Poor posture is a common problem in children and adolescents and has a prevalence of 22-65% [1–3]. The quantification of body posture in the sagittal plane, which is important for clinical diagnosis, can be measured by means of angle and distance parameters [4–7]. In posture assessment in clinical daily practice, it is important to define which specific posture is being assessed by the researcher.
Both proprio-receptive and also visual and vestibular information are involved in posture control [8]. Thus, the quality of posture being determined clinically is dependent on the influence of receptors, of the central nervous processing system, and of the muscles. Differences between passive and active postures and between postures with open or closed eyes could be relevant for the assessment of poor posture and at the same time lead to new starting points for therapeutic interventions.
The first objective of the present trial was to investigate whether the geometric parameters describing posture in children and adolescents in the sagittal plane change when the subjects try to correct their posture by activating muscles.
The study also investigates whether changes in the posture parameters occur when an actively corrected posture is to be maintained and the eyes are closed.
Materials and Methods
Two hundred sixteen male children and adolescents took part in the study (12.4 ± 2.5 years, 47.5 ± 15.8 kg, 158.3± 17.5 cm). The subjects were recruited by means of newspaper ads within the framework of an interdisciplinary university research project that explores weak posture in children (www.kidcheck.de). Parents who thought that their children suffered from weak posture and who wanted their children to be examined attended an examination appointment at our posture analysis laboratory (Saarland University, Germany). In the years 2012 – 2014 a total of 348 children and adolescents between 7.0 and 17.6 years participated in the project. For this study, posture data of all 216 male participants was analysed post-hoc.
The participants and their parents or guardians were informed prior to the trial, in accordance with the requirements of the Declaration of Helsinki, on the trial objective and trial procedure and gave their written informed consent.
All the posture parameters were calculated based on posture images of the sagittal plane. This is a practical, reliable and valid examination method [9,10]. For this, the test subjects had to undress and high-contrast marker points or marker spheres (12 mm in diameter) were stuck onto the anatomical reference points indicated in [Table/Fig-1]. The subjects were placed sideways in front of a measuring wall looking straight ahead. A total of three posture images were taken using a high-resolution camera (Olympus SP510UZ, resolution 2304 x 3072 pixels), which was placed on a tripod at hip-height in each case. The measuring wall was used to calibrate the camera image on a horizontal plane.
Anatomical landmarks that are used in the study: 1 – skull, 2 – auditory canal, 3 – acromion, 4 – distal sternum, 5 – maximum of thoracic kyphosis, 6 – SIAS, 7 – maximum of lumbar lordosis, 8 – trochanter major, 9 – malleolus lateralis, 10 – sole of foot
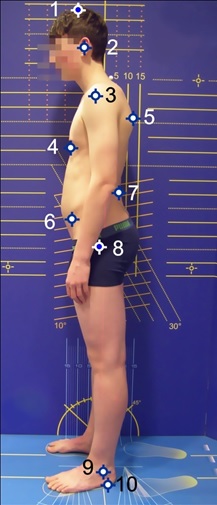
The subjects were asked to take the following postures one after another [Table/Fig-2]:
Posture positions that were analysed in the study (boy, 15 y): a. passive habitual posture, subject standing relaxed; hip, shoulder and ear are in front of the plumb line (yellow); b. actively erected posture, eyes open; note that all horizontal marker distances are reduced; c. actively erected posture, eyes closed; hip, shoulder and ear distances increase again (for description of posture positions, see text).
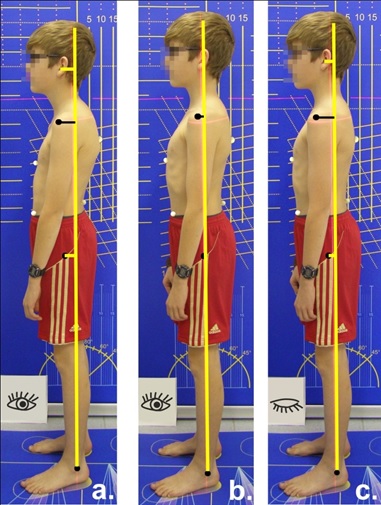
Relaxed habitual posture (passive posture PA): The subjects were told to stand in a relaxed way, facing forwards with their arms hanging down at the sides of their bodies.
