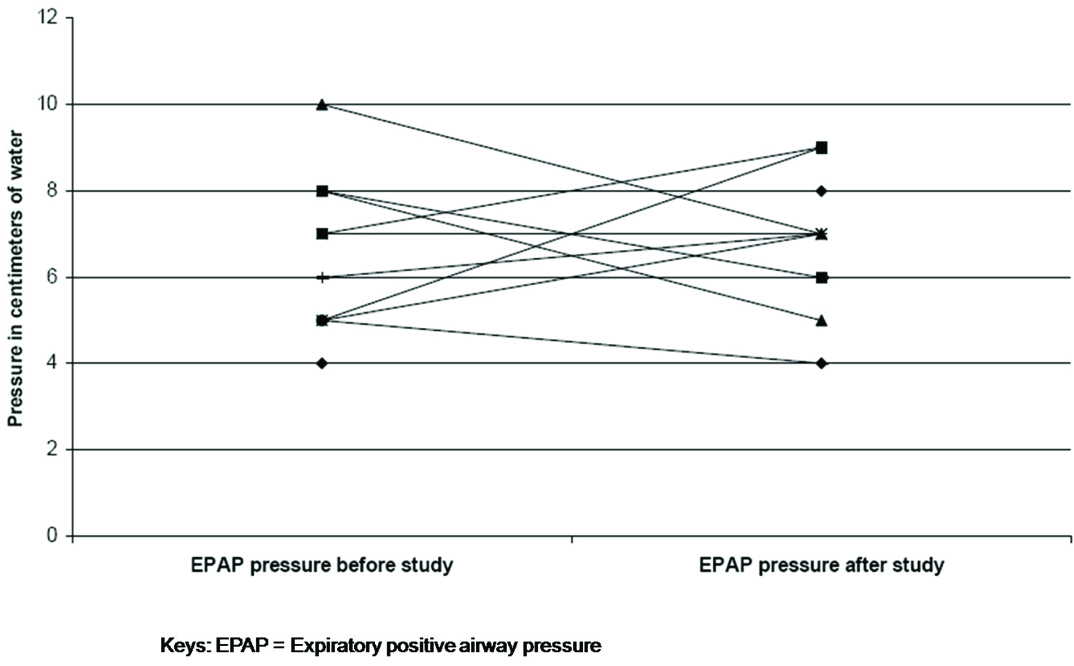
Comparison of Epworth sleepiness score before and after study.
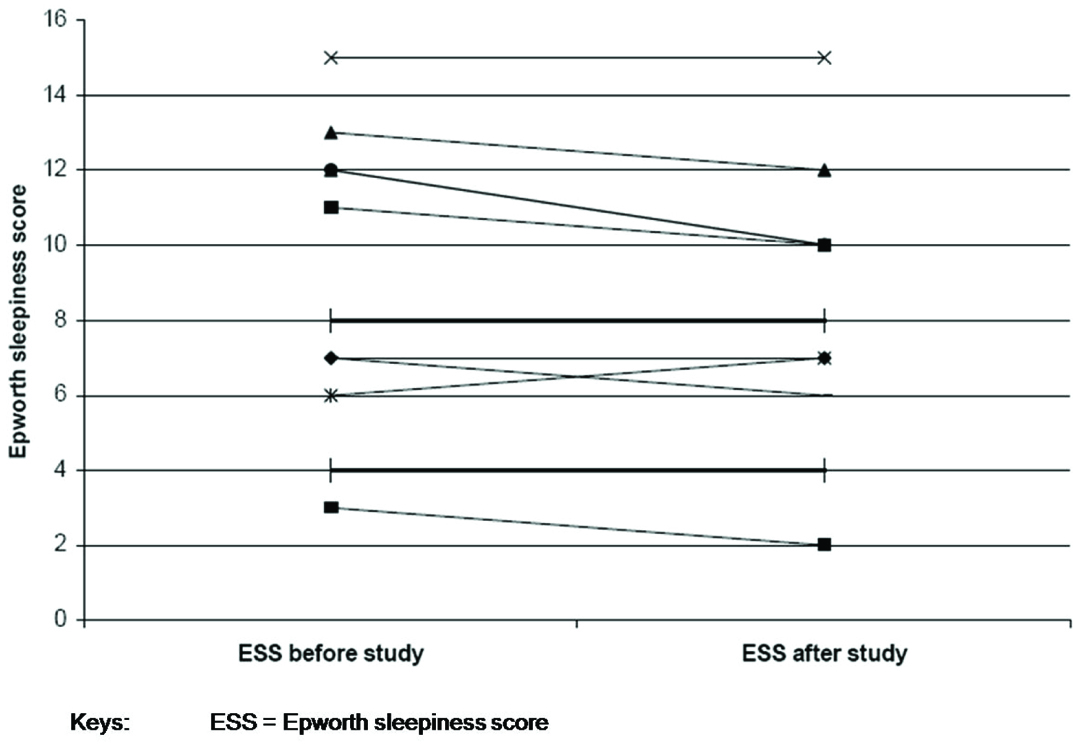
Comparison of visual analogue scale for patient satisfaction with home ventilation before and after study.
