Bacterial Heat Shock Protein Activity
Farajollah Maleki1, Afra Khosravi2, Ahmad Nasser3, Hamid Taghinejad4, Mitra Azizian5
1 Lecturer, Clinical Microbiology Research Center, Ilam University of Medical Science, Ilam, Iran.
2 Professor, Department of Immunology, Faculty of Medicine, Ilam University of Medical Sciences, Ilam, Iran.
3 Lecturer, Clinical Microbiology Research Center, Ilam University of Medical Science, Ilam, Iran.
4 Assistant Professor, Department of Nursing, Faculty of Nursing and Midwifery, Ilam University of Medical Sciences, Ilam, Iran.
5 Lecturer, Clinical Microbiology Research Center, Ilam University of Medical Science, Ilam, Iran.
NAME, ADDRESS, E-MAIL ID OF THE CORRESPONDING AUTHOR: Dr. Mitra Azizian, Clinical Microbiology Research Center, Ilam University of Medical Science, Ilam, Iran. E-mail : mitra_azizian@yahoo.com
Bacteria are exposed to different types of stress in their growth conditions. They have developed appropriate responses, modulated by the re-modeling of protein complexes and by phosphorylation dependent signal transduction systems, to adapt and to survive in a variety range of nature. Proteins are essential components for biologic activity in the eukaryotic and prokaryotic cell. Heat Shock Proteins (HSP) have been identified from various organisms and have critical role in cell hemostasis. Chaperone can sense environment and have different potential role in the organism evolution.
Bacterial chaperone,Heat shock protein90,Chaperone DnaK,Heat shock protein GroEL
Introduction
The Heat-shock response involves the induction of many proteins called heat-shock proteins, or HSP in response to variations of temperature. The HSPs include chaperones and proteases that are presumably essential for overcoming changes that involve protein denaturation. HSP plays an important role in pathogenesis [1]. In Escherichia coli the heat-shock response is controlled by a specific sigma factor-32 (σ32), this factor coded by the rpoH gene and binds to heat-shock promoters located upstream of heat-shock genes [2]. Many molecular chaperones have developed to control protein folding and protection of the cell from accelerating temperature [3]. HSP include chaperones and proteases that are necessary for maintaining the cell, in abnormal condition such protein denaturation.
The 90-kDa HSP are member of molecular chaperones family that are wide spread in prokaryote and eukaryote cell [4]. 90-KDa Heat Shock Protein (HSP90) is conserved from bacteria to eukaryotic cell with approximate 50% sequence similarity between E.coli and humans [5]. HSP90 has a low ATPase activity causing large conformational changes of HSP90 that cause structural changes to bound substrate protein and mediate release of nascent protein from the chaperone [6]. HSP proteins have a major role in cellular functions, such as protein trafficking, receptor maturation and signal transduction [7]. HSP90 proteins are dimeric and with each protomer contain three conserved domains: N-terminal domain that binds and hydrolyzes ATP, a C-terminal and middle domain that is important for dimerization [8]. HSP90 has kinase activity and nuclear receptor ligand binding by an unknown mechanism [9]. This activity has suggested that HSP90 can interact with unfolded or non-native protein.
Activity role of HSP90: Cyanobacterial HSP90 fix particular protein that is required for the assembly of protein complexes called phycobilisomes [10]. Cells abrogated to induce heat shock protein to eliminate the related transcription factor, cause accumulation of large protein aggregates in bacterial cell [11]. The major classes of HSPs called chaperones have ability to identify non-native proteins such as unfolded protein. In abnormal conditions such thermal stress, bacterial HSP90 has crucial role in protein folding [12]. HSP90 is utilize the ATP in the N-terminal domains and deform as then make contacts with the middle domain [13]. Only if the ATP utilized, HSP90 returns to the open state. HSP90 is involved in assembly group of macromolecular protein that have major role in scaffolding proteins [13]. HSP90 dimer is compound of three stable domains N-terminal domain, middle domain and C-terminal domain [Table/Fig-1]. ATP hydrolyzed at the N-terminal and dimerization occurs at the C-terminal.
