Introduction
Current clinical detection of Human immunodeficiency virus 1 (HIV-1) is used to target viral genes and proteins. However, the immunoassay, such as viral culture or Polymerase Chain Reaction (PCR), lacks accuracy in the diagnosis, as these conventional assays rely on the stable genome and HIV-1 is a highly-mutated virus. Next generation sequencing (NGS) promises to be transformative for the practice of infectious disease, and the rapidly reducing cost and processing time mean that this will become a feasible technology in diagnostic and research laboratories in the near future. The technology offers the superior sensitivity to detect the pathogenic viruses, including unknown and unexpected strains.
Aim
To leverage the NGS technology in order to improve current HIV-1 diagnosis and genotyping methods.
Materials and Methods
Ten blood samples were collected from HIV-1 infected patients which were diagnosed by RT PCR at Singapore Communicable Disease Centre, Tan Tock Seng Hospital from October 2014 to March 2015. Viral RNAs were extracted from blood plasma and reversed into cDNA. The HIV-1 cDNA samples were cleaned up using a PCR purification kit and the sequencing library was prepared and identified through MiSeq.
Results
Two common mutations were observed in all ten samples. The common mutations were identified at genome locations 1908 and 2104 as missense and silent mutations respectively, conferring S37N and S3S found on aspartic protease and reverse transcriptase subunits.
Conclusion
The common mutations identified in this study were not previously reported, therefore suggesting the potential for them to be used for identification of viral infection, disease transmission and drug resistance. This was especially the case for, missense mutation S37N which could cause an amino acid change in viral proteases thus reducing the binding affinity of some protease inhibitors. Thus, the unique common mutations identified in this study could be used as diagnostic biomarkers to indicate the origin of infection as being from Singapore.
Introduction
HIV-1 virus has high mutation rate since they replicate their copies using RNA polymerase, which could have high errors in the gene copying [1]. This high mutation is very critical for the virus survival and evolution. The mutation rate is responsible for conferring the drug resistance during viral infection in the human host. Because of its high mutation and recombination rates, HIV-1 always exists as a diverse population in hosts. Therefore, many drug-resistance mutations exist as quasispecies at a frequency of less than 20% of the mix [2,3]. Also, it will lead to the variance of viral strains.
To date, viral culture or PCR has been regarded as the standard metric because of its high sensitivity and the fact that it was the method used to detect HIV-1 [4,5]. HIV-1 culture test, also named as peripheral blood mononuclear cell micro-culture assay for HIV-1 isolation, is a laborious laboratory test invitro. The culture test uses an antibody to detect the presence of the viral antigen in saliva, urine or blood [6]. PCR is another preferred method for monitoring the HIV-1 infection, and the specific PCR primer need to be designed according to the specific region of the virus [7]. Thus, the conventional methods like viral culture or PCR, rely on the stable genome of the microbes. However, the mutated HIV-1 strain could be missing the detection by general viral culture methods, due to that it could be more difficult in growing. The mutated DNA will also cause the primer design and classical PCR method to become elusive. Furthermore, the biomarker protein expression will change in the mutated strains. As a result, antibody-based culture test can no longer detect virus accurately [8–10]. Thus, the conventional methods lack robustness in the identification of the mutated strains. This will lead to an urgent need for tools that can rapidly detect and characterize them for infection management.
Next generation sequencing technology is a currently developed DNA/RNA sequencing tool in gene identification [10]. Its current applications include the targeted sequencing, which is used to identify genes that are involved in diseases [10,11]. It is recognized to be a far-reaching technology which can work as a tool for genomic healthcare for patients. NGS has considerable advantages over PCR in terms of improved accuracy of repeated regions, less cost and capability to detect low-frequency alleles [12,13]. A study of pathogenic Chlamydia trachomatis sample has demonstrated that NGS confers 91% perfect matching to reference. The accuracy of 95-100% coverage of a reference bacterial genome had been reached [14]. Another advantage of NGS is that it has the ability to process multiple sequencing reactions, millions in fact, in a parallel fashion. Many fragments of DNA are able to be sequenced at the same time, which allows the sequencing to be much easier with this technological advancement.