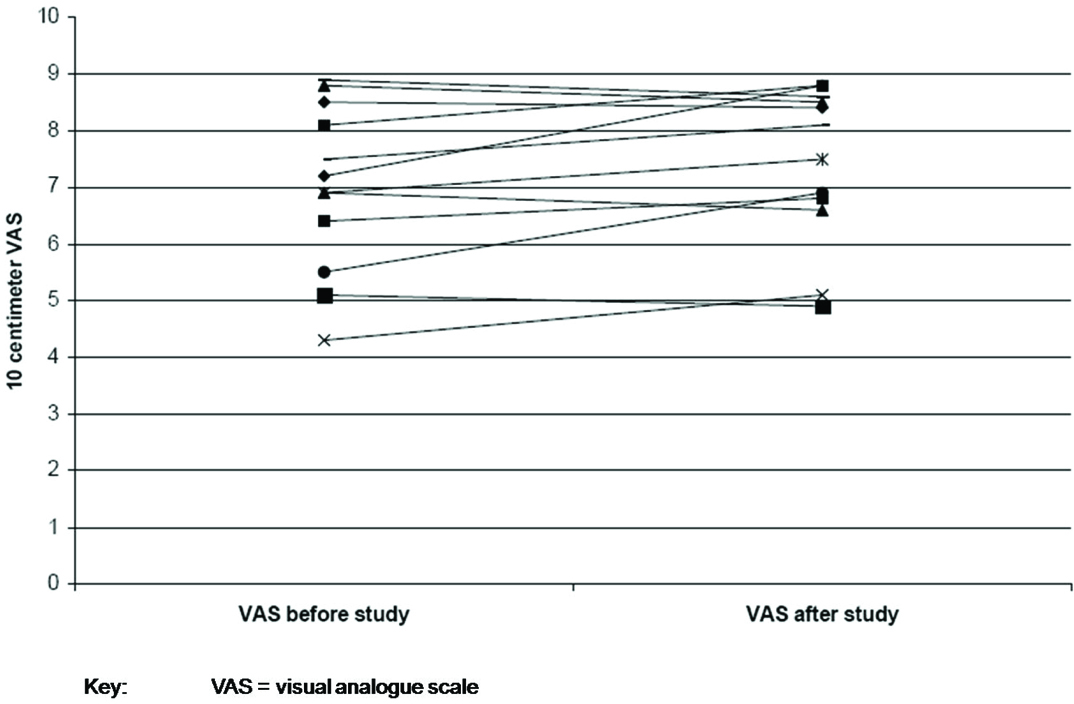
Arterial blood gas values during wakefulness on NPPV treatment prior to the study and at follow-up clinic visit after the study.