Outline of HSP90, N-terminal: binding site of ATP, middle domain: contribute in dimerization and C-terminal: Dimerization
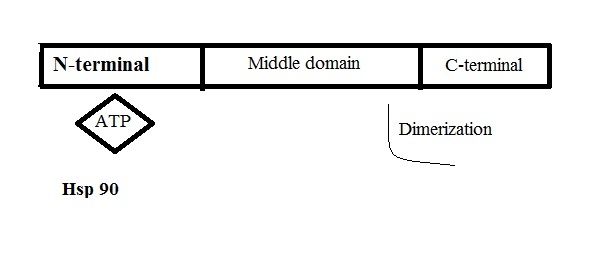
HSP90 have kinase activity and nuclear receptor ligand binding by an unknown pathway [9]. HSP90 is a molecular chaperone known to elevate the folding, refolding and activation of a broad range of protein substrates. This modulation suggested that HSP90 can interact with unfolded substrates that are delayed in their folding pathway [3]. HSP90 can actively effect the combination of bound substrates, which could modify the substrate folding process and cause functional outcome. If the HSP90 absence, recovery of cells from a heat shock is delayed, and this retard can be eliminated by overproduction of HSP90 [14]. Chaperones can fold the proteins which is a major role to ensure functional assembly. However, when the temperature is reduced, these proteins act mainly on the regulation of protein synthesis [15]. Two main chaperones associated with HSP90: DnaK and GroEL. DnaK (HSP70) chaperone has been found as a HSP90-interacting protein. GroEL (HSP60) chaperone is essential for growth under many environmental conditions [16–18].
DnaK and GroEL: Major HSPs (DnaK, GroEL) are molecular chaperones that assist in correct folding and assembly of proteins and are involved in diverse cellular processes including DNA replication, UV mutagenesis, bacterial growth, RNA transcription and flagella synthesis [19,20]. GroEL together with GroES translocate protein across membrane barriers and possibility secretion. Functions of these HSPs are to prevent protein denaturation and to reactivate partially denatured proteins. The chaperone DnaK is an enzyme that uses ATP and ADP released by N-terminal, ATP catalysis and release of unfolded proteins by a C-terminal domain. DnaK can bind to nascent polypeptide chains and assistance in refolding protein and degradation [21].
Regulation of HSP: Regulation of HSP is based on alternative sigma factors that direct RNA polymerase to specific promoters that differ from the housekeeping promoters. Two major sigma factors have been described: sigma-32 and sigma-E (σE) [22]. The sigma-32 factor, encoded by the rpoH gene and regulated post transcriptionally and involves protein stability and translation efficiency. Under normal conditions, the amount of active sigma-32 is kept low [23]. Chaperones inhibit σ32 activity by sequestering σ32 away from RNA-polymerase. This is accomplished by low translation rate of its mRNA which results from the sequestration of the Shine-Dalgarno sequence and the start codon within the secondary structure of the rpoH mRNA [23]. After a precipitate increase in temperature, the secondary structure is removed cause enhanced translation [24] [Table/Fig-2].
Translational induction is due to temperature melting of rpoH mRNA secondary structure resulting to produce active σ32.
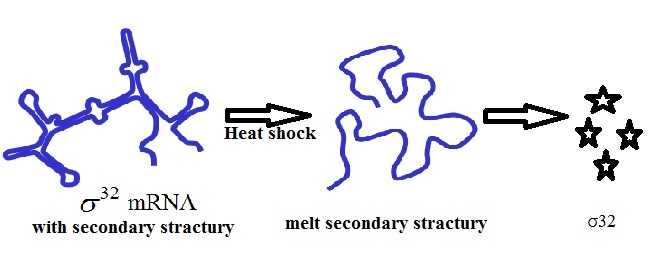
First, a temperature upshifts from 300 to 390C or higher results in the increased translation of rpoH gene [25]. mRNA sites within the five ‘region of rpoH gene form temperature-sensitive secondary structures that detach the ribosome-binding site but at higher temperatures, these secondary structures deliquesce, thereby enabling more efficient translation of the rpoH message [25]. Most HSPs are synthesized at low levels under normal conditions but are induced rapidly and transiently upon exposure to high temperature [26].
Clinical effect: Heat shock protein60 found in both eukaryotic and prokaryotic cell with high sequences similarity [27]. HSP can interact with immune cell and produce antibody response. This effect seen in different bacterial cells such Helicobacter pylari and Mycobacterium leprae [28,29]. One of major heat shock protein an H.pylory is HSP60 that anti H.pylori HSP60 antibody against it found in most of patients. This HSP60 can induce cytokine such a IL-8, IL-1β and TNF-α [30,31] and interact with immune system that suggested to play major role in pathogenesis of gastric cancer. HSP 60 expressed on the surface of bacterial cell and act as adhesion to host cell [32]. High titers of antibody against HSP60 were found with bad diagnosis led to gastric cancer. This effect related to secretion of TNF-α and IL-8 [33]. One of hypothesis for pathology of disease is molecular mimicry between bacterial HSP60 and human HSP60 [34]. In the other hand O2 radical, outer membrane vesicle and pH change can damage the human cell and induce immune response [35].