Materials and Methods
Clinical Specimens: Blood samples from patients were collected from October 2014 to March 2015 at Singapore Communicable Diseases Centre. The laboratory protocol details have been published [15]. Patient plasma was separated from blood samples and stored at -80oC. An in-house RT-PCR assay was used to diagnose the HIV-1 positive patients [4]. Ten clinical positive samples were chosen for the RNA isolation. Before the isolation, 1 mL of frozen plasma was thawed and was centrifuged at 24,000 x g for an hour at 4°C. A 600 μL of supernatant was discarded and the remained 400 μL of plasma containing the viral particles were used for viral RNA isolation using the Magna Pure Compact Nucleic Acid Isolation kit (Roche Applied Science, Switzerland), according to the manufacturer’s manual. RNA was subsequently eluted in 50 μL of the kit elution buffer. After RNA isolation, RNA samples were reverse-transcribed into their complementary DNAs (cDNA) using the One-Step RT-PCR kit (QIAGEN, Valencia, US). The amplified primers were as following: forward primer: 5’- GAA CAG ACC AGA GCC AAC AGC CCC ACC-3’ from HIV-1 genome positions 2139 to 2165; reverse primer II:5’-TTT GAC TTG CCC AAT TTA GTT TTC CCA C-3’and II: 5’- TTT GAC TTG CCC AAT TTA ACT TTC CCA C-3’ from HIV-1 genome positions 3330 to 3357 [4,16].
DNA clean up: cDNA was cleaned by PCR Purification Kit (QIAGEN, Valencia, US) [17] as stated by the vendor’ instructions. Different concentration of the purified DNA samples was then quantified using the Picogreen [18].
DNA concentration quantification: cDNA sample concentration was quantified with Quant-iT Picogreen dsDNA Reagent following the company’s suggested protocol (Thermo Fisher Scientific, Grand Island, USA). A 50 μL of the standards and samples were added into wells of the Greiner 96 flat bottom black polystyrol plate. The samples were aliquotted into the wells in triplicates. Then they were mixed by tapping plate gently and after that 50μL of diluted Picogreen were added to each plate well. Then the plate was incubated in the dark for 2~5 minutes before being sent for the fluorescence reading with the excitation wavelength at 480nm and the emission wavelength at 520nm.
Agarose gel electrophoresis: A 1.2% agarose (Thermo Fisher Scientific, Grand Island, US) gel was cast. 1.2 g of agarose powder was added to 100 mL of 1X TAE buffer. A 2 μL of Gel Red nucleic acid gel stain (Biotium Inc. Hayward, US) was also added after the mixture was completely dissolved by heating. A 3 μL of 6 X loading dye was mixed with 15 μL of the amplified DNA templates and loaded into the wells of the gel. DNA markers were used to estimate the size of the amplified DNA. The agarose gel was run in TAE buffer using the constant electric flow of 100 V for 90 mins [19].
NGS DNA library construction: A 1ng DNA was used, and Nextera enrichment sample was followed for the NGS library construction (Illumina, San Diego, US) [20]. Thirteen cycles of PCR were applied for sample DNA amplification. Once the PCR programme was completed, a real-time PCR run was done on the amplified product to ensure that the target of interest has been amplified followed by agarose gel running to check for the bands. The samples were put into the ABI 7500 Fast Real-Time PCR System following the thermocycling program.
Library pooling and sequencing: As preparation, a heat block was set at 96°C, the sequencing reagent cartridge was thawed at room temperature and an ice bucket was prepared by combining 3 parts ice and 1 part water. A new Eppendorf tube was used to pool DNA library and 5 μL of the mixture from each DNA sample were transferred into the pool tube, followed by pipetting up and down 5 times to mix the sample. Following that, the pool tube was incubated using a heat block, at 96oC for 2 minutes, inverted two times to mix, and then immediately placed into the ice box for 5 minutes. After incubation, the contents of DNA pool library were loaded into the thawed reagent cartridge’s sample reservoir and placed into the MiSeq machine (Illumina, San Diego, US) [21] for sequencing as multiplex two read libraries for 318 cycles (including additional 16 cycles of index reads).
Data analysis: The quality of the sequencing results was verified by Sequencing Analysis Viewer (Illumina, San Diego, USA) and by resequencing to reference sequences using Illumina MiSeq Reporter. The HIV-1 reference sequence (Accession No. AF033819.3) is from GeneBank. The results were further aligned for quality and variance by website tool of the IGV (Integrative Genomics Viewer, the Broad institute) [22]. The identified HIV-1 mutations were further examined through Basic Local Alignment Search Tools (BLAST) from National Centre for Biotechnology Information (NCBI). The common mutations were also evaluated as potential biomarkers in disease transmission, pathogen prevalence and drug resistance.
