Traditionally, 1.8-2 Gy (Gray) of radiation delivered daily for five days per week is regarded as the conventional treatment scheme for carcinoma cervix in humans. The cellular effect of low dose radiation with dose fractions less than 1 Gy, have been extensively studied previously [1–4]. Studies by Joiner et al., in early 1990’s on the effect of radiation doses below 1 Gy found that at very low doses, many prokaryotic and eukaryotic cells exhibit extreme hypersensitivity to radiation leading to increased cell death [3,5,6]. Subsequent experiments showed that, at very low doses (less than 30 cGy), several human cancer cell lines (for example, H29 Colorectal cell line, Be11, MeWo and U1 melanoma cell line, RT12 and UM-UC-3 bladder carcinoma, DU145 prostate carcinoma, A549 lung adenocarcinoma, T98G glioblastoma cell line) exhibit hypersensitivity to radiation leading to increased cell kill [4]. At a dose range between 30-80c Gy, an increased resistance per unit dose of radiation was observed, due to the activation of early G2-M checkpoint causing G2 phase cellular arrest. Thus, the part of the cell survival curve below 1 Gy is divided into a hyper-radiosensitive phase (HRS) and an induced radio-resistance phase (IRR). The aim of this study was to study the radiobiological response of cervical cancer cell line in low dose region below 1 Gy and explore the existence of HRS and IRR. We have selected HeLa cell line as it is one of the most well-known cell lines of cervical cancer, used in many radiobiological studies.
Materials and Methods
Following materials and reagents were used as received, HeLa cells (obtained from Kings Institute of Preventive Medicine Chennai), Phosphate buffered saline (PBS), Eagle’s minimum essential medium (EMEM), Fetal Bovine Serum (10% FBS), antibiotics (streptomycin, penicillin and amphotericin), Trypsin, EDTA, glutaraldehyde, crystal violet.
Cell Culture
HeLa cell line was cultured in Eagle’s Minimum Essential Medium (E-MEM) supplemented with fetal bovine serum (FBS), antibiotics (penicillin, streptomycin), and amphotericin B. Cell line was maintained in CO2 incubator at 37°C, 5% CO2 concentration and 90% humidity (Nuaire CO2 incubator). Medium was changed every 72 hours to maintain viability and sub cultured to maintain continuity of the cell line.
Clonogenic Assay
For clonogenic assay, cell suspensions of various concentrations were plated. For this purpose ideal seeding concentration was determined by carrying out multiple initial experiments so as to prevent overlap of cells. For the experimental study, cells were plated in 24 well plates (for Cobalt 60 radiation) and 96 well plates (for radiation in linear accelerator) with different cell concentrations as described by Guirado et al., [7]. For radiation in Co60, γ rays, each well was seeded with 250 or 500 cells per well and for 6 MV and 15 MV radiation, each well was plated with 25 cells after standardization. The well chambers were filled with medium and incubated at 37°C. Cell count was done by haemocytometer with trypan blue exclusion technique. As HeLa cells are adherent cells they stick to the bottom of the plate and forming monolayer.
Irradiation Technique
After 24 hours of incubation at 37°C, allowing sufficient time for the cells to completely adhere to the surface, the cells were treated with radiation in megavoltage radiotherapy machines, Telecobalt (Theratron, 780C, Theratronics Ltd) and linear accelerator (Varian Clinac 2100C/D). Cells were treated in Co60 gamma rays, 6 MV photon (different dose rates 200 MU/min, 300 MU/min), and 15 MV photon energy beam (200 MU/min). Different dose ranges in low dose region (5 cGy to 100 cGy) and beyond, up to 4 Gy was used. Customized phantom was made for cellular radiation using Perspex with modification of the method described by Guirado et al., [7]. The plate was placed in a Perspex slab with an inbuilt hole of the size of the plate. Perspex sheets of 10 cm thickness were kept below the plate to generate maximum backscatter. For radiotherapy, field size was kept as 20 x 20 cm2. Source to surface distance (SSD) was kept at 80 cm for Co-60 beam and 100 cm for 6 MV and 15 MV photons. A build-up of 1.2 cm perspex (equivalent thickness in water for 1.5 cm) was used for 15 MV photon and build up was not used for Co-60 and 6 MV photons as dmax was less than or equal to 1.5cm. The treatment time calculation was done for SSD technique for a field size of 20 x20 cm2 at the depth of 1.5 cm for Co-60 and at dmax for other photon energies. Calculation was done using available treatment planning system (TPS). The radiation technique is depicted in [Table/Fig-1]. The control group in each experiment was subjected to same procedure expected actual radiation (sham treatment done).