Actively erect posture with eyes open (Aeo): The subjects were told to try to erect their posture by actively tensing their muscles. To give them a model they were told: “Imagine that you are a puppet and are being pulled up from your head by means of a string.” The subjects were told to continue to breathe normally.
Actively erect posture with eyes closed (Aec): The subjects were told to maintain the actively erect posture, continue to breathe normally and to close their eyes. After 60 seconds the third posture image was taken.
The image data was transferred to a computer. With the aid of the posture analysis programme Corpus concepts (AFG, Idar-Oberstein, Germany), the following posture parameters were determined [Table/Fig-3]:
Posture parameters in the sagittal plane: A) ear plumb line distance (dE), shoulder plumb line distance (dS), hip plumb line distance (dH); b) trunk incline (TI), upper body tilt (BT); c) plumb line distances that are used to calculate the posture index (see text).
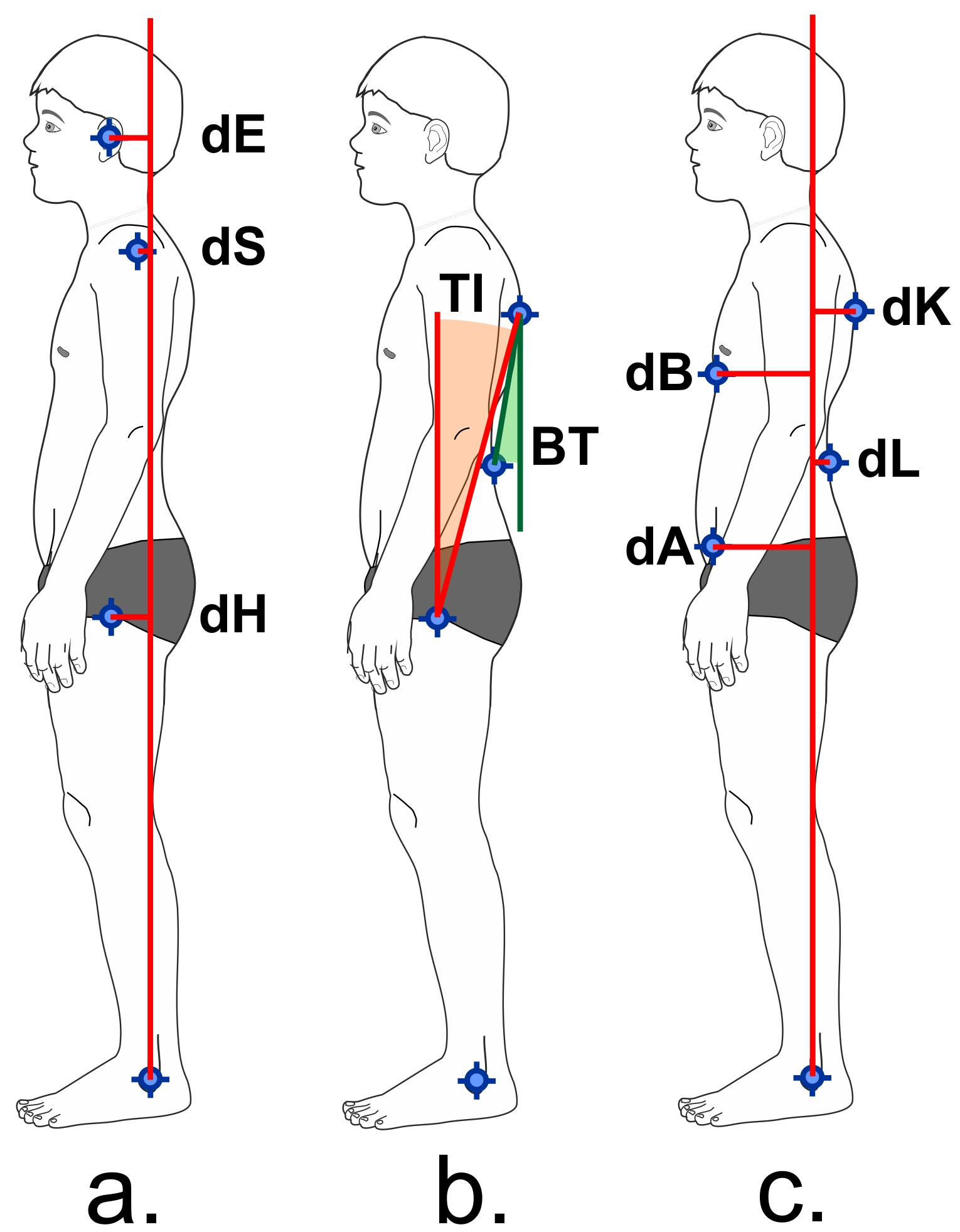
- Horizontal distances between the marker points of the hip, shoulder and ear and the perpendicular to the ankle joint (perpendicular distance).
- Upper body tilt (angle of the junction of marker 5 and 7 from the vertical).
- Trunk incline (angle of the junction of marker 5 and 8 from the vertical).
- Posture index.
The posture index according to Fröhner [11] is a parameter which gives a summarised assessment of trunk alignment, by calculating the distances of four body points to the perpendicular through the ankle joint. The caudal tip of the sternum, the point of maximum lumbar lordosis, the point of maximum thoracic kyphosis and the Spina iliaca anterior superior (SIAS) act as reference points. The posture index is calculated using (dK+dA) / (dB+dL), where dK = horizontal distance between thoracal kyphosis and plumb line, dA = horizontal distance between SIAS and plumb line, dB = horizontal distance between breastbone and plumb line, dL = horizontal distance between lumbar lordosis and plumb line. A stable posture is indicated by values between 1.0 and 1.3 [11].
Thehorizontal perpendicular distances were scaled according to the subject’s body height (vertical distance between marker 1 and 10 in [Table/Fig-1].
Statistical Analysis
The statistical parameters were calculated using the software WinSTAT for Windows Excel. Differences between passive habitual posture (PA), active with open eyes (Aeo) and active with closed eyes (Aec) were tested for significance with the t-Test or Wilcoxon-Test after testing for normal distribution of differences. The p-value was set at 0.05. After Bonferroni correction of the 12 difference hypotheses the significance level was 0.004.
Results
When changing from passive habitual (PA) to active posture (Aeo) all examined parameters changed significantly [Table/Fig-4]. When standing in an activated posture all perpendicular distances decreased, as did the trunk incline of the torso and upper body [Table/Fig-5]. This indicates that the subjects reduced the forward flexion of their body. The biggest changes of all perpendicular distances were observed for the ear marker (-4.44 ± 2.43 % height for PA versus -3.04 ± 2.60 % height for Aeo). As body tilt (BT) decreased from 13.91° ± 4.88° to 12.85° ± 5.0°, the lumbar lordosis was reduced when posture was activated. Beyond that, the significantly reduced posture index (PI) indicates a straighter position of the upper body in active posture.
Posture parameters in the three test situations (mean ±standard deviation), N=216.