Results
HIV-1 samples VI and VII were chosen for the gel electrophoresis to ensure that the DNA libraries were successfully fragmented and tagmented [Table/Fig-1]. The smear seen in the respective lanes in the agarose gel indicates that the longer cDNA fragments had been effectively fragmented into smaller pieces between 200 and 500 bp, as indicated by a smear through its lane [Table/Fig-1]. The size of these DNA pieces was compatible with our sequencing platform.
Agarose Gel Electrophoresis of HIV-1 sample VI and sample VII. Lane 1, ultra-low DNA ladder; Lanes 2 & 3, sample VI and sample VII before sequencing library construction; Lanes 4 & 5, sample VI and sample VII after sequencing library construction; Lane 6, 1 kb DNA ladder.
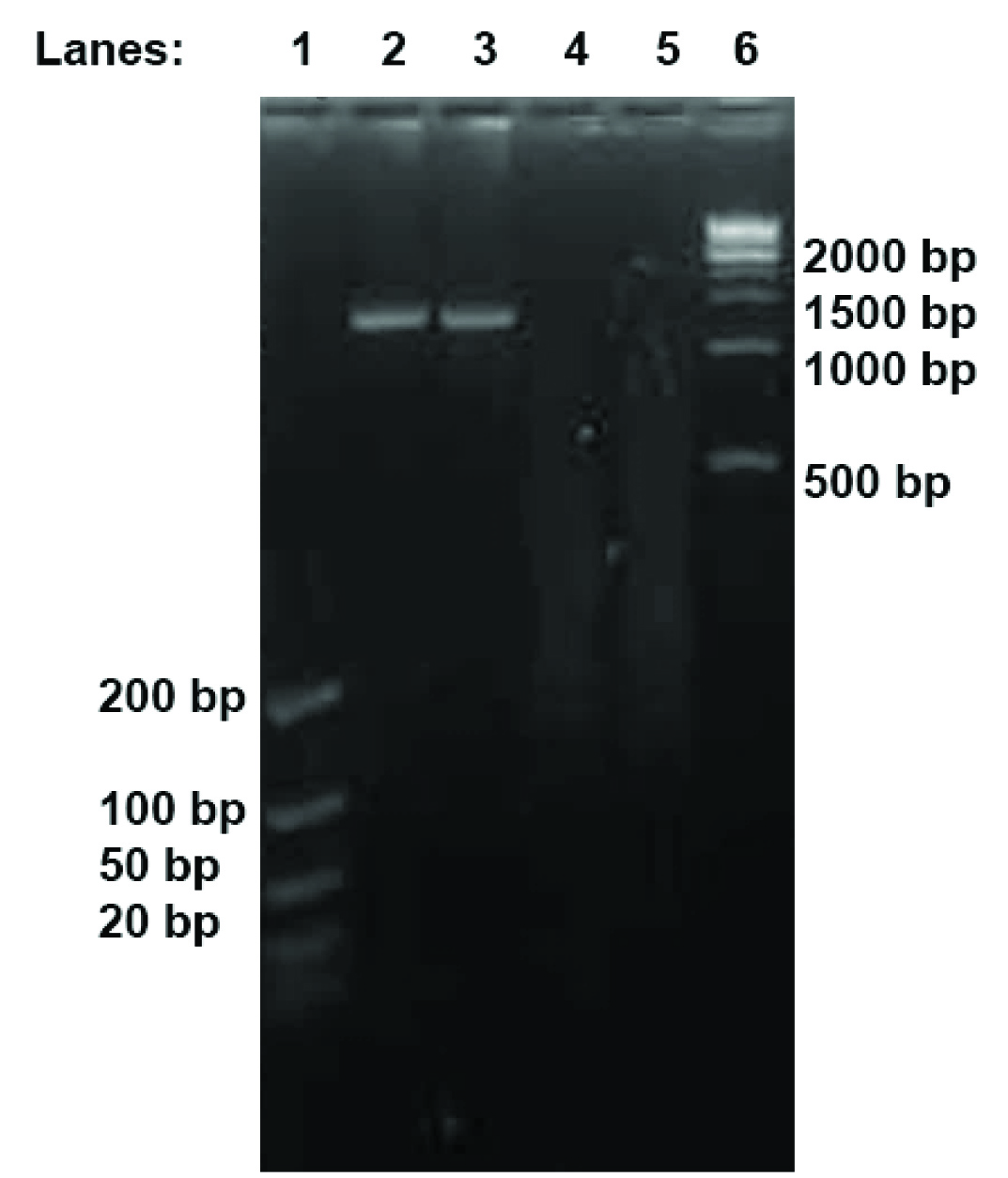
NGS confers 97.3% of the reads identified. The sequencing quality of the base calling from the resulting images was verified by quality score (Q). 95.1% of the reading sequencing results was ≥Q30, which represents more than 99.9% accuracy for the base calling. 96.68% of Read 1 and 93.46% of Read 2 sequences were ≥Q30. In comparison with the HIV-1 reference genome, we were able to identify the mutations through the sequencing analysis.
