Vigabatrin (VGB) is an Antiepileptic Drug (AED) which has been used in refractory epilepsy and Infantile Spasms (IS) for more than 10 years but had a restricted usage due to some concern about its safety profile, as it can cause retinal toxicity [1–4]. However, VGB has now been approved by the US Food and Drug Administration (FDA) as monotherapy for IS. A number of studies supported the use of VGB in the symptomatic treatment of IS, making it the drug of choice in this condition [5–7]. The success is more in cases of tuberous sclerosis complex where the drug controls spasms in up to 95% of patients [8–10]. The response is better with higher doses of VGB as compared with low-dose [11]. When VGB is compared with hormonal treatment (with corticosteroids) for IS, patients treated with VGB have shown a better control of spasms and better cognitive outcome [12].
VGB acts as a selective inhibitor of gamma amino butyric acid transaminase (GABA-T), an enzyme that degrades Gamma Amino Butyric Acid (GABA) in the brain. Thus, VGB administration results in elevated brain GABA levels. It is thought that epileptic seizures may be due to low levels of GABA. By increasing the amount of GABA, Vigabatrin reduces the likelihood of an epileptic seizure [13,14]. VGB has a favorable pharmacokinetic profile since, it is not metabolized by the liver (does not induce the hepatic cytochrome p 450 system), is excreted by the kidney, has minimal protein binding, and has a long effective half-life, allowing once- or twice-daily dosing. Interaction with other antiepileptic drugs is minimal [15].
Clinical experience in humans shows that Vigabatrin provides effective seizure control and has excellent tolerability. However, long term usage and higher doses of this drug usually leads to certain visual field defects. Micro–vacuolation in different parts of the brain has also been noted in various animal studies. All these have been extensively studied but other side effects including ataxia, tremors and abnormal gait [16] have received little attention. These effects indicate towards an involvement of the cerebellum but the pathogenesis remains poorly understood.
The aim of the present study was to see the histopathological effects of this drug on the cerebellar tissue of albino rats. The findings may help in guiding the treating physician to include certain tests to assess cerebellar function also apart from monitoring the visual system during the course of treatment.
Material and Methods
Sixty albino rats (Rattus norwegius, Wistar strain) of both sexes, weighing 150-200gm were taken from the central animal house of the institute, after obtaining permission from the Institutional Animal Ethical Committee. When procured, the rats were healthy and free from any disease or disability.
The rats were housed in cages and kept under the same environmental conditions which included 12 hour light/dark cycles. They were fed with standard rat feed and allowed water ad libitum with daily monitoring of the body weight.
The animals were divided into two main groups:
Group 1- Control group of 15 rats.
Group 2- Experimental group of 45 rats.
The experimental group was divided into 3 subgroups-
Subgroup 2a, 2b & 2c having 15 rats each.
Each of the three subgroups was given intraperitoneal Vigabatrin in doses of:
Subgroup 2a → 125 mg/kg body weight
Subgroup 2b → 250 mg/kg body weight
Subgroup 2c → 500 mg/kg body weight
The drug was administered as a single dose daily for a period of 28 days. All the doses were calculated according to the standard guidelines for drug administration to rats in animal studies. Simultaneously, the control group was given an equivalent dose of intraperitoneal injection of normal saline-0.9%. All the animals were weighed on a weighing machine prior to initiation of drug therapy and thereafter daily at each dose administration.
After four weeks of treatment for each group, the rats were sacrificed after giving deep anaesthesia by ether inhalation. A mid line incision was given, starting from the dorsal aspect of upper part of the neck and was extended to the head. After reflecting the scalp, the dorsal calvarium was removed in small chips, taking care not to damage the underlying brain tissue. The brain was carefully dissected out. The cerebellum was slightly lifted up and the cerebellar peduncles were cut, thus separating the cerebellum from the brainstem. The cerebellum was separated from the rest of the brain. The two cerebellar hemispheres were separated by a sagittal cut passing through the vermis. The tissues were washed with normal saline and fixed in the fixative (10% formal saline containing 100 ml of formalin, 8.5 grams of sodium chloride and 900ml of water) contained in glass bottles with glass stoppers which had been properly labelled beforehand. Tissue processing was done. Sagittal histological sections of 4-5 μm thickness were taken and stained with routine Haematoxylin and Eosin stain. Special stains (Beilschowsky’s Silver Stain and Modified Luxol Fast Blue Stain) were also used to look for any axonal degeneration and demyelination. Slides were observed under the light microscope in low and high magnifications.