Experimental set up of cellular radiation; culture plates placed in perspex phantom
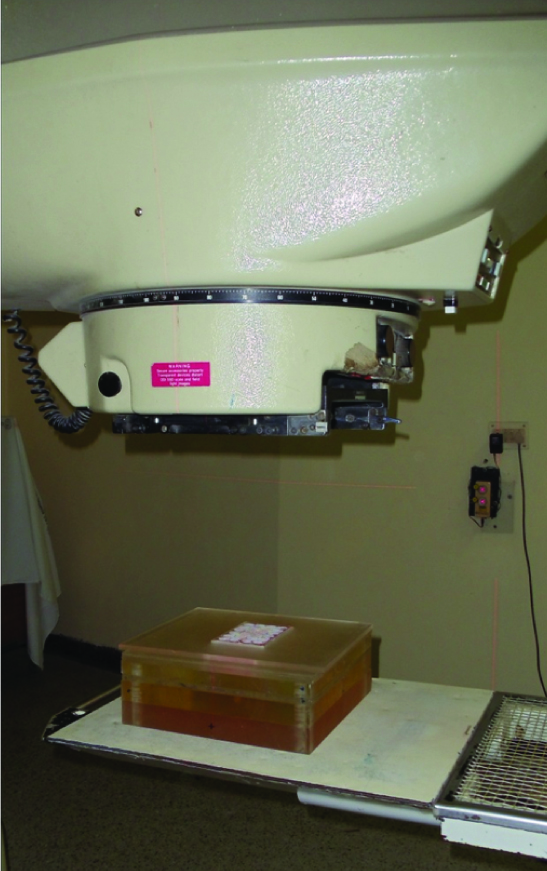
Clonogenic Assay
After radiation, cells were incubated for 7 days at 37°C with 5% CO2 concentration and 90% humidity. Medium was changed every 72 hours. Crystal violet (3%) staining was done after 7 days after fixation with 6% glutaraldehyde as described by Franken et al., [8]. Colonies were observed under microscope and viable colonies were counted (colonies with cells more than 50). In unirradiated plates (control) percentage of cells seeded that forms viable colonies provide plating efficiency (PE). Surviving fraction was calculated using the following formula [8].
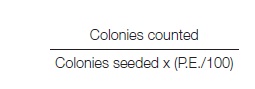
Statistical Analysis and Radiobiological Modelling
Radiobiological survival curve was obtained by plotting dose on a linear scale (x axis) and surviving fraction on a logarithmic scale (y axis). The low dose cellular response was modelled by Joiner’s induced repair model as shown below where αs and αr are model parameters (slope) and Dc represents the transition dose from HRS to IRR [4].
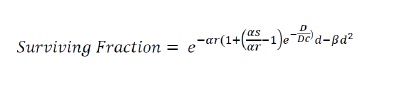
Results
HeLa cell line has ability to form colonies from single clonogenic cells. HeLa cells assume a highly stretched, amoeboid form, diffusely spread when grown with FBS.
Morphological Changes After Radiation
After radiation, cells which loose clonogenic potential were seen suffer three fates (i) they may undergo few mitotic divisions followed by cell death (mitotic catastrophe). During this process they may form micro colonies where the cell number never exceeds 50 (abortive colonies) even after long period of incubation. (ii) They may remain as single cells (iii) cells may undergo complete cell lysis where due to complete dissolution of cellular components that cannot be visualized after staining. After exposure to radiation, some of the single cells may continue to grow in size (even though they cannot divide due to loss of mitotic potential) and forms giant cells. We observed formation of elliptical or elongated giant cells adherent to the surface with enlarged nucleus with occasional double nuclei. The frequency of giant cell formation increases with increased dose of radiation [Table/Fig-2].