The nucleotides indicated on the fragments were the mutated bases that were different to the reported bases in the HIV-1 reference genome. Based on our results [Table/Fig-2&3], only the mutated bases with at least 90 percent base mutations corresponding to the HIV-1 reference genome were recorded. The mutated bases from all ten sequenced HIV-1 samples were collated. There were various mutations identified from our HIV-1 NGS results. In order to find the common mutation among the ten HIV-1 samples, all the mutations for the ten HIV-1 samples were aligned and compared with the reference genome. Ten individual HIV-1 sequencing and analysing results are summarized in the tables [Table/Fig-2&3]. Two common mutations were observed in all ten samples and were identified at the genome position 1908 and 2104.
The nucleotide change at position 1908 among ten samples.
Sample ID | Original Base | Mutated Base | Percentage | No of base counted |
---|
I | G | A | 97% | 799 |
II | G | A | 97% | 1129 |
III | G | A | 99% | 5418 |
IV | G | A | 97% | 14175 |
V | G | A | 100% | 36 |
VI | G | A | 100% | 175 |
VII | G | A | 99% | 595 |
VIII | G | A | 99% | 14218 |
IX | G | A | 99% | 73364 |
X | G | A | 100% | 13357 |
The nucleotide change at position 2104 among ten samples.
Sample ID | Original Base | Mutated Base | Percentage | No of base counted |
---|
I | C | T | 94% | 216 |
II | C | T | 97% | 156 |
III | C | T | 100% | 146 |
IV | C | T | 99% | 26749 |
V | C | T | 100% | 21 |
VI | C | T | 93% | 43 |
VII | C | T | 92% | 161 |
VIII | C | T | 99% | 38452 |
IX | C | T | 98% | 21067 |
X | C | T | 93% | 43 |
At HIV-1 genome position 1908 [Table/Fig-2], there was a base change from Guanine (G) to Adenine (A). The base change A1908G causes a missense mutation as the codon changes from AGT to AAT. This results in the substitution of amino acid from Serine (S) to Asparagine (N). The novel missense point mutation of S38N was discovered in our studies. In addition, at HIV-1 genome position 2104 [Table/Fig-3], a common base change of Cytosine (C) to Thymine (T) was identified in the HIV-1 samples as well. These two common mutations are located at HIV-1 gag-pol region. There are other many random mutations in the HIV-1 genome which arise due to selective pressure. Random mutations refer to any mutations that have more than 90% mutated base that can be found at any HIV-1 genome location. For example, the random mutations at 1870 were only identified in Sample I, but not in the other nine samples [Table/Fig-4]. The finding of the random mutations demonstrates that the overall sequencing was correct and the common sequencing results were not due to cross contamination.
The nucleotide change at position 1870 among ten samples.
Sample ID | Original Base | Mutated Base | Percentage | No of base counted |
---|
I | G | A | 97% | 554 |
II | G | G | 100% | 799 |
III | G | G | 95% | 1816 |
IV | G | G | 100% | 17862 |
V | G | G | 97% | 156 |
VI | G | G | 99% | 232 |
VII | G | G | 100% | 1861 |
VIII | G | G | 100% | 20054 |
IX | G | G | 100% | 40417 |
X | G | G | 100% | 7207 |
Discussion
The advent of NGS technologies offering different chemistries, simplified sample preparation, faster processing times, and reduced cost per base pair sequenced prompted us to translate currently available NGS technology into clinical diagnostics for HIV-1 studies. HIV-1 genomes had been previously sequenced through Roche 454 pyrosequencing in the years 2012 [23] and 2013 [24], and some mutations were discovered in this deadly virus. Here, we used Illumina Miseq platform to sequence the HIV-1 clinical samples. We took advantage of Nextera library construction technology, which allows NGS a tiny amount input cDNA (1ng) as opposed to general amplicon methodology (500ng) [23]. Thus, the amplification cycles in the library preparation will be fewer and the error from the PCR-induced amplification and recombination will be minimized [25]. Furthermore, in the previous studies, the HIV-1 samples were from America and UK [23,24]. As HIV-1 is a globally spreading pathogen and there are various HIV-1 subtypes in different regions around the world, it is conceivable that there would exist different mutations in the other subtypes. In this study, we sequenced ten HIV-1 clinical strains isolated in Singapore. Our NGS results demonstrated that no two virions were exactly identical among the various clinical samples. This suggests that HIV-1 always exists as a diverse population, due to its high mutation and recombination rates. This is in consistent with other findings that have shown that HIV-1 is one of the highest-mutated virus [23,26]. More importantly, as compared to their Western counterparts, two specific common mutations were found here in the HIV-1 pol gene [Table/Fig-5]. Since the pol gene itself consists of 3 factors for viral replication and is one of the least variable regions, it has been the main target for disease diagnosis and drug development [27].