Observations
Features suggestive of neuronal cell loss were seen in the cerebellar cortex of rats in all the subgroups receiving intraperitoneal VGB. The control group showed a normal histological picture.
The cerebellar cortex is normally made up of three layers i.e. the outer Molecular layer, middle Purkinje cell layer and inner Granular cell layer [Table/Fig-1].
Control group cerebellar cortex showing the three layers; ML: Molecular layer, PCL: Purkinje cell layer and GL: Granular cell layer. (H&E Stain X 200)
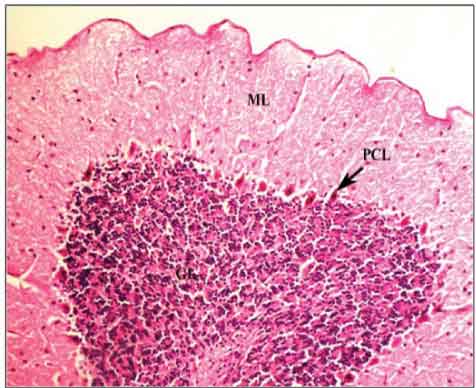
Normally, the axons of Purkinje cells are myelinated and can be seen passing through the Granular cell layer in Luxol Fast Blue stained slides (a stain which specifically stains the myelin sheath). In the present study, decreased number of myelinated axonal fibres passing through the Granular layer was noticed in all the treated groups as compared to the control, which could be due to a decreased number of Purkinje cells, indicating neuronal cell loss. The high dose group showed an almost negligible number of these fibres [Table/Fig-2,3]. The cell loss was confirmed and further assessed by two parameters.
Control group cerebellar cortex showing myelinated axons of Purkinje cells (arrows) passing through the Granular layer (GL). (Modified Luxol Fast Blue Stain X200)
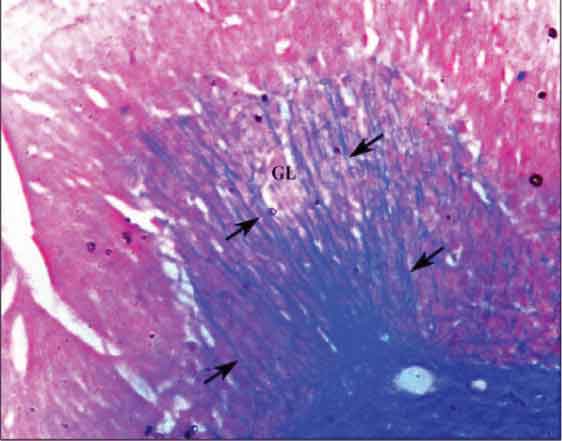
Cerebellar cortex of subgroup 2a showing decreased number of myelinated axons of Purkinje cells (arrows) passing through the Granular layer (GL). (Modified Luxol Fast Blue Stain X200)
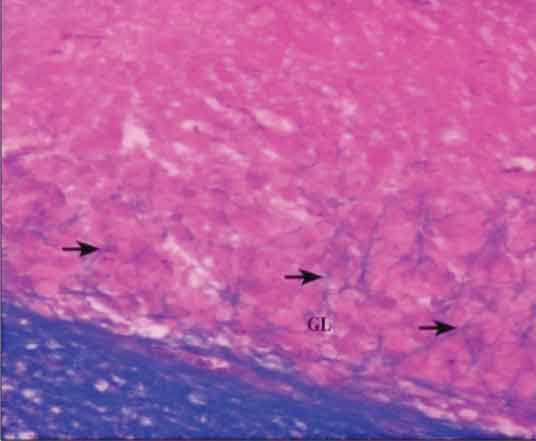
(a) Cell Count
Cell counts were done in all three layers of the cerebellar cortex of each rat in ten random fields under high power. The average values of the number of cells for each layer and standard deviation were calculated. Student’s t-test was applied and p values were drawn which showed a highly significant decrease in the cell counts as compared to the control in Purkinje and Granular cell layers of moderate and high dose groups (p–values <0.01) while in the molecular layer highly significant decrease was found in the high dose group only (p–value <0.01) [Table/Fig-4].