Postureposition | passive | activeeyes open | activeeyesclosed | differences |
---|
PA | Aeo | Aec |
---|
mean stdev | mean stdev | mean stdev | PA - Aeo | Aeo - Aec | PA - Aec |
---|
dHip(% height) | -2.8±1.82 | -1.94±1.79 | -2.38±1.75 | * w | * w | * w |
dShoulder(% height) | -3.18±2.39 | -2.27±2.46 | -3.23±2.74 | * w | * w | 0.699 w |
dEar[% height] | -4.44±2.43 | -3.04±2.60 | -4.00±1.74 | * w | * w | 0.008 w |
TI Trunk incline (°) | 16.8±4.10 | 15.01±3.83 | 15.05±4.15 | * t | 0.468 t | * t |
BT Body tilt(°) | 13.91±4.88 | 12.85±5.00 | 12.80±5.66 | * t | 0.922 t | * t |
PI Posture index (-) | 1.12±0.20 | 1.02±0.21 | 1.03±0.22 | * t | 0.059 t | * t |
Negative values for perpendicular distances mean that the marker point is located in front of the plumb line. The three columns to the right indicate significant differences (p-values) between the three posture positions (PA – passive posture, Aeo – active posture with open eyes, Aec – active posture with closed eyes): * p<0.004; t: t-Test, w: Wilcoxon-Test. dHip, dShoulder, dEar are the horizontal distances between the marker points and the plumb line through the ankle marker (other parameters see text).
Box plots of the calculated posture parameters for the three test situations. The boxes span the interquartile range (25 and 75 percentile); whiskers mark the 5 and 95 percentile. The horizontal bar marks the median, and black squares mark outliers. Red dotted lines link the medians.
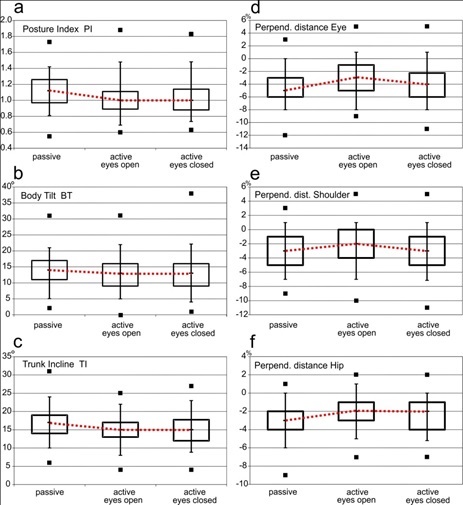
Upon closing the eyes, all the perpendicular distances (dHip, dShoulder, dEar) again increased significantly [Table/Fig-4], column Aeo-Aec). While the forward flexion of the body was reduced when posture was voluntary activated, this correction disappeared after the eyes were closed [Table/Fig-5 d-f]. The perpendicular shoulder distance (dShoulder, -3.23 ± 2.74 % height) and ear distance (dEar, -4.00 ± 1.74% height) almost reached the base level of passive habitual posture [Table/Fig-5 d+e].
The parameters describing the alignment of the trunk segments in relation to one another (posture index PI, body tilt BT, trunk incline TI) remained the same in the two actively controlled posture positions [Table/Fig-5 a-c].
Discussion
Poor posture in children and adolescents is suggested to be responsible for back pain both in young and adult age [4]. Therefore, proper posture assessment is crucial to initiate appropriate clinical intervention [9]. However, the question arises which posture positions should ideally be measured. In our study, both passive and active posture positions of children and adolescents were examined and compared. We were able to show that significant differences exist between active and passive posture positions, and that changes in posture occur when the eyes were closed. Therefore, these three posture positions should be compared in clinical posture assessment.
Posture control as a regulatory process: In order to understand the importance of distinguishing these posture positions, it is helpful to realise how the central nervous system (CNS) regulates posture and what measurable changes in trunk alignment occur during that process.
Posture is a result of a complex regulatory process, in which active structures (muscles) and passive structures (ligaments, tendons, joints) interact [12, 13]. In the relaxed passive posture position most of our subjects showed a slight tendency to tilt forwards in relation to the plumb line to the ankle, which is reflected geometrically in the increase in the perpendicular distances from caudal to cranial. This is consistent with other studies which show that the regulation of the centre of mass is primarily carried out through the ankle joint [14,15].