The graphic with various genes present in gag-pol in the given reference HIV-1 genome. The two common mutations at the position 1908 and 2104 were highlighted in the green and blue respectively.
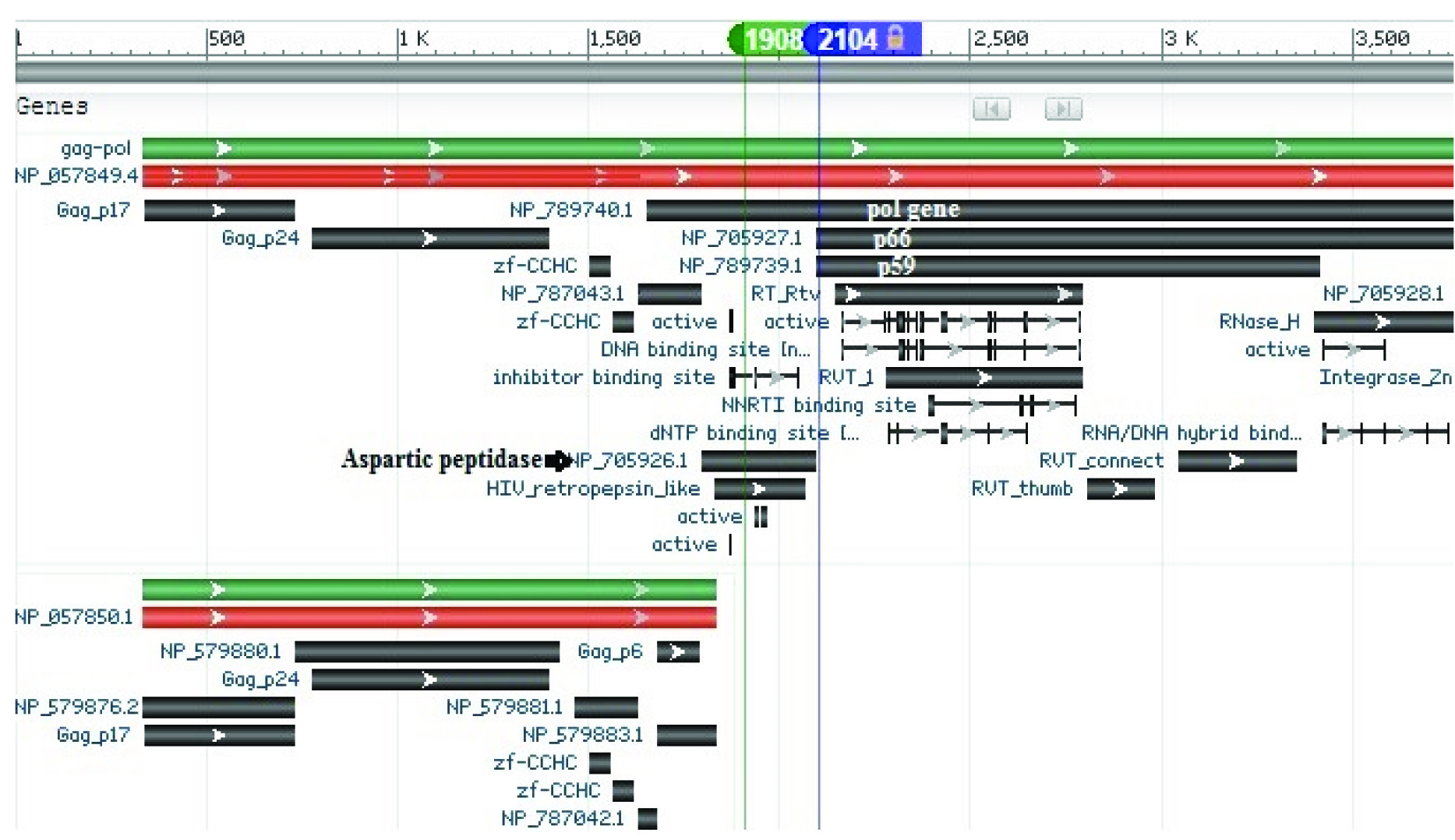
The missense mutation identified at HIV-1 genome position 1908 is located in the pol gene coding for aspartic peptidase, leading to the mutation at S37N on the virus’s aspartic proteinase and S93N on the pol peptide [Table/Fig-5]. Aspartic protease is responsible for the cleavages in the Gag-Pol polyprotein. Antiviral drugs like ritonavir and saquinavir are protease inhibitors which cause the dysfunction of these enzymes, and ultimately stop viral replication [28]. The missense mutation at genome position 1908 confers to the mutation at the codon S37N of the aspartic protease gene. The mutation identified would have led to a change in the conformation of the enzyme which allows the potential resistance to the inhibitory effects of the drug and encourages the expansion of HIV-1 population in the hosts. The location of this common mutation has not been reported in other papers [23,29,30]; of those reported, these were at amino acid codons 24, 32, 46, 47, 48, 50, 54, 76, 82, 84, 88, 90 from the Stanford HIV Drug resistance Database [31]. Therefore, the identification of the mutation at a new location may suggest that there may be an emergence of a potentially new resistant HIV-1 strain.
Another mutation at genome position 2104 which leads to a silent mutation on reverse transcriptase subunits was also identified from our results. This mutation was within the reverse transcriptase subunits, p51 and p66, which leads to mutation at S3S on the p51/p66 reverse transcriptase subunits and S158S on the Pol peptide. The p51 subunit is encoded in the first 440 amino acids of the p66 subunit which corresponds, although not precisely, to the polymerase domain while the rest of the p66 contains the RNAse H (enzymatic) domain [26]. Generally, the coding of the same amino acid through silent mutation would maintain the protein sequence of the enzyme, hence preserving its activity. However, silent mutations can also lead to the altered folding pattern of mRNA, and thus alter the translational efficiency and protein expression level [32]. This minor alteration in protein structure has been observed in particular cancers in humans. The silent mutation in the MDR gene at exon 26 produces polymorphic P-glycoprotein which enables the effective pumping of chemotherapy drugs out of the cell [33]. The common mutation identified codes for the same amino acid, and therefore it is highly possible that the HIV-1 virus from Singapore is easily mutated at