Mean values of cell counts per high power field in different layers of the cerebellar cortex in different dose groups
Layers | Control Mean ± SD | Group 2a Mean ± SD | Group 2b Mean ± SD | Group2c Mean ± SD |
---|
Molecular layer | 25.6 ± 4.53 | 24.3 ± 5.12 p value 0.3153 | 24.1 ± 2.13 p value 0.2221 | 19.9 ± 2.92 p value 0.0009 |
Purkinje cell layer | 11.8 ± 2.39 | 11.8 ± 2.25 p value 0.5 | 7.4 ± 2.55 p value 0.0032 | 5.8 ± 2.15 p value 0.0004 |
Granular layer | 503.6 ± 21.90 | 494.8 ±35.01 p value 0.267 | 455.2 ± 38.59 p value 0.0003 | 393.5 ± 34.00 pvalue 0.6061E+06 |
(b) Distance Between Two Purkinje Cells
The mean distance between two Purkinje cells was measured per high power field in ten random fields with an eyepiece micrometer. A highly significant increase as compared to the control was found in moderate and high dose groups (p–values <0.01) [Table/Fig-5,6,7,8and9].
Comparison of mean values of the distance (in μ) between two Purkinje Cells per high power field in control & different dose groups
| Control Mean ± SD | Group 2 a Mean ± SD | Group 2 b Mean ± SD | Group2 c Mean ± SD |
---|
Distance between two Purkinje cells(in μ) | 17.72 ± 4.02 | 17.48 ± 2.97 p value 0.445 | 30.02 ± 9.68 p value 0.006 | 39.75 ± 15.69 p value 0.002 |
Control cerebellar cortex showing Purkinje cells (arrow). (Beilschowsky’s Silver Stain X400)
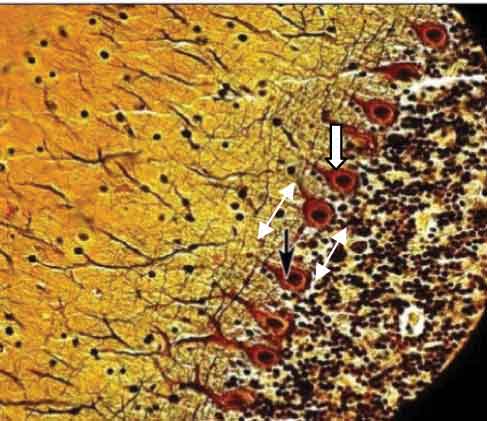
Cerebellar cortex of subgroup 2c showing marked decrease in number of Purkinje cells and increased distance between two cells (Beilschowsky’s Silver Stain X400)
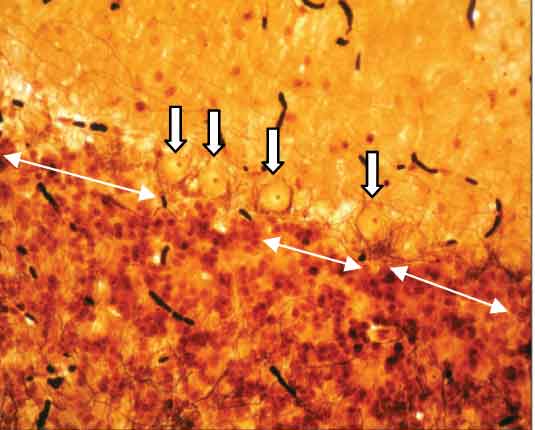
Control cerebellar cortex showing Purkinje cells (arrow). (H&E Stain X400)
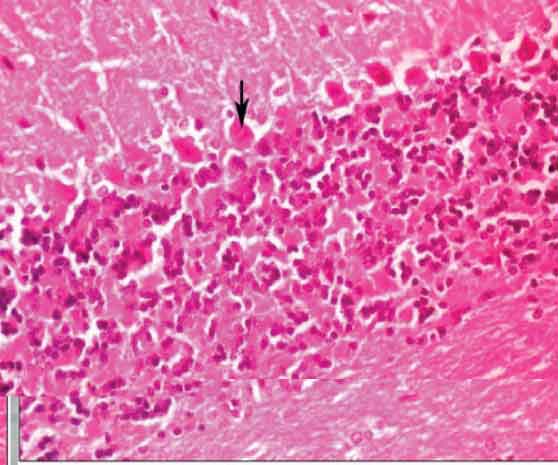
Cerebellar cortex of subgroup 2c showing complete loss of Purkinje cells in the field. (H&E Stain X400)
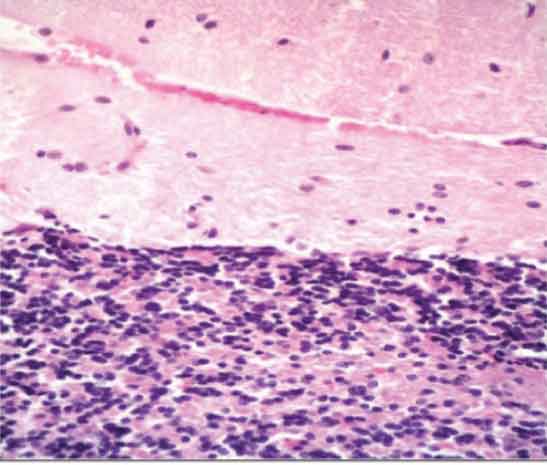
No signs of axonal degeneration were found in the experimental group of rats.
