Nanosurface – The Future of Implants
GK Thakral1, Rashmi Thakral2, Neeraj Sharma3, Jyotsana Seth4, Pallavi Vashisht5
1 Professor, Department of Prosthodontics, SDCH, Rishikesh, India.
2 Professor, Department of Periodontology, SDCH, Rishikesh, India.
3 Senior Lecturer, Department of Prosthodontics, SDCH, Rishikesh, India.
4 Senior Lecturer, Department of Prosthodontics, SDCH, Rishikesh, India.
5 Senior Lecturer, Department of Prosthodontics, SDCH, Rishikesh, India.
NAME, ADDRESS, E-MAIL ID OF THE CORRESPONDING AUTHOR: Dr. GK Thakral, Saket, Kinkraig Bridle Path, Kulri, Mussoorie-248179, India.
Phone: +91-98-972-60666,
E-mail: gkthakral@yahoo.com
Nanotechnology is a relatively newer field of science that is finding enormous scope in the dental & medical science. Use of endosseous dental implant surfaces having nano-scale topography is fast becoming part of modern implantology. The purpose of this review is to discuss and understand the role of nanoscale surface modification of titanium materials for the purpose of improving various phases of implantology including osseointegration. Nanotechnology equips bioengineers with newer ways of interacting with relevant biological processes. On the other hand, the field of nanotechnology provides means of understanding and achieving cell specific functions. An understanding of the role of nano-topography leads to the significant osseointegration modulations by nanoscale modification of the implants surface. Use of nanotechnology to modify the topography of titanium endosseous implant can drastically improve cellular and tissue responses that may benefit osseointegration and dental implant procedures.
Dental implants,Osteogenesis,Osseointegration,Topography,Surface treatment,Nanotechnology
Introduction
Dental implant is a prosthetic device made of alloplastic material (s) implanted into the oral tissues beneath the mucosal and/or periosteal layer, and on/or within the bone to provide retention and support for a fixed or removable dental prosthesis. Dental implants are poised to achieve significant growth as increasing populations become aware of the long term benefits associated with implant supported restorations and prostheses. Economically viable and technologically advanced dental implants are fast shifting from an optional surgical procedure to a more acceptable and much sought-after treatment option for missing teeth. Majority of dental implants today are made of commercially pure (cp) titanium (1-4 grades) or titanium alloys like Ti-6Al-4V. Factors that contribute to the ultimate success of an implant include the physiological conditions of the recipient, implant placement procedure, implant material, implant design and implant surface.
Implant surface character is one implant design factor that affects the rate and extent of osseointegration. Precisely how much of implant surface directly contacts bone, how rapidly this bone accrual occurs, and the mechanical nature of the bone/implant connection is influenced by the nature of the implant surface itself [1,2]. There is a range of micron level surface topography that enhances the adherent osteoblasts differentiation and extracellular matrix formation/mineralization. It has been shown that nano-surface topography effectively enhances extracellular matrix synthesis of adherent cells and provides a faster and more reliable osseointegration response as compared to conventional implant surface [3].
The impact of nanotechnology has begun to appear on the dental implant surface designs in a significant manner. The technology involves increasing the complexity of the surface topography with the addition of nanoscale molecules [Table/Fig-1] [4]. It is now an established fact that surface topography plays a determinant role in the osseointegration procedure. Titanium oxide nano-tubes have shown an increased osseointegration compared to conventional titanium surfaces. The surface holds promise for improving the longevity of dental implants. Since osteoblasts readily adhere to this novel surface, dental implants coated with TiO2 nanotubes could significantly improve healing following dental implant surgery [Table/Fig-2] [4]. Nanotechnology is bound to change the prospects and longevity of dental implants within the next decade. The article discusses distinct advantages of nanosurface modifications over conventional implant surface encompassing various aspects of dental implantology.