Patient Initials | pH | Carbon Dioxide Tension (mmHg) | Oxygen Tension(mmHg) | Oxygen Saturation(%) | Base Excess |
---|
Before Study | After Study | Before Study | After Study | Before Study | After Study | Before Study | After Study | Before Study | After Study |
---|
FC | 7.43 | 7.45 | 44.8 | 42.4 | 70.8 | 71.1 | 94.3 | 94.2 | 4.8 | 1.0 |
HE | 7.40 | 7.45 | 45.7 | 44.1 | 69.7 | 70.3 | 94.5 | 93.2 | 3.0 | 7.2 |
BW | 7.45 | 7.35 | 39.8 | 34.7 | 68.4 | 72.5 | 93.8 | 93.7 | 3.4 | -5.9 |
CC | 7.47 | 7.47 | 45.2 | 49.6 | 50.0 | 54.2 | 83.2 | 87.9 | 7.7 | 11.4 |
HW | 7.36 | 7.39 | 42.7 | 42.1 | 61.8 | 64.0 | 89.6 | 91.4 | -1.5 | 0.2 |
BI | 7.35 | 7.37 | 41.5 | 40.9 | 70.5 | 72.2 | 93.2 | 93 | -2.5 | -2.1 |
WA | 7.39 | 7.45 | 49.1 | 39.3 | 64.4 | 80.8 | 92.6 | 96.5 | 4.1 | 1.1 |
MM | 7.41 | 7.43 | 47.7 | 48.2 | 55.4 | 63.7 | 89.2 | 93 | 1.1 | 6.3 |
SJ | 7.39 | - | 48.1 | - | 73.9 | - | 91.5 | - | 2.4 | - |
RS | 7.41 | 7.45 | 53.7 | 46.5 | 61.7 | 67.9 | 91.1 | 93 | 7.7 | 6.9 |
GT | 7.41 | 7.44 | 43.9 | 41.1 | 75.1 | 80.2 | 93.2 | 95.1 | 1.1 | 0.1 |
FA | 7.35 | 7.35 | 48.4 | 45.2 | 64.6 | 63.4 | 93 | 93.4 | 0.3 | -1.1 |
The mean duration of polysomnography recording on NPPV was 322 minutes (± 58 minutes). As most patients could not sleep well without NPPV, further polysomnography data are presented for the time spent on the mask receiving NPPV. The total sleep time was 216 minutes (± 42 minutes) and sleep efficacy was 70% (±12%) respectively. The mean duration of rapid eye movement sleep was 16% (±10%). The mean oxygen saturation was 91% (±3%) and the mean lowest oxygen saturation was 82% (± 4%). A temporary acute reversible rise (two patients) as well as an acute fall (one patient) in the PcCO2 measurements were noted when the patient lay on the side on which the ear clip was placed.
Discussion
One of the major limiting factors to the continuous long term measurement of PcCO2 has been the need to change the site of measurement at three to four hour intervals to prevent skin burns due to high temperature of the sensor probes (up to 45 degrees Celsius) [14,15]. The recommended maximum duration for which a sensor can be placed cutaneously at 42 degrees Celsius is 8 hours. None of the patients in this study manifested a skin burn.
PcCO2 measurement is a safe and sensitive tool to detect alveolar ventilation in patients receiving nocturnal NPPV for respiratory failure [16,17]. PcCO2 is superior to intermittent measurement of PaCO2 in patients receiving nocturnal NPPV, as it is non invasive and gives a more complete picture about alveolar ventilation [18]. PcCO2 measurement however may underestimate PaCO2 measurement in acute hypercapnic respiratory failure, however, their agreement is reported to be acceptable otherwise [19].
The use of volume targeted bi-level ventilation in obesity hypoventilation syndrome has shown to reduce nocturnal hypercapnia [20]. The findings of our study show the utility of continuous PcCO2 measurements during nocturnal sleep studies in the titration of NPPV pressures in patients with chronic hypercapnic respiratory failure due to hypoventilation. We also introduced a correction factor for the signal drift which is known to occur with such PcCO2 measurement devices. There was a good correlation between the baseline PcCO2 and the baseline arterial carbon dioxide tension (R=0.92) as well as the morning corrected PcCO2 and the morning arterial carbon dioxide tension (R=0.85). To the authors current knowledge this is the first study in which continuous cutaneous capnography could be successfully used to titrate NPPV pressures in patients with hypoventilation. However, titration has to include drift correction. Drift correction of PcCO2 improves the efficacy of PcCO2 monitoring when compared to PaO2 [17]. It is possible that in the future such drift correction measures might already be incorporated in the device.