Discussion
Heat Shock Proteins (HSP) are a group of proteins that is induced by elevated temperature. The major members of this group are a class of functionally related proteins involved in the folding and refolding of other proteins. The expression of HSP increased when cells are exposed to elevated temperatures [36]. Several chaperones, including HSP70 and HSP90, are required for the folding and regulation of a variety of proteins [37]. Several HSP have chaperones activity for other proteins. If the protein aggregation is low, chaperon is at work, however in high protein aggregation proteases lysis the protein into amino acid [Table/Fig-3].
Inactive, non-native or unstable proteins are recognized by either proteases or a chaperone complex which leads to degradation of the proteins to smaller peptides or formation of native proteins.
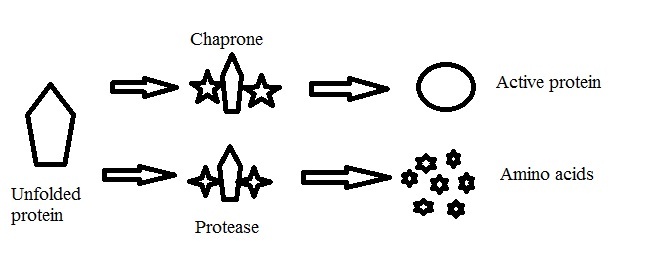
HSPs plays major role in protein interactions such as folding and supporting the establishment of suitable protein compound and prevention of unwanted protein aggregation [38,39].
Conclusion
Bacterial cells which produce HSPs can grow in more challenging temperature. Accelerated temperature in bacterial cell environment leads to damage natural protein, HSPs with refolding or degradation effect can save the bacterial cell. This effect helps the bacterial cell to survive in displacement from environment to body. In the other hands bacterial HSP with similarity to human HSP can interact with immune cells and cause autoimmune response leading to cancer such gastric cancer or leprosy lesion in leprosy disease.
[1]. Bohne J, Sokolovic Z, Goebel W, Transcriptional regulation of prfA and PrfA-regulated virulence genes in Listeria monocytogenes Mol Microbiol 1994 11(6):1141-50. [Google Scholar]
[2]. Straus DB, Walter WA, Gross CA, The heat shock response of E. coli is regulated by changes in the concentration of [sigma]32 Nature 1987 329(6137):348-51. [Google Scholar]
[3]. Street TO, Elucidating the mechanism of substrate recognition by the bacterial HSP90 molecular chaperone Journal of Molecular Biology 2014 426(12):2393-404. [Google Scholar]
[4]. Finka A, Goloubinoff P, Proteomic data from human cell cultures refine mechanisms of chaperone-mediated protein homeostasis Cell Stress Chaperones 2013 18(5):591-605. [Google Scholar]
[5]. Nakamoto H, Physical interaction between bacterial heat shock protein (HSP) 90 and HSP70 chaperones mediates their cooperative action to refold denatured proteins J Biol Chem 2014 289(9):6110-19. [Google Scholar]
[6]. Krukenberg KA, Conformational dynamics of the molecular chaperone HSP90 Q Rev Biophys 2011 44(2):229-55. [Google Scholar]
[7]. Taipale M, Jarosz DF, Lindquist S, HSP90 at the hub of protein homeostasis: emerging mechanistic insights Nat Rev Mol Cell Biol 2010 11(7):515-28. [Google Scholar]
[8]. Mayer MP, Gymnastics of molecular chaperones Mol Cell 2010 39(3):321-31. [Google Scholar]
[9]. Wayne N, Mishra P, Bolon DN, HSP90 and client protein maturation Methods Mol Biol 2011 787:33-44. [Google Scholar]
[10]. Sato T, HtpG, the prokaryotic homologue of HSP90, stabilizes a phycobilisome protein in the cyanobacterium Synechococcus elongatus PCC 7942 Molecular Microbiology 2010 76(3):576-89. [Google Scholar]
[11]. Gragerov AI, Protein aggregation and inclusion body formation in Escherichia coli rpoH mutant defective in heat shock protein induction FEBS Lett 1991 291(2):222-24. [Google Scholar]
[12]. Thomas JG, Baneyx F, ClpB and HtpG facilitate de novo protein folding in stressed Escherichia coli cells Molecular Microbiology 2000 36(6):1360-70. [Google Scholar]
[13]. Buchner J, Bacterial HSP90 – desperately seeking clients Molecular Microbiology 2010 76(3):540-44. [Google Scholar]
[14]. Versteeg S, Mogk A, Schumann W, The Bacillus subtilis htpG gene is not involved in thermal stress management Mol Gen Genet 1999 261(3):582-88. [Google Scholar]