Discussion
VGB is an AED that has demonstrated significant efficacy as adjunctive therapy for patients with poorly controlled partial epilepsy, refractory to other AEDs and has a very important role in IS. Cerebellar childhood epilepsies present as a big challenge for every paediatric neurologist and IS represent a particularly urgent and difficult-to-treat syndrome. The approval of this new drug by the FDA as an alternative treatment of IS represents a major contribution to this area as the only other treatment available is hormonal [17]. Superior cognitive outcome has been reported following VGB treatment as compared to treatment with hormones in cases of IS where a known etiology like Tuberous sclerosis complex is present [18].
Despite the clinical importance of this drug, various side effects associated with its use cannot be neglected. Exhaustive work has been done on finding out the basis of the visual defects which are commonly seen after its usage but little attention has been given to others like ataxia, gait abnormalities and tremors which indicate towards an involvement of the cerebellum.
In the present study, cell loss in the cerebellar cortex was the main feature in the experimental group. This loss increased with increasing doses of VGB. Elevated GABA levels secondary to inhibition of GABA-T may be involved in the pathogenesis as they are toxic to the neurons and neuroglia in the brain. This toxicity could be a result of direct injury by GABA or an indirect injury due to its metabolite, glutamate.
Glutamate is the dominant excitatory amino acid and the primary neurotransmitter in about one-half of all the synapses in the brain [19]. It is responsible for many important neurologic functions including cognition, memory, movement, and sensation. In pathologic conditions, glutamate mediates neuronal injury or neuronal death, particularly through activation of the N-methyl-D-aspartate (NMDA) receptor subtype of the glutamate receptors. Neuronal glutamate is released from the presynaptic terminal of neuronal axons into the synaptic cleft and then works as a neurotransmitter. Reuptake of extracellular glutamate takes place at the presynaptic terminals and adjacent glial cells, energy for this reuptake being provided by the mitochondria. Excessive glutamate binding to NMDA receptors allows entry of Ca2+ into the post–synaptic neuron, causing necrotic cell death or apoptosis, whereas the excessive glutamate binding to non-NMDA receptors allows entry of Na+ into the post–synaptic neuron, resulting in cytotoxic edema. Because glial cells also have these receptors, excessive glutamate leads to glial cell damage as well [20]. This could have been the underlying mechanism behind the cell loss in the present study too. Since we did not use stains specific for neuroglial cells in our study it is difficult to say whether the damage involved the neuroglia too.
Qiao et al., observed the neuropathological findings & did biochemical assays in the brain of rats after giving injectable VGB in three different doses for 2 weeks. They found a reduction in myelination, reduced activities of myelin and oligodendrocyte associated enzymes and decrease in myelin basic protein on Western Blots, confirming toxic damage to the oligodendrocytes which are the cells responsible for myelination in the central nervous system [21].
Butler et al., observed the effects of three different doses of VGB given for 90 days on the central nervous system of albino rats. They found that neuronal cell bodies as well as oligodendroglial cell bodies in different parts of the brain were normal [22]. The findings in the present study are contradictory to those of Butler et al.
Conclusion
Although VGB is well tolerated and has the most favourable improvement rates in IS as compared with other newer AEDs, it may be neurotoxic, particularly affecting the cerebellum. Therefore, we suggest that tests for assessing cerebellar function should be done at the beginning of treatment and thereafter at regular intervals which will help in determining the onset of toxicity. Early assessment of efficacy and ongoing evaluation of the benefits and risks of Vigabatrin therapy should be done. The potential for seizure reduction must be weighed against safety risks.