Most patients could not sleep properly in the first part of the study as they were accustomed to the overnight non invasive home ventilation. However, they could sleep better once they were initiated on NPPV. The sleep efficacy when the patients received NPPV was 70%. This relatively low sleep efficacy compared to the normal population is attributed to the study setting, the effect of sleeping in the sleep laboratory and to intermittent changes in NPPV settings. In two patients there was an acute rise in the PcCO2 and in one an acute fall in PcCO2 as the patient laid on the side where the ear sensor was placed. Sleeping on the side where the ear sensor is placed may lead to compression of the vessels thereby slowing the blood flow in that area. This can lead to stagnation of blood at the site of measurement and thus to an increase in the PcCO2 as the newly generated PcCO2 is not easily flown away by the freely circulating blood. On the other hand, sleeping on the same side where the sensor is placed can also loosen the contact of the sensor with the skin and thus lead to an acute fall in PcCO2. These findings indicate that a protection device around the sensor or the ear lobe could be a useful solution to prevent pressure on the earlobe or avoid slipping of the ear sensor when the patient sleeps or turns on to the side where the sensor is placed. Also, other sites where PcCO2 can be measured are forehead, infraclavicular, chest and anterior abdominal wall [21,22].
The findings of our study show that cutaneous capnography can be used to titrate ventilatory settings in patients with chronic respiratory failure due to hypoventilation. We have chosen to study patients who were already receiving home ventilation so that we could compare the NPPV settings determined in this study to the existing settings of the patients.
Limitation
Several patients included in the study were either being “over” or “under” ventilated based on the study pressures compared to their existing pressures. A small change in the pressure settings can significantly affect tolerability of the patient to bi-level NPPV. Whether quality of life can be substantially improved with adoption of PcCO2 in the titration studies will need a larger randomized trial. Studies examining the utility of this device in patients undergoing first time titration of bi-level ventilation in the sleep laboratory are needed. Patients with additional oxygen supply would be of special interest, since the oximetry in these patients is not helpful for the ventilator settings.
Conclusion
In conclusion, cutaneous capnography using a digital ear lobe sensor is safe and feasible during overnight polysomnography and might serve as a useful additional tool to optimize NPPV settings in patients with chronic hypercapnic respiratory failure due to hypoventilation.
Keys: BMI = body mass index; IPAP = Inspiratory positive airway pressure; EPAP = expiratory positive airway pressure; ESS = Epworth sleepiness score; VAS = visual analogue scale; OHS = obesity hypoventilation syndrome
[1]. Claman DM, Piper A, Sanders MH, Stiller RA, Votteri BA, Nocturnal noninvasive positive pressure ventilatory assistance CHEST Journal 1996 110(6):1581-88. [Google Scholar]
[2]. de Llano LAP, Golpe R, Piquer MO, Racamonde AV, Caruncho MV, Muinelos OC, Short-term and long-term effects of nasal intermittent positive pressure ventilation in patients with obesity-hypoventilation syndrome CHEST Journal 2005 128(2):587-94. [Google Scholar]
[3]. Hillberg RE, Johnson DC, Noninvasive ventilation New England Journal of Medicine 1997 337(24):1746-52. [Google Scholar]
[4]. Chhajed PN, Heuss LT, Tamm M, Cutaneous carbon dioxide monitoring in adults Current Opinion in Anesthesiology 2004 17(6):521-25. [Google Scholar]
