While studying the blood gases from a 5 years cohort of neonatal admissions, a surprising observation was made in 6 infants admitted with pulmonary hypoplasia, 2 from diaphragmatic hernia and 4 from anhydramnios due to various causes. Eighteen blood gases were obtained from them in the first 18 hours. When 3 samples affected by perinatal asphyxia were removed, remarkably precise and unexpected relationships appeared between arterial carbonic and lactic acids, as shown in [Table/Fig-1]. As arterial blood carbonic acid increased up to PCO2 of 120mmHg, lactate decreased to as low as 0.5mmol/L, rather than increasing as most would expect, and only increased modestly from worsening hypoxia as PCO2 rose above this to 250mmHg.
At first sight the most plausible explanation for this unexpected relationship seemed to be that under ventilation causes vasodilation, improving tissue perfusion and lowering lactate, whereas over ventilation causes vasoconstriction and decreased venous return, reducing tissue perfusion and increasing lactate.
Only after analyzing this cohort of blood gases and reviewing historical literature did it become clear that this relation is in fact primarily a physiological one, determined by the cell membrane anion exchange mechanism and the Gibbs-Donnan law of equilibrium. As a part of his enormous body of pioneering work, Donald van Slyke described the physiological basis of this relation in 1923 [1]. In 1919-20, other pioneers reported that experimental alkalosis causes lactic acidosis, and twice independently demonstrated that exposure to low oxygen tension produces alkalosis characterized by the concomitant appearance of lactic acid in blood and urine [2]. This led Howard Haggard and Yandell Henderson to recognize that when PCO2 increases the very weak nature of carbonic acid protects intracellular pH from the stronger organic and mineral acids, but when PCO2 falls these strong acids replace carbonic acid compromising cell function [3].
Material and Methods
Over a 68 months period from 1996 to 2001, lactate was measured on all blood gas samples taken as clinically required from newborn babies admitted after delivery to the neonatal unit in New Plymouth, New Zealand. Whenever electrolyte measurements were also needed, all 6 macro minerals, protein, urea and creatinine were measured on the same blood samples as used for blood gases, to allow mineral base and organic acid to be calculated [4]. This was done in order to prospectively evaluate a new neonatal TPN regimen [5]. The results were recorded with antenatal, delivery and postnatal events, clinical and laboratory details and treatments.
To establish how changes in carbonic acid effect lactic and organic acids, all arterial blood samples from infants given high calcium TPN were analyzed. These infants were chosen because they had the greatest requirements for ventilator support and hence need for blood gases in the cohort, and because their parenteral fluid therapy caused no acid base disturbance [6]. Continuous positive airways pressure (CPAP) was given by bubble device with binasal Hudson prongs [7] and intermittent positive pressure ventilation (IPPV) by Draeger ventilators.
The effects of perinatal asphyxia were excluded, by removing samples with high and falling lactate soon after delivery. Blood samples taken while these infants were receiving dexamethasone were also not included.
Carbonic acid mmol/L = 0.0306 X PCO2 mmHg
Results were expressed as mean ± sample standard deviation (SD) and analysis performed with standard parametric tests, linear, power and logarithmic regression analysis.
Results
Excluding samples with high and falling lactate soon after delivery and those on dexamethasone, a total of 1231 arterial blood PCO2 measurements with lactate and 696 with organic acid were made in 63 infants, before or while they received high calcium TPN. They had the following birth weights and gestational ages:
24-29 weeks 21 infants 21 infants 991 ± 268g 26.7 ± 1.6 wk
30-36 weeks 37 infants 37 infants 1857 ± 474g 32.4 ± 1.7 wk
37-41 weeks 5 infants 3231 ± 887g 39.2 ± 2.0 wk
Fifty one had respiratory distress syndrome and 2 had meconium aspiration syndrome. Twenty two needed CPAP only, and 31 needed IPPV and surfactant followed by CPAP. All survived and were free of infection. Having excluded samples affected by asphyxia, otherwise unexplained lactic acidosis affected some of these infants during the first 48 hours, as shown in [Table/Fig-2].