(a) HeLa cell colony formation (control plate); (b) Abortive colonies in radiated plate; (c) Colonies with giant cell formation
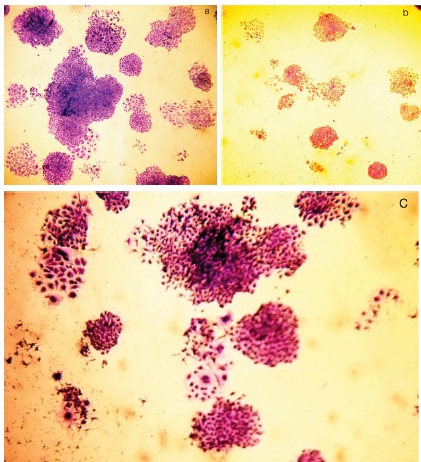
Response to Low Dose Radiation (below 1 Gy)
Exposure to Cobalt 60 radiation
The cell survival curve after radiation to cobalt 60 is shown in [Table/Fig-3]. The points are fitted in induced repair model. As can be seen from the curve the extrapolation of the quadratic model fails to fit the points below the dose range of 1 Gy. As shown in [Table/Fig-3] (inset) the curve below 1 Gy shows two distinct parts (initial hypersensitive area followed by an area of induced radioresistance). There is a distinct transition point between these two areas which was 28 cGy (Dc). The values of αr and αs were 1.55 and 11.01 respectively. The fact that αs > αr, with non zeo value of Dc supports the existence of HRS. The data points were well fitted in an induced repair model (goodness of fit, R2=0.96).
Low dose radiation response with Cobalt 60 radiation (n=4)
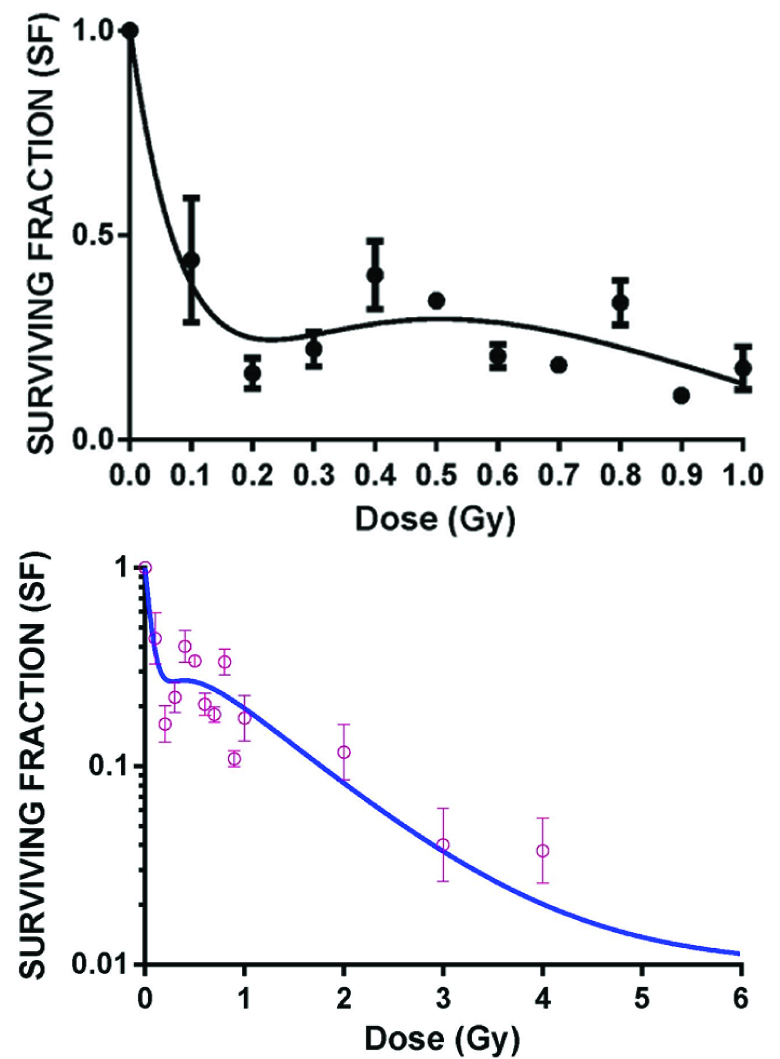
Exposure to 6 MV and 15 MV photon beam
The survival curve of HeLa cells on exposure to 6 MV and 15 MV photon beam energies are shown in [Table/Fig-4,5]. [Table/Fig-4] shows survival curve in low dose region on exposure to 6 MV photon beams of dose rate 200 MU/min and 300 MU/min. The curve clearly shows the initial part of hyper radiosensitivity followed by induced radioresistance with the point of