A cluster of titanium dioxide nanotubes
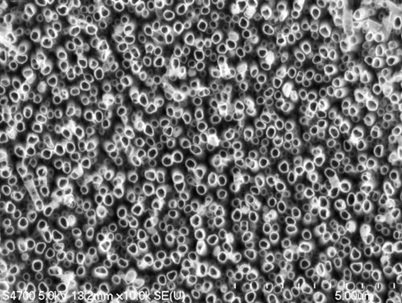
Bone cell anchoring to a surface of titanium dioxide nanotubes
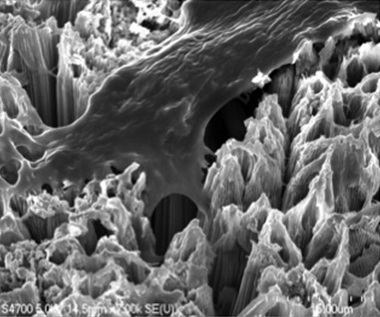
Nano Technology
Nanotechnology can be defined as the science and engineering involved in the design, synthesis, characterization and application of materials and devices whose smallest functional organization, in at least one dimension is on the nanometer scale or one billionth of a meter [5]. Nanotechnology therefore, involves materials that have a nano-sized topography or are composed of nano-sized materials ranging between 1 and 100 nm (10–9m) [6]. An evaluation of nano-mechanical properties of the surrounding bone by nano-indentation revealed that while both implants exhibited similar bone-to-implant contact, the nano-indentation demonstrated that the tissue quality was significantly enhanced around the hydroxyapatite (HA)-coated implants [7]. Nanotechnology may involve one-dimensional concepts (nanodots and nanowires) or the self-assembly of more complex structures (nanotubes). Materials are also classified according to their form and structure as nanostructures, nanocrystals, nanocoatings, nanoparticles, and nanofibers [6]. Nano-scale modification of the titanium endosseous implant surface may lead to alteration in the topography as well as chemistry of the surface. Therefore, the goal of nanoscale modification should be a specific chemical modification of cp titanium (grade 4 and 5). A distinct implication associated with nanoscale manipulation of any material is that it also leads to inherent chemical changes on the material surface. Albrektsson and Wennerberg [8] divided implant surface quality into three categories: i) mechanical properties, ii) topographic properties, and iii) physico-chemical properties. They pointed out that these characteristics are inter-related and a change in any of these groups affects the others as well. This significant observation is quite relevant to the study of nano-surface modifications of the endosseous cp titanium implant surface.
Methods of Imparting Nano Features
There are various methods to create nanoscale features at the implant surface. These methods include: i) Physical methods, like self-assembly of mono-layers, compaction of nano particles and ion beam deposition; ii) Chemical methods, like acid etching, peroxidation, alkali treatment (NaOH) and anodization; iii) Nano particle deposition like sol-gel (colloidal particle deposition) and discrete crystalline deposition; and iv) Lithography and contact printing technique [Table/Fig-3] [9].
Methods for creating nano-features on cp titanium implants
Methods | Characteristics |
---|
Self-assembly of monolayers | The exposed functional end group could be a molecule with different functions (an osteoinductive or cell adhesive molecule). |
Compaction of nanoparticles | Conserves the chemistry of the surface among different topographies. Not readily applied over implant surfaces. |
Ion beam deposition | Can impart nanofeatures to the surface based on the material used. |
Acid etching | Combined with other methods (sandblasting and/or peroxidation) can impart nanofeatures to the surface and remove contaminants. |
Peroxidation | Produces a titania gel layer. Both chemical and topography changes are imparted. |
Alkali treatment (NaOH) | Produces a sodium titanate gel layer allowing hydroxyapatite deposition. Both chemical and topographic changes are imparted. |
Anodization | Can impart nanofeatures to the surface creating a new oxide layer (based on the material used). |
Sol–gel (colloidal particle adsorption) | Creates a thin-film of controlled chemical characteristics. Atomic-scale interactions display strong physical interactions |
Discrete crystalline deposition | Superimposes a nanoscale surface topographical complexity on the surface |
Lithography and contact printing technique | Many different shapes and materials can be applied over the surface.Approaches are labor intensive and require considerable development prior to clinical translation and application on implant surface. |
One of the main concerns related to coating the implant surface is the risk of coating detachment and toxicity of related debris. An evaluation of relationship of particle size and cell viability and proliferation compared to micron-particles revealed that nanoparticles of titanium and alumina had less negative impact in cell viability and proliferation as compared to conventional particles. There may be an advantage to nanoscale modification of surfaces using sol–gel coating methods. The quantum interaction of high electron density at the atomic level can enforce high bond strength between the substrate and nano-scale coating [3]. Studies reveal that the addition of a nanometer-scale calcium phosphate treatment to a dual acid-etched implant surface appeared to increase the extent of bone development after 4 and 8 weeks of healing. It was observed that this rapid accrual of bone at the implant surface expedites the implant healing period and supports early loading protocols [10]. Some of the commercial establishment manufacturing dental implants have already started using the technology to modify their products. Others are on a fast track to catch-up through the process of research and development.