this position under selection pressure, such as the host defense systems in local patients. The presence of this pressure may have been the stimulus for the virus to develop its survival ability by mutation and at the same time preserve the activity of its viral enzymes. As a result, the identification of these common mutations could be used as diagnostic biomarkers for the strain of HIV-1 in Singapore. As these common mutations are different compared to the reference genome and are only identified in virus from Singapore, this makes them promising biomarkers for local HIV-1 strains. Additionally, the HIV-1 strain with its unique mutated location could have resulted from drug interactions with the unique immunologic response of the local patients [34]. During antiviral drug treatment, most HIV-1 viruses can be killed. However, if a drug-resistant HIV-1 strain is present, even in small numbers, it can proliferate and dominate the HIV-1 viral pool [35]. This information could be used as evidence for health agencies to put in place more stringent control measures that can slow the transmission of the virus. The findings of the study suggest that the identification of these common mutations could be used as diagnostic biomarkers for the strain of HIV-1 in Singapore and further studies are required to confirm the same. In the future work, we need to confirm that these common mutations are not predominantly found in other Asian countries, therefore they could be potential biomarkers which enable the tracing of HIV-1 strains that originate within the country.
Conclusion
In essence, NGS gives vastly superior information for the accurate detection of HIV-1 genomic mutations. Thus, NGS could set to be the next standard of healthcare method for HIV-1. This study outcome holds great potential for helping to provide clinicians with deeper insights into disease diagnosis and pathogen transmission. This information can serve as an indicator that allows the monitoring of the spread of HIV-1 infections based on their origin of infection. The assumption would be that the spread of HIV-1 strains carrying these common mutations is localized within Singapore. Additionally, the novel common mutation identified from this study would also be helpful to examine their involvement in conferring antiviral drug resistance in HIV-1.
Contribution
The First, Second and Third Author (WHS, QL & ZZM) contributed in performing research and writing manuscript. The Fourth, Fifth and Sixth Author (JJL, WXK & OTN) contributed in providing sample and discussing result. The Seventh and Eighth Author (ELT & PL) contributed in heading project, designing research and proof reading this manuscript.