Arterial lactate during the first 6 weeks in 63 infants given 1998 TPN, excluding samples with high and falling levels soon after delivery
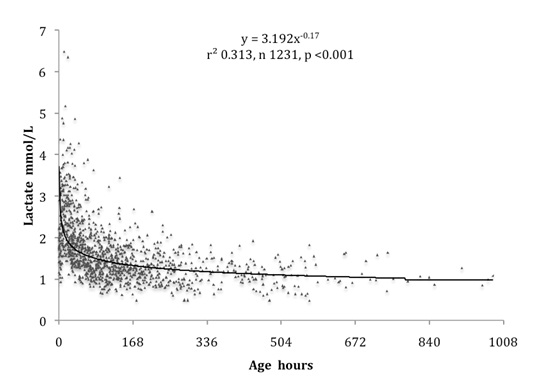
A strong inverse relation was found between carbonic and lactic acids in infants of all gestations, as shown in [Table/Fig-3]. High lactic for carbonic acid measurements were obtained mainly from infants below 30 weeks gestation. Term infants had a similar relation to that seen in the 4 infants with pulmonary hypoplasia, but with lower lactic acid for carbonic acid levels. This inverse relation between carbonic and lactic acids has the hallmarks of the expected inverse physiological relationship imposed by the Gibbs-Donnan equilibrium. Including samples from infants with pulmonary hypoplasia with PCO2 greater than 100 mmHg, but excluding all those with PCO2 greater than 121 mmHg provided the best estimate of this relation. As shown in [Table/Fig-4], this estimate is slightly greater than the value 60 / PCO2 mmHg, which may be conveniently taken as a measure of the physiological lactic acid in mmol/L for any PCO2 up to 120 mmHg.
Arterial lactic acid increased as carbonic acid fell in infants of all gestational ages, with high lactic levels for carbonic acid being mainly found in below 30 weeks gestation infants
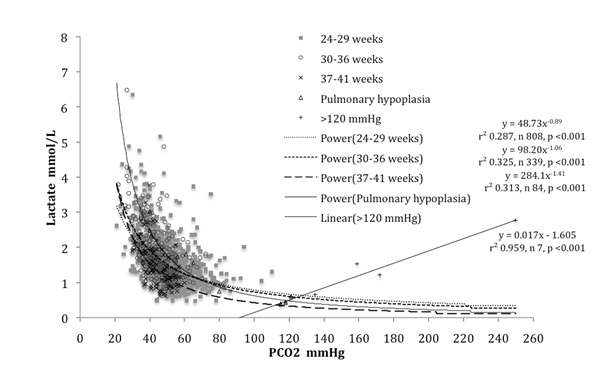
Arterial carbonic lactic acid correlation fits well with 60 ÷ PCO2 (mmHg) = physiological lactic acid (mmol/L) 68 of 1232 (5.52%) samples had values above twice physiological lactic acid
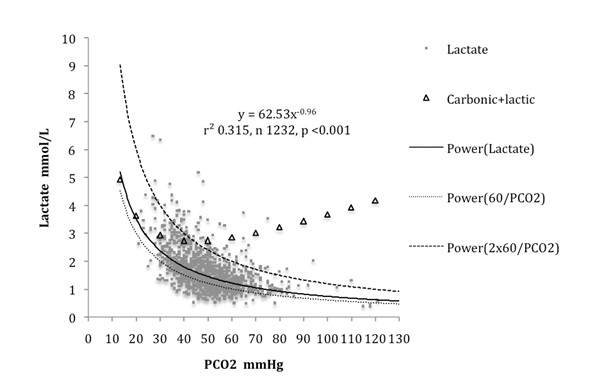
The sum of carbonic and physiological lactic acids increased gradually from 2.72 mmol/L to 4.17 mmol/L as carbonic acid trebled from 40 to 120 mmHg, but increased more rapidly to 4.92 mmol/L as carbonic acid fell to one third its normal value, as shown in [Table/Fig-4].