[5]. Sanders MH, Kern NB, Costantino JP, Stiller RA, Strollo PJ, Studnicki KA, Accuracy of end-tidal and transcutaneous PCO2 monitoring during sleep CHEST Journal 1994 106(2):472-83. [Google Scholar]
[6]. Senn O, Clarenbach CF, Kaplan V, Maggiorini M, Bloch KE, Monitoring carbon dioxide tension and arterial oxygen saturation by a single earlobe sensor in patients with critical illness or sleep apnea CHEST Journal 2005 128(3):1291-96. [Google Scholar]
[7]. Heuss LT, Chhajed PN, Schnieper P, Hirt T, Beglinger C, Combined pulse oximetry/cutaneous carbon dioxide tension monitoring during colonoscopies: pilot study with a smart ear clip Digestion 2004 70(3):152-58. [Google Scholar]
[8]. Chhajed PN, Kaegi B, Rajasekaran R, Tamm M, Detection of hypoventilation during thoracoscopy: combined cutaneous carbon dioxide tension and oximetry monitoring with a new digital sensor CHEST Journal 2005 127(2):585-88. [Google Scholar]
[9]. Chhajed PN, Langewitz W, Tamm M, (Un) explained hyperventilation Respiration 2006 73(6):825 [Google Scholar]
[10]. Skin temperature at the pulse oximeter probe. In medical and electrical equipment-particular requirements for basic safety and essential performance of pulse oximeter equipment for medical use. Geneva: International Organization for Standardization; 2003-2004. p. Annex BB. 9943-46 [Google Scholar]
[11]. Chhajed PN, Gehrer S, Chhajed TP, Strobel I, Brutsche M, Tamm M, Optimization of non-invasive ventilation pressures using combined oximetry and cutaneous carbon dioxide tension monitoring CHEST Journal 2005 128(4_MeetingAbstracts):382S-S. [Google Scholar]
[12]. Quan S, Gillin JC, Littner M, Shepard J, Sleep-related breathing disorders in adults: Recommendations for syndrome definition and measurement techniques in clinical research. editorials Sleep 1999 22(5):662-89. [Google Scholar]
[13]. Young J, When should you use statistics? Swiss Medical Weekly 2005 135(23/24):337 [Google Scholar]
[14]. Clark JS, Votteri B, Ariagno RL, Cheung P, Eichhorn JH, Fallat RJ, Noninvasive assessment of blood gases The American Review of Respiratory Disease 1992 145(1):220-32. [Google Scholar]
[15]. Freeman ML, Hennessy JT, Cass OW, Pheley AM, Carbon dioxide retention and oxygen desaturation during gastrointestinal endoscopy Gastroenterology 1993 105:331-39. [Google Scholar]
[16]. Aarrestad S, Tollefsen E, Kleiven AL, Qvarfort M, Janssens JP, Skjonsberg OH, Validity of transcutaneous PCO2 in monitoring chronic hypoventilation treated with non-invasive ventilation Respiratory medicine 2016 112:112-18. [Google Scholar]
[17]. Storre JH, Steurer B, Kabitz HJ, Dreher M, Windisch W, Transcutaneous PCO2 monitoring during initiation of noninvasive ventilation Chest 2007 132(6):1810-16. [Google Scholar]
[18]. Storre JH, Magnet FS, Dreher M, Windisch W, Transcutaneous monitoring as a replacement for arterial PCO2 monitoring during nocturnal non-invasive ventilation Respiratory Medicine 2011 105(1):143-50. [Google Scholar]
[19]. Ruiz Y, Farrero E, Cordoba A, Gonzalez N, Dorca J, Prats E, Transcutaneous carbon dioxide monitoring in subjects with acute respiratory failure and severe hypercapnia Respiratory Care 2016 61(4):428-33. [Google Scholar]
[20]. Janssens J-P, Metzger M, Sforza E, Impact of volume targeting on efficacy of bi-level non-invasive ventilation and sleep in obesity-hypoventilation Respiratory Medicine 2009 103(2):165-72. [Google Scholar]
[21]. Chhajed PN, Chaudhari P, Tulasigeri C, Kate A, Kesarwani R, Miedinger D, Infraclavicular sensor site: a new promising site for transcutaneous capnography Scand J Clin Lab Invest 2012 72(4):340-42. [Google Scholar]
[22]. Nishiyama T, Kohno Y, Koishi K, Comparison of ear and chest probes in transcutaneous carbon dioxide pressure measurements during general anesthesia in adults J Clin Monit Comput 2011 25(5):323-28. [Google Scholar]