[1]. Cuevas JM, Geller R, Garijo R, Lopez-Aldeguer J, Sanjuan R, Extremely High Mutation Rate of HIV-1 In Vivo PLoS Biol 2015 13(9):e1002251 [Google Scholar]
[2]. Bansode V, McCormack GP, Crampin AC, Ngwira B, Shrestha RK, French N, Characterizing the emergence and persistence of drug resistant mutations in HIV-1 subtype C infections using 454 ultra deep pyrosequencing BMC Infect Dis 2013 13:52 [Google Scholar]
[3]. Bush S, Tebit DM, HIV-1 Group O Origin, Evolution, Pathogenesis, and Treatment: Unraveling the Complexity of an Outlier 25 Years Later AIDS Rev 2015 17(3):147-58. [Google Scholar]
[4]. Kaur P, Khong WX, Wee SY, Tan EL, Pipper J, Koay E, Clinical evaluation of a low cost, in-house developed real-time RT-PCR human immunodeficiency virus type 1 (HIV-1) quantitation assay for HIV-1 infected patients PLoS One 2014 9(3):e89826 [Google Scholar]
[5]. Burgard M, Mayaux MJ, Blanche S, Ferroni A, Guihard-Moscato ML, Allemon MC, The use of viral culture and p24 antigen testing to diagnose human immunodeficiency virus infection in neonates. The HIV Infection in Newborns French Collaborative Study Group N Engl J Med 1992 327(17):1192-97. [Google Scholar]
[6]. Jackson JB, Coombs RW, Sannerud K, Rhame FS, Balfour HH, Jr, Rapid and sensitive viral culture method for human immunodeficiency virus type 1 J Clin Microbiol 1988 26(7):1416-18. [Google Scholar]
[7]. Hopkins M, Hau S, Tiernan C, Papadimitropoulos A, Chawla A, Beloukas A, Comparative performance of the new Aptima HIV-1 Quant Dx assay with three commercial PCR-based HIV-1 RNA quantitation assays J Clin Virol 2015 69:56-62. [Google Scholar]
[8]. Schito ML, D’Souza MP, Owen SM, Busch MP, Challenges for rapid molecular HIV diagnostics J Infect Dis 2010 201(Suppl 1):S1-6. [Google Scholar]
[9]. Boonham N, Kreuze J, Winter S, van der Vlugt R, Bergervoet J, Tomlinson J, Methods in virus diagnostics: from ELISA to next generation sequencing Virus Res 2014 186:20-31. [Google Scholar]
[10]. Behjati S, Tarpey PS, What is next generation sequencing? Arch Dis Child Educ Pract Ed 2013 98(6):236-38. [Google Scholar]
[11]. Grada A, Weinbrecht K, Next-generation sequencing: methodology and application J Invest Dermatol 2013 133(8):e11 [Google Scholar]
[12]. da Fonseca AJ, Galvao RS, Miranda AE, Ferreira LC, Chen Z, Comparison of three human papillomavirus DNA detection methods: Next generation sequencing, multiplex-PCR and nested-PCR followed by Sanger based sequencing J Med Virol 2016 88(5):888-94. [Google Scholar]
[13]. Tuononen K, Maki-Nevala S, Sarhadi VK, Wirtanen A, Ronty M, Salmenkivi K, Comparison of targeted next-generation sequencing (NGS) and real-time PCR in the detection of EGFR, KRAS, and BRAF mutations on formalin-fixed, paraffin-embedded tumor material of non-small cell lung carcinoma-superiority of NGS Genes Chromosomes Cancer 2013 52(5):503-11. [Google Scholar]
[14]. Christiansen MT, Brown AC, Kundu S, Tutill HJ, Williams R, Brown JR, Whole-genome enrichment and sequencing of Chlamydia trachomatis directly from clinical samples BMC Infect Dis 2014 14:591 [Google Scholar]
[15]. Ng OT, Munshaw S, Lamers SL, Chew KK, Lin L, Redd AD, Molecular epidemiology of HIV type 1 in Singapore and identification of novel CRF01_AE/B recombinant forms AIDS Res Hum Retroviruses 2011 27(10):1135-37. [Google Scholar]
[16]. Chew KK, Ng KY, Khong WX, Kaur P, Yap JK, Chua A, Clinical evaluation of an in-house human immunodeficiency virus (HIV) genotyping assay for the detection of drug resistance mutations in HIV-1 infected patients in Singapore Ann Acad Med Singapore 2012 41(12):553-58. [Google Scholar]