[15]. Georlette D, Some like it cold: biocatalysis at low temperatures FEMS Microbiol Rev 2004 28(1):25-42. [Google Scholar]
[16]. Houry WA, Identification of in vivo substrates of the chaperonin GroEL Nature 1999 402(6758):147-54. [Google Scholar]
[17]. Wong P, Houry WA, Chaperone networks in bacteria: analysis of protein homeostasis in minimal cells J Struct Biol 2004 146(1-2):79-89. [Google Scholar]
[18]. Wand-Wurttenberger A, Surface expression by mononuclear phagocytes of an epitope shared with mycobacterial heat shock protein 60 Eur J Immunol 1991 21(4):1089-92. [Google Scholar]
[19]. Yura T, Nagai H, Mori H, Regulation of the heat-shock response in bacteria Annu Rev Microbiol 1993 47:321-50. [Google Scholar]
[20]. Fayet O, Ziegelhoffer T, Georgopoulos C, The groES and groEL heat shock gene products of Escherichia coli are essential for bacterial growth at all temperatures Journal of Bacteriology 1989 171(3):1379-85. [Google Scholar]
[21]. Stevens SY, The solution structure of the bacterial HSP70 chaperone protein domain DnaK(393-507) in complex with the peptide NRLLLTG Protein Sci 2003 12(11):2588-96. [Google Scholar]
[22]. Model P, Jovanovic G, Dworkin J, The Escherichia coli phage-shock-protein (psp) operon Mol Microbiol 1997 24(2):255-61. [Google Scholar]
[23]. Wolfgang S, CIRCE HAIR and ROSE Regulation of the bacterial heat shock response Biology International 2001 40:3-5. [Google Scholar]
[24]. Morita MT, Translational induction of heat shock transcription factor σ (32): evidence for a built-in RNA thermosensor Genes & Development 1999 13(6):655-65. [Google Scholar]
[25]. Moat AG, Foster JW, Spector MP, Microbial stress responses, in Microbial Physiology 2002 Wiley-Liss, Inc [Google Scholar]
[26]. Abee T, Wouters JA, Microbial stress response in minimal processing Int J Food Microbiol 1999 50(1-2):65-91. [Google Scholar]
[27]. Carlsen TG, A role for anti-HSP60 antibodies in arthritis : a critical review OA Arthritis 2013 :1 [Google Scholar]
[28]. Liao KW, Antibodies against Helicobacter pylori heat shock protein 60 aggravate HSP60-mediated proinflammatory responses Cytokine 2011 55(2):174-80. [Google Scholar]
[29]. Singh I, Molecular mimicry between HSP 65 of Mycobacterium leprae and cytokeratin 10 of the host keratin; role in pathogenesis of leprosy Cell Immunol 2012 278(1-2):63-75. [Google Scholar]
[30]. Demaria S, Cancer and Inflammation: Promise for Biological Therapy Journal of immunotherapy. (Hagerstown, Md. : 1997) 2010 33(4):335-51. [Google Scholar]
[31]. Lin CS, A potential role for Helicobacter pylori heat shock protein 60 in gastric tumorigenesis Biochem Biophys Res Commun 2010 392(2):183-89. [Google Scholar]
[32]. Yamaguchi H, Flow cytometric analysis of the heat shock protein 60 expressed on the cell surface of Helicobacter pylori J Med Microbiol 1996 45(4):270-77. [Google Scholar]
[33]. Tanaka A, Helicobacter pylori heat shock protein 60 antibodies are associated with gastric cancer Pathol Res Pract 2009 205(10):690-94. [Google Scholar]
[34]. Lahesmaa R, Skurnik M, Toivanen P, Molecular mimicry: any role in the pathogenesis of spondyloarthropathies? Immunol Res 1993 12(2):193-208. [Google Scholar]
[35]. Nasser A, Zamirnasta M, Jalilian FA, Bacterial Nanoparticle As a Vaccine for meningococcal disease Biosciences biotechnology research asia 2014 11(2):449-53. [Google Scholar]
[36]. De Maio A, Heat shock proteins: facts, thoughts, and dreams Shock 1999 11(1):1-12. [Google Scholar]
[37]. Young JC, Moarefi I, Hartl FU, HSP90: a specialized but essential protein-folding tool J Cell Biol 2001 154(2):267-73. [Google Scholar]
[38]. Walter S, Buchner J, Molecular chaperones—cellular machines for protein folding Angew Chem Int Ed Engl 2002 41(7):1098-113. [Google Scholar]
[39]. Borges JC, Ramos CH, Protein folding assisted by chaperones Protein Pept Lett 2005 12(3):257-61. [Google Scholar]