Nanotechnology and Cellular activity
Studies have highlighted the significance of micron-scale topography that compared various surface preparations of cp titanium to an electro-polished surface negative control-group and a hydroxyapatite coated positive control-group [11]. Their observation that a micron-scale rough surface prepared by grit blasting and subsequent acid etching was capable of rapid and increased bone accumulation further strengthened an earlier report that a TiO2 grit blasted surface also supported more rapid and increased bone accrual at cp titanium implants [12]. The study also pointed to a significant fact that the cp titanium surface could be modified to enhance bone accumulation and suggested that cp titanium was not only “bioinert” or ”biocompatible”, but was also capable to influence cellular activity or tissue responses leading to better and greater osteogenesis and thereby promoting better osseointegration. Nanotopography seems to influence cell interactions at surface of the material being used. It also leads to changed cell behavior when compared to conventional sized topography. The cellular protein adsorption is altered by nanoscale modification of bulk material. Depending on the nano-architecture, cell spreading may be increased or decreased. The present undefined mechanisms indicate that cell proliferation appears to be enhanced by nanoscale topography. Several investigators have shown that nanoscale topography enhances osteoblast differentiation [13–15] [Table/Fig-4] [15].
Depiction of broad range of nano-scale topography effects observed in cellular protein adsorption in bulk materials. Both cell specificity and extent of cell adhesion are altered. Depending on the nano-architecture cell spreading may be increased or decreased
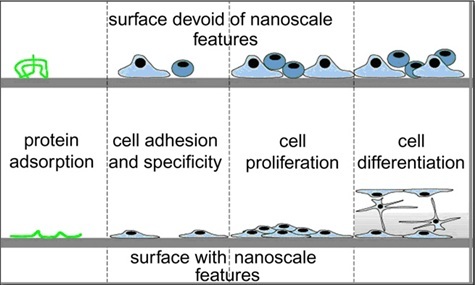
Protein/surface interactions (surface wettability) in Nano-surface
Alteration in initial protein surface interaction is believed to control osteoblast adhesion, a critical aspect of the osseointegration process [16]. When implant comes in contact with a biological environment, the initiation of protein adsorption (e.g. plasma fibronectin) promotes subsequent cell attachment and proliferation. Change in surface energy or wettability of a biomaterial corresponds to a typical way of altering cell interactions with the surface. Nano-scale topography is now an established way of altering protein interactions within a surface. Webster et al., demonstrated an increased vitronectin adsorption on nanostructured surfaces when compared to conventional surfaces [17,18]. Their study also suggested an increased osteoblast adhesion when compared to other cell types, such as fibroblasts, on the nanosurfaces [18].
Cell adhesion, spreading and motility in Nanosurface
Irrespective of the surface-adsorbed proteins, cells are remarkable in their ability to sense nanostructure. Nano-features of a surface affect both cell adhesion and cell motility. Both of these cell traits are attributed in part, to the function of integrins [18,19]. Underlying substratum topography influences cell behaviors by both direct and indirect interactions. In 20–40 nm features produced by H2O2/H2SO4 treatment there were definitive interactive points for lamellipodia of spreading cells [Table/Fig-5] [18]. Nano structure of alloplastic surfaces may have distinctive traits affecting cell interactions. Cell behavior is affected by both, the dimension and the density of the nano structures [20].
Nanoscale topography-cell interactions on a nano-surface produced by H2O2/H2SO4 treatment(A) 10,000_ image of adherent cell, (B) and (C) represent 100,000_images of the same adherent cell and (D) 200,000_ magnification of the cell with nano-features. (B) Higher magnification of the rectangle in (A). (D) Higher magnification of the rectangle in (B)
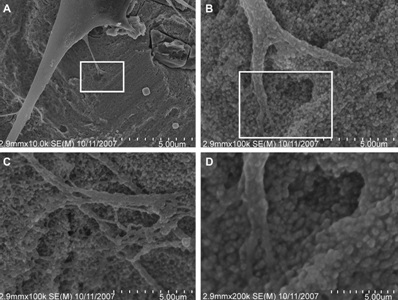
Proliferation in Nano-surface
Nanosurface modifications promote adherent cell proliferation. Zhao et al., utilizing three distinct methods – electrochemical machining, anodization and chemical etching to produce reproducible submicron-scale structures on titanium surfaces reported an opposing relationship between cell proliferation and cell differentiation with increase in micro scale of surface features [20]. It has further been supported by studies that observed an increase in the osteoblast proliferation on nano scale materials like alumina, titanium and hydroxyapatite [21,22]. However, the mechanism involved is still not clear as to how nanostructured surfaces modulate the adherent osteoblast response.