When moving from an habitual passive to an active posture, it is well known that a measurable displacement of the trunk segments in relation to each other takes place [16], caused by increased muscle activity [17]. This process was assessed in our study by comparing the changes in the sagittal posture parameters.
Posture control without the visual system: The changes that occurred when the eyes were closed are interesting. An increased forward flexion of the body could be observed. The alignment of the trunk segments to one another remained unchanged, even with the eyes closed, as shown by the constant posture index, trunk incline and upper body tilt. This initially seems to be contradictory but can be explained by the posture control mechanisms in the CNS. The main receptor systems involved in posture control are the proprio-receptive information systems (muscle, tendon and joint receptors [18], mechano-receptive information systems (pressure sensors in the skin) and vestibular and visual information systems [19]. During the process of sensory integration the CNS uses this information from receptors to perceive the current body posture and to generate muscle reactions if a posture deviation is noted [20]. When the eyes are closed, the visual receptor system (the ‘optical analyser’) is turned off from a neurophysiological point of view. With the loss of visual information the CNS has to rely on the remaining receptor information systems to guarantee the upright stance of the body and the stabilisation of the trunk segments in relation to one another [21].
A forward displacement of the body, such as that observed when the subject’s eyes were closed, at the same time generates a greater mechanical pressure on the forefoot. This may be interpreted as a strategy to provoke a greater level of receptor information from the mechano-receptors, in order to allow a more effective perception of the position of the body in the sagittal plane, despite the eyes being closed.
These results are consistent with the research done by Collins et al., who hypothesized that switching off the visual sense increases the level of muscular activity across the joints of the lower limb, and thus increases the stiffness of the musculoskeletal system [22]. This is medically relevant, since Dolphens et al., were able to show that the forward displacement of the body is an important parameter that is linked to the appearance of complaints [5]. The quality of control of the forward displacement of the body is largely dependent on vision. Peterka could show that during quiet stance with eyes open normal subjects derive about one-third of their orientation information from vision [8].
Relevance for clinical practice: In terms of medical posture assessment it can be deduced that the observed change of the active posture once the eyes are closed allows us to draw significant conclusions regarding the role of the optical analyser in the area of posture control [23]. The observed worsening in posture which occurs when the eyes are closed could thus be interpreted to quantify the deficit of the proprio-receptive awareness of the body position on the sagittal plane. Therefore, a comparison between the active posture with open eyes and closed eyes can provide useful information for the physical therapist to decide which kind of exercise a child should perform to improve its poor posture, and its proprio-receptive awareness of the body position. If the posture worsens when the eyes are closed, an exercise program focusing on body awareness could be helpful.
By comparing the posture parameters in the three posture positions examined in this study, physicians are able to make differential diagnostic statements 1. About the ability of intentionally activating the muscles relevant to posture, 2. About the precision of posture control, and 3. About the significance of the visual system for posture control. Therapeutic intervention aimed at treating poor posture can be established using these results.
Our results may be summarized as follows
The significant change in all the parameters recorded in the sagittal plane indicates that these are suitable for assessing body posture and for measuring differences between passive and active posture of children and adolescents.
For medical diagnosis this also implies that there is a requirement to always examine both passive and active posture positions and to compare the two.
Posture transition when the eyes are closed provides important information about the performance of proprio-receptive information processing (body awareness) of the subject examined.
Conclusion
In clinical assessment of posture of children and adolescents, habitual passive posture and active posture with open and closed eyes are recommended to be compared by measuring sagittal posture parameters. The comparison of these parameters enables conclu-sions about the quality of posture control and the proprio-receptive awareness of posture.
Negative values for perpendicular distances mean that the marker point is located in front of the plumb line. The three columns to the right indicate significant differences (p-values) between the three posture positions (PA – passive posture, Aeo – active posture with open eyes, Aec – active posture with closed eyes): * p<0.004; t: t-Test, w: Wilcoxon-Test. dHip, dShoulder, dEar are the horizontal distances between the marker points and the plumb line through the ankle marker (other parameters see text).