[17]. He Y, Vogelstein B, Velculescu VE, Papadopoulos N, Kinzler KW, The antisense transcriptomes of human cells Science 2008 322(5909):1855-57. [Google Scholar]
[18]. Dragan AI, Casas-Finet JR, Bishop ES, Strouse RJ, Schenerman MA, Geddes CD, Characterization of PicoGreen interaction with dsDNA and the origin of its fluorescence enhancement upon binding Biophys J 2010 99(9):3010-19. [Google Scholar]
[19]. Koontz L, Agarose gel electrophoresis Methods Enzymol 2013 529:35-45. [Google Scholar]
[20]. Schirmer M, Ijaz UZ, D’Amore R, Hall N, Sloan WT, Quince C, Insight into biases and sequencing errors for amplicon sequencing with the Illumina MiSeq platform Nucleic Acids Res 2015 43(6):e37 [Google Scholar]
[21]. Caporaso JG, Lauber CL, Walters WA, Berg-Lyons D, Huntley J, Fierer N, Ultra-high-throughput microbial community analysis on the Illumina HiSeq and MiSeq platforms ISME J 2012 6(8):1621-24. [Google Scholar]
[22]. Robinson JT, Thorvaldsdottir H, Winckler W, Guttman M, Lander ES, Getz G, Integrative genomics viewer Nat Biotechnol 2011 29(1):24-26. [Google Scholar]
[23]. Gall A, Ferns B, Morris C, Watson S, Cotten M, Robinson M, Universal amplification, next-generation sequencing, and assembly of HIV-1 genomes J Clin Microbiol 2012 50(12):3838-44. [Google Scholar]
[24]. Di Giallonardo F, Zagordi O, Duport Y, Leemann C, Joos B, Kunzli-Gontarczyk M, Next-generation sequencing of HIV-1 RNA genomes: determination of error rates and minimizing artificial recombination PLoS One 2013 8(9):e74249 [Google Scholar]
[25]. Heavens D, Accinelli GG, Clavijo B, Clark MD, A method to simultaneously construct up to 12 differently sized Illumina Nextera long mate pair libraries with reduced DNA input, time, and cost Biotechniques 2015 59(1):42-45. [Google Scholar]
[26]. Hu WS, Hughes SH, HIV-1 reverse transcription Cold Spring Harb Perspect Med 2012 2(10) [Google Scholar]
[27]. Arts EJ, Hazuda DJ, HIV-1 antiretroviral drug therapy Cold Spring Harb Perspect Med 2012 2(4):a007161 [Google Scholar]
[28]. Falkensammer B, Fasser W, Scherer K, Zemann A, Parson W, Ulmer H, Drug monitoring and viral response to lopinavir/ritonavir or saquinavir/ritonavir containing regimens in individuals infected with the human immunodeficiency virus type 1 Int J Immunopathol Pharmacol 2005 18(1):145-54. [Google Scholar]
[29]. Telwatte S, Hearps AC, Johnson A, Latham CF, Moore K, Agius P, Silent mutations at codons 65 and 66 in reverse transcriptase alleviate indel formation and restore fitness in subtype B HIV-1 containing D67N and K70R drug resistance mutations Nucleic Acids Res 2015 43(6):3256-71. [Google Scholar]
[30]. Harrigan PR, Sheen CW, Gill VS, Wynhoven B, Hudson E, Lima VD, Silent mutations are selected in HIV-1 reverse transcriptase and affect enzymatic efficiency AIDS 2008 22(18):2501-08. [Google Scholar]
[31]. Database HDR. Major HIV-1 Drug Resistance Mutations 2015 [updated March 09, 2015]. Available from: http://hivdb.stanford.edu/pages/download/resistanceMutations_handout.pdf [Google Scholar]
[32]. Shabalina SA, Spiridonov NA, Kashina A, Sounds of silence: synonymous nucleotides as a key to biological regulation and complexity Nucleic Acids Res 2013 41(4):2073-94. [Google Scholar]
[33]. Kuritzkes DR, HIV-1 subtype as a determinant of disease progression J Infect Dis 2008 197(5):638-39. [Google Scholar]
[34]. Shafer RW, Genotypic testing for human immunodeficiency virus type 1 drug resistance Clin Microbiol Rev 2002 15(2):247-77. [Google Scholar]
[35]. Shafer RW, Schapiro JM, HIV-1 drug resistance mutations: an updated framework for the second decade of HAART AIDS Rev 2008 10(2):67-84. [Google Scholar]