An abnormally high lactate for carbonic acid can be defined as a lactate more than double the physiological lactic acid. Overall the proportion of samples with abnormally high lactate for carbonic acid was 5.52%. As shown in [Table/Fig-5], this proportion was low in samples obtained from infants who did not need respiratory support and was significantly higher in samples obtained from those needing IPPV than CPAP. These differences were not evident in lactate levels alone.
Effects of IPPV and CPAP on arterial lactate and on the proportion of samples with lactate above twice physiological lactic acid during the first week
| IPPV | CPAP | No support | p IPPV v CPAP |
---|
Arterial samples n | 482 | 232 | 162 | |
Lactate mmol/L | 1.87 ± 0.85 | 1.79 ± 0.63 | 1.69 ± 0.61 | ns |
>120/PCO2 | 9.13 ± 2.57% | 4.74 ± 2.73% | 2.47 ± 2.39% | <0.05 |
A similar strong inverse relation between carbonic and organic acids was found as shown in [Table/Fig-6]. While carbonic and lactic acids are independent, carbonic and organic acids are not, since bicarbonate is used to calculate organic acid. The fact that carbonic acid influenced organic acid in a very similar way to lactic acid adds validity to the measurements of organic acid. The relation between carbonic and organic acids was independent of glomerular filtration rate (GFR), being found at a significant level in each group when classified according to GFR: <0.67, 0.67-0.99, >1.00 L/kg/day [6].
Arterial blood carbonic acid was inversely related to organic acid
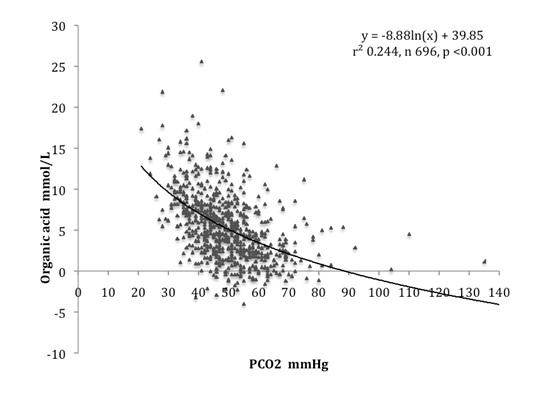
Discussion
Cell membrane anion exchange and the Gibbs-Donnan equilibrium
In order to maintain the Gibbs-Donnan equilibrium, changes in carbonic acid cause inverse changes in lactic acid, resulting from interchange of their anions through the cell membrane anion exchange mechanism. Changes in carbonic acid cause similar inverse changes in organic acid. The considerable magnitude of this physiological effect does not appear to have been previously reported. The physiological lactic acid for any given PCO2 up to 120 mmHg is conveniently given by dividing 60 by the PCO2 in mmHg. Three fold increases in PCO2 from 40 to 120 mmHg cause three fold fall in lactic acid from 1.5 mmol/L to 0.5 mmol/L, so that the sum of carbonic and lactic acids increases from 2.72 mmol/L to only 4.17 mmol/L. This is clearly an effective way to buffer carbonic acid and certainly helps to explain the neuro-protective effect of hypercarbia [8,9].
However, decreases in PCO2 rapidly increase lactic and organic acids, highlighting the importance of avoiding any degree of hyperventilation in ventilated preterm infants. This may exacerbate pre-existing lactic acidosis from circulatory compromise or organic acidosis from low glomerular filtration rates, made worse by suboptimal TPN [6]. This helps to explain how hypocarbia causes periventricular leucomalacia and neurodevelopmental deficit [10–12].
Lactate measurements more than 120 / PCO2 in mmHg may be regarded as abnormally high, indicating some pathological process. Such measurements were found mainly in below 30 weeks gestation infants during the first 48 hours, particularly in those requiring ventilation, and were unlikely in those on CPAP or free of support. These pathological measurements may signify circulatory compromise from positive pressure ventilation decreasing venous return. At the time reducing ventilation and administering blood were effective means of restoring these high lactate for PCO2 levels to normal. The validity of this hypothesis should now be tested by comparing lactate for PCO2 with other measures of circulatory status, such as superior vena caval flow made on Doppler echocardiography [13,14].