deflection (Dc at 25 cGy). An inverse dose rate effect was observed below 1 Gy which did not persist beyond 1 Gy (data not shown). With exposure to 15 MV energy beam (at dose rate of 200 MU/min) HRS and IRR phenomenon was found to persist though the transition point Dc shifted to a dose of 40 cGy [Table/Fig-5].
Low dose radiation response with 6 MV photon (n=3)
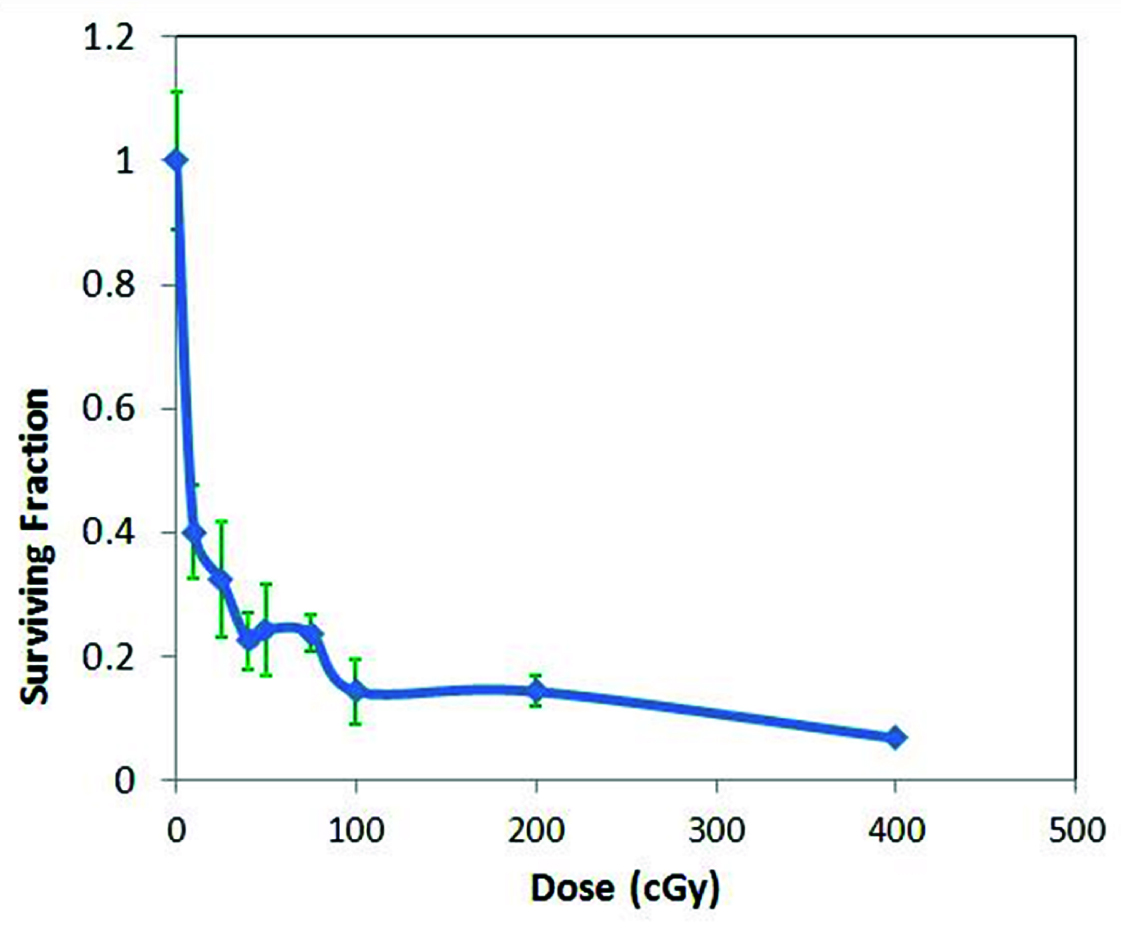
Low dose radiation response to 15 MV photon (n=3)
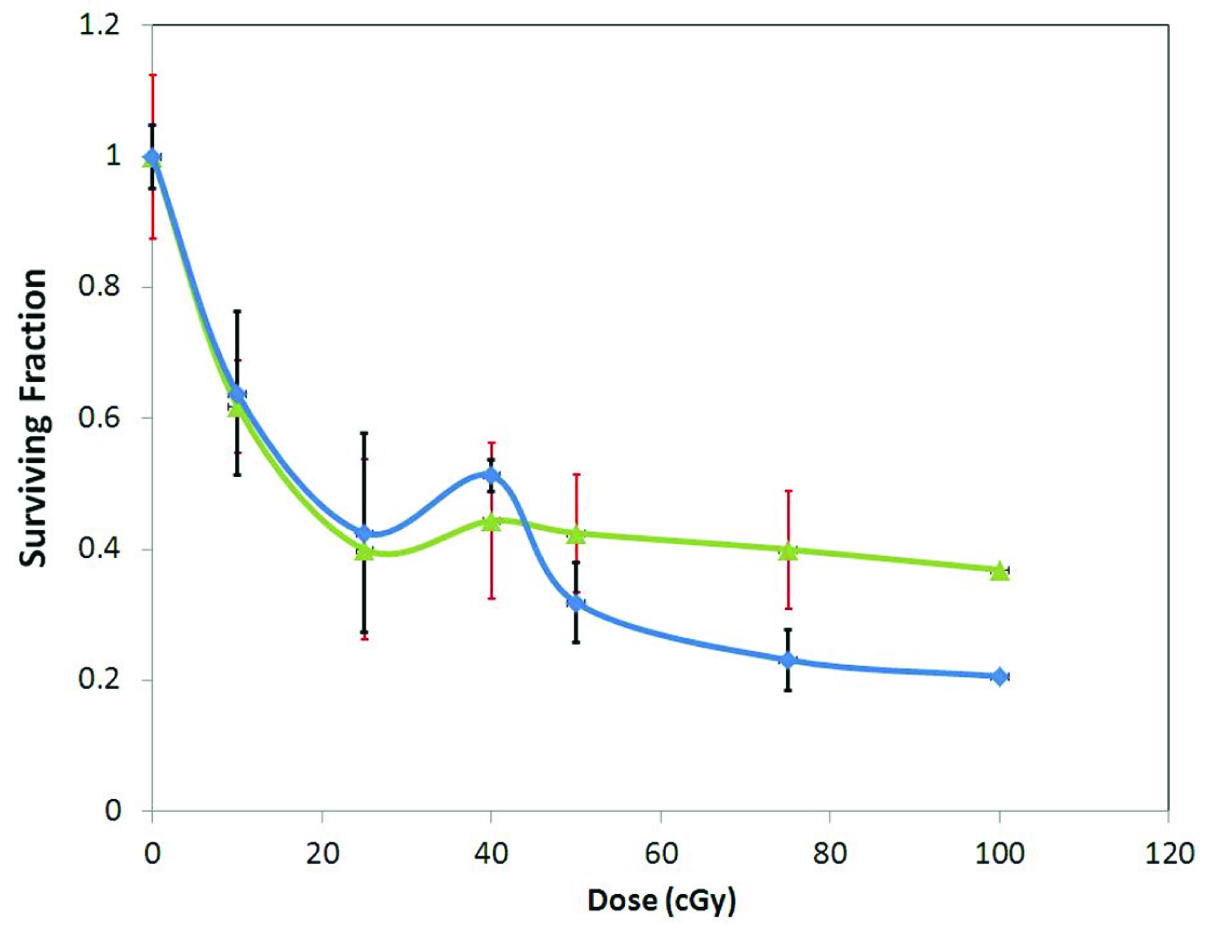
Discussion
The study clearly shows the existence of the phenomenon of low dose hypersensitivity and induced radioresistance in HeLa cell. HeLa cell line demonstrates low dose hypersensitivity. Previously, The HRS/IRR response has been studied in SiHa cell line that showed no evidence of HRS [9].The transition dose was found to be 28 cGy for Co60 beam, 25 cGy for 6 MV and 40 cGy for 15 MV photon. The cellular effects of ionizing radiation were described initially by Puck and Marcus [10–12]. They first observed the formation of giant cells in HeLa cell colonies following exposure to radiation. These cells where characterized by high metabolic activity and can be readily stained by vital stains. The DNA is the principal cellular target of ionizing radiation and the molecular mechanisms involved in the effect of radiation on the cell is complex. Series of molecular events take place after irradiation to the cell [13–15]. As a response of radiation induced damage, Ionizing