Enterobacteriaceae are the most common UTI pathogens in community and hospital settings [1]. Due to an increase in the antibiotic usage, antibiotic resistance is a great concern globally [2]. Increased antimicrobial resistance among Enterobacteriaceae poses a challenge in choosing appropriate empiric therapy especially when MDR Enterobacteriaceae are the suspected pathogens causing infection [3].
The most common cause of resistance among Enterobacteriaceae is production of ESBLs and AmpC beta-lactamases (AmpC). AmpC beta-lactamases initially received less consideration globally, but now it has become a growing problem [4]. AmpC β-lactamases are cephalosporinases that confer resistance to cefoxitin, cephalothin, cefazolin, most penicillins and β-lactam/β-lactam inhibitor combinations [5].
Gram negative organisms express AmpC β-lactamases either by deregulation of the ampC chromosomal gene or by acquisition of the ampC gene by plasmid which are commonly called as plasmid-mediated AmpC β-lactamases (pAmpC) [6]. The presence of pAmpC producing Enterobacteriaceae has been reported worldwide including Spain, Turkey, Libya, France, Algeria, Egypt and India [4,7].
Detection of organisms expressing AmpC β-lactamases is a necessity for addressing surveillance, for hospital infection control issues as well as for choosing optimal antimicrobial therapy [6]. The purpose of this study was to evaluate the antibiotic susceptibility pattern (antibiogram) of Enterobacteriaceae strains isolated from urine samples, to find out the prevalence of AmpC β-lactamase and its genotypes in a tertiary care hospital from Southern India.
Materials and Methods
This cross-sectional study based on clinical laboratory samples collected randomly during the period of July 2019 to February 2020 at the Rajah Muthaiah Medical College, Annamalai University, Chidambaram, Tamil Nadu, India was conducted. The study received Institutional Board Review approval from the Ethics Committee of the Annamalai University, Chidambaram (IHEC/525/2019).
Inclusion and Exclusion criteria: The dataset includes 60 Enterobacteriaceae strains (40 Escherichia coli samples, 16 Klebsiella pneumoniae samples, and three Proteus species and one Enterobacter species samples) from 33 females and 27 male subjects. Only urine samples recovered from UTI due to Enterobacteriaceae isolates were included in the study. Repetitive urine samples were excluded in the study. Isolates were collected from the microbiology laboratory. Hence, no informed consent was obtained.
All the isolates were identified by standard biochemical reactions and antibiotic susceptibility was performed by Kirby Bauer method as per Clinical and Laboratory Standard Institute (CLSI, formerly National Committee for Clinical Laboratory Standards (NCCLS)) guidelines 2018 [8].
AmpC β-lactamase detection: Initial screening for AmpC production was done by the Kirby Bauer disc diffusion method with 30 μg cefoxitin. Bacterial isolates that yielded a zone diameter of less than 18 mm were considered as positive for AmpC production [9]. Phenotypic confirmation of AmpC was by