Current recommendations are that TPN for very preterm babies should have a high protein content, over 30g/L of amino acids, but with only up to 10% glucose [15]. Such TPN intakes provide as little as 22kcal/g amino acids [16]. This causes amino acid catabolism to be increased to meet energy needs, usually leaving insufficient protein for growth. A recent report on infants receiving such TPN described increases in blood urea in proportion to the amount of protein given, but no significant differences in bicarbonate [17]. This does not exclude serious organic acidosis from increased protein catabolism. On TPN providing only 25 kcal/g amino acids and containing gluconate and sulphate, serious organic acidosis was completely masked by mineral alkalosis with normal bicarbonate and base excess [6]. The highest organic acid levels, around 20mmol/L, were consistent measurements in one baby between 48 and 96 hours with PCO2 between 37 and 43 mmHg. Similar levels were associated with disability following acute and chronic perinatal asphyxia [18]. This baby was the only one in the study to later develop otherwise unexplained periventricular leucomalacia.
Measurements of organic acid, ammonia, amino acids and ideally sulphate and urate are urgently needed to ensure that such high protein TPN regimens are safe, particularly during the first few days when glomerular filtration rates are low as well as sometimes PCO2.
In well and poorly grown preterm infants on high calcium TPN, providing 30kcal/g amino acids and containing no gluconate or sulphate, increasing protein intake caused no increase at all in urea excretion, indicating no increase in protein catabolism [19]. These infants had normal organic levels for their glomerular filtration rates [6].
Sequential patient analysis
This paper is the last of 6 reports based on data obtained from sequential patient analysis (SPA). This system produces good evidence on which to base clinical decisions from very detailed clinical observations made on a relatively small number of patients. It involves methodically recording on all patients a predetermined, comprehensive and interlinked group of routine clinical measurements. These are then analyzed by a predetermined system to provide accurate information regarding the area of clinical concern. Any change in care can be assessed by change in these measurements. This system of analysis is prospective, but different from randomized controlled trials (RCT) because much more clinical information is systematically recorded in an easily analyzed form without issues of selection bias. Unfortunately SPA does not meet with the approval of our current RCT obsession, despite the fact that it is a much more powerful tool, as has been pointed out by other fans, who describe SPA as “statistical process control” or “quality improvement research”[20,21].
SPA researchers work with teams of professionals who aim to do their best possible job for the patients. The SPA researchers obsessively record all the relevant clinical data in an analyzable form while maintaining strict patient confidentiality. The public like this approach and do not expect ethical approval to first be needed. But without it the work acquires pariah status academically. This is unjustified because properly conducted SPA research is a very powerful way of assessing certain aspects of patient care. These are often areas that cannot be addressed by RCT research. Furthermore doubters of SPA research can easily verify its claims by comparing their clinical measurements.
These SPA studies have confirmed important knowledge that helps care for newborn babies and it would be unethical not to submit the reports to the most authoritative publisher.
They have confirmed that changes in mineral base as well as carbonic and organic acids alter arterial blood pH. They have also provided the evidence to design a TPN regimen that allows very premature babies to grow normally with no acid base or bone mineral disturbance. They have confirmed the prognostic value of lactate measurements, and explained why they are superior to base deficit. Finally they have demonstrated that carbonic acid causes inverse changes in lactic and organic acids, an important aspect of physiology to consider when ventilating infants.
Now it is hoped that the time is ripe for this theory of acid base balance to become incorporated into standard acid base teaching, so that its benefits can be widely appreciated. One hundred years ago the original theory of acid base balance recognized mineral base, but as blood gas analyzers were developed, the convenience of using bicarbonate as a measure of the metabolic component became widely appreciated, and the role of mineral base was largely ignored. Recognizing how mineral base balances organic and carbonic acids changes the once bewildering subject of acid base balance into a simple, logical and readily applicable one.