Selectivity of adhesion in Nano-surface
Selectivity of cell adhesion is an interesting feature attributed to nanoscale topographic surfaces. Several studies have revealed a relative lowering of fibroblast adhesion compared to osteoblast adhesion on evaluation of nano and micron-structured surfaces [23,24]. The affinity ratio between osteoblasts and fibroblasts was 3 to 1 on nanosized materials as compared to the conventional materials depicting a ratio of 1 to 1 [18]. Studies on other cell types such as smooth muscle cells and chondrocytes have also reported similar results [25]. All these observations may lead to some major implications in specification of tissue response at bone and mucosal surface of the dental implant/abutment assembly.
Differentiation
A rapid differentiation of adherent mesenchymal cells along the osteoblast lineage is as significant for the process of osseointegration as supporting osteoblast-specific adhesion and adherent cellular proliferation. Studies have revealed that alkaline phosphatase synthesis and calcium mineral content increased in cell layers formed on nanosized materials after 21 and 28 days [26, 27].
Nanosurface and bacterial proliferation
Another significant finding with nanosurfaces has been found to be a diminished bacterial adhesion and proliferation [28]. There was a marked decrease in bacterial colonization on nanostructured TiO2 and ZnO irrespective of the fact that these surfaces promote osteoblast adhesion and differentiation. These initial observations may suggest the need for further exploration of the implant abutment surface with focus on biofilm accumulation and peri-implantitis.
Nanosurface and surface-reactivity
Endosseous implant surface reactivity may get influenced by nanosurface modifications. Insignificant bone bonding occurs at endosseous titanium implants, especially during the initial phases of bone formation [29]. Nano-scale topographic modifications tend to change the chemical reactivity of materials and presence of bone on implant surface during early stages [30]. Bone bonding seems to be an advantage associated with titanium implants with nano-scale surface modifications.
Nano-surface implants in dentistry
Advantages of nanotopography on biomaterials have been demonstrated as early as 1999 by Webster et al., [25]. The nanotopography is linked to increased gene expression and is indicative of faster osteoblastic differentiation. Various options are available to impart nanoscale surface modifications on implants [Table/Fig-3]. Some of these have already been commercially deployed and clinically used to enhance bone response. A positive bone response on nanosurface biomaterials is already an established fact. Up to what extent the nanotechnology and nanotopography can be used to improve tissue and abutment interface bonding remain to be seen. Commercially available current implants using nanotopography may not have acquired the level of microstructure desired for the ultimate goal of perfection in the area. Further research and study in the field may open up new horizons of a more favorable use of titanium with nanosurface in implant dentistry. The development may well make dental implants last for life in the mouth with practically no failures.
Conclusion
Available research data may just be the tip of an iceberg on a very complex issue of nano-surface modifications in implants and an improved bone response associated with it. Nanosurface modification changes the chemical / biological reaction of the implant in the mouth. This altered behavioral pattern has been linked to a changed implant surface interaction with ions, biomolecules and cells. This change in interactions, in turn favorably influences molecular and cellular activities leading to altered osseointegration. It still needs to be explored whether this changed behavior can be attributed to the nanosurface modifications alone. The inherent benefits and limitations of nanostructure modifications on implant surface will become evident on further evaluation and clinical trials after their long term use in human mouth.