Radiation Induced Factor (IRIF), a histone (H2AX) based protein complex is formed at the damaged site of the DNA. This leads to activation of three signal transduction pathways mediated by Ataxia-telangiectasia-mutated (ATM) protein, Ku70- Ku 80 and Ataxia Telangiectasia related (ATR) protein. These three signal pathways ultimately results in activation of one or more of the three effect or mechanisms like- a) cell death (by apoptosis); b) activation of cell cycle checkpoints leading to delay in cell division; and c) DNA repair pathway. On exposure to ionizing radiation, the ser 1981 residue of the ATM protein is activated [1]. This molecular change in ATM protein is threshold dose dependent and various studies have shown distinct threshold response [12]. In addition to ATM protein the trimeric MRN complex (Mre11, RAD50 and NBS1) plays a vital role in radiation response. The phosphorylated ATM interacts with NBS1resulting in cell-cycle arrest and p-53 dependent apoptosis [16].
In the part of the cell survival curve below 1 Gy, the survival fraction has a distinct transition in the region of 0.3-0.4 Gy from hyperradiosensitive phase (HRS) to induced radioresistance (IRR). Studies by Kreuger and Enns et al., have demonstrated the role of apoptosis in HRS [15,17]. It has been demonstrated that the apoptotic response was mediated through the p53- dependent activation of Caspase 3, which forms part of the signaling cascade downstream of ATM activation, and is a key player in HRS [16]. Studies by Enns et al., showed a discernable activation of caspase-3 at the doses that induce HRS for the cell lines with low dose hypersensitivity [17]. This is followed by threshold activated ATM activation leading to cellular arrest in G2 phase (described above) which is responsible for IRR.
The study has significant clinical implications. Since induced radioresistance causes arrest of the cell cycles in G2 phase, chemotherapeutic effect of agents (such as Taxanes, Vinca alkaloids and etoposide) that act principally in the G2 phase can be increased by combining with radiation dose between 40 cGy-100 cGy, in cervical cancer leading to possible increased cell death and arrest of the cell cycle at the G2 phase. This may form the biological basis of the clinical study to investigate the chemopotentiating effect of low dose radiation in cervical cancer.
Limitations of the Study
The study was based on conventional clonogenic assay. Use of flow cytometer, or Dynamic Microscopic Image Processing Scanner (DMIPS) would further the resolution of the study. The effect of low dose hypersensitivity in potentiating the action of cell cycle specific chemotherapy should be investigated.
Conclusion
HeLa cell line demonstrates marked HRS and IRR with distinct transition dose. This may form the biological basis of the clinical study to investigate the chemopotentiating effect of low dose radiation in cervical cancer.