[1]. Cooper LF, Biologic determinants of bone formation for osseointegration: clues for future clinical improvements J Prosth Dent 1998 80:439-49. [Google Scholar]
[2]. Nanci A, Chemical modification of titanium surfaces for covalent attachment of biological molecules J Biomed Mater Res 1998 40:324-35. [Google Scholar]
[3]. Gutwein LG, Webster TJ, Increased viable osteoblast density in the presence of nanophase compared to conventional alumina and titania particles IJ Biomaterials 2004 25:4175-83. [Google Scholar]
[4]. Tolou Shokuhfar, Intercalation of anti-inflammatory drug molecules within TiO2 nanotubes JRSC Adv 2013 3(38):17380-6. [Google Scholar]
[5]. Silva GA, Introduction to nanotechnology and its application to medicine J SurgNeurol 2004 61:216-20. [Google Scholar]
[6]. Christenson EM, Nanobiomaterial applications in orthopedics J Orthop Res 2007 25:11-22. [Google Scholar]
[7]. Jimbo R, Nano Hydroxyapatite-coated Implants Improve Bone Nanomechanical Properties JDR 2012 91:121172-7. [Google Scholar]
[8]. Albrektsson T, Wennerberg A, Oral implant surfaces: part 1, Focusing on topographic and chemical properties of different surfaces and in vivo responses to them Int J Prosth 2004 17:536-43. [Google Scholar]
[9]. Webster TJ, Ejiofor JU, Increased osteoblast adhesion on nanophasemetals:Ti, Ti6Al4V, and CoCrMo IJ Biomaterials 2004 25:4731-9. [Google Scholar]
[10]. Goené RJ, Influence of a nanometer-scale surface enhancement on de novo bone formation on titanium implants: a histomorphometric study in human maxillae Int J Perio Restorative Dent 2007 27:211-9. [Google Scholar]
[11]. Gotfredsen K, Clinical and radiographic evaluation of submerged and nonsubmerged implants in monkeys Int J Prosth 1990 3:463-9. [Google Scholar]
[12]. Klabunde KJ, Nanocrystals as stoichiometric reagents with unique surface chemistry J Phys Chem 1996 100:121 [Google Scholar]
[13]. Wu SJ, Sintering of nanophase g-Al2O3 powder J Am Ceram Soc 1996 79:2207 [Google Scholar]
[14]. Baraton MI, FTIR study of nanostructured alumina nitride powder surface: determination of the acidic/basic sites by CO, CO2, and acetic acid adsorptions J Nano Mater 1997 8:435 [Google Scholar]
[15]. Balasundaram G, Using hydroxyapatite nanoparticles and decreased crystallinity to promote osteoblast adhesion similar to functionalizing with RGD IJ Biomaterials 2006 27:2798-805. [Google Scholar]
[16]. Webster TJ, Mechanisms of enhanced osteoblast adhesion on nanophase alumina involve vitronectin J Tissue Eng 2001 7:291-301. [Google Scholar]
[17]. Webster TJ, Specific proteins mediate enhanced osteoblast adhesion on nanophase ceramics J Biomed Mater Res 2000 51:475-83. [Google Scholar]
[18]. Brunette DM, The effects of implant surface topography on the behavior of cells Int J Oral Maxillofac Implants 1988 3:231-4. [Google Scholar]
[19]. Cavalcanti Adam EA, Cell spreading and focal adhesion dynamics are regulated by spacing of integrin ligands Biophys J 2007 92:2964-74. [Google Scholar]
[20]. Zhao G, Osteoblastlike cells are sensitive to submicron-scale surface structure Clin Oral Implants Res 2006 17:258-64. [Google Scholar]
[21]. Webster TJ, Enhanced functions of osteoblasts on nanophase ceramics IJ Biomaterials 2000 21:1803-10. [Google Scholar]
[22]. Price RL, Osteoblast function on nanophase alumina materials: influence of chemistry, phase, and topography J Biomed Mater Res A 2003 67:1284-93. [Google Scholar]
[23]. McManus AJ, Evaluation of cytocompatibility and bending modulus of nanoceramic/polymer composites J Biomed Mater Res A 2005 72:98-106. [Google Scholar]
[24]. Price RL, Nanometer surface roughness increases select osteoblast adhesion on carbon nanofiber compacts J Biomed Mater Res A 2004 70:129-38. [Google Scholar]
[25]. Webster TJ, Osteoblast adhesion on nanophase ceramics IJ Biomaterials 1999 20:1221-7. [Google Scholar]
[26]. Meirelles L, On nano size structures for enhanced early bone formation [Ph.D.] 2007 GothenburgJ Gothenburg University [Google Scholar]
[27]. Colon G, Increased osteoblast and decreased Staphylococcus epidermidis functions on nanophase ZnO and TiO2 J Biomed Mater Res A 2006 78:595-604. [Google Scholar]
[28]. Davies JE, Bone bonding at natural and biomaterial surfaces IJ Biomaterials 2007 28:5058-67. [Google Scholar]
[29]. Tasker LH, Applications of nanotechnology in orthopaedics Clin Orthop Relat Res 2007 456:243-9. [Google Scholar]
[30]. Ellingsen JE, Improved retention and bone-to-implant contact with fluoride-modified titanium implants IJ Oral Maxillofac Implants 2004 19:659-66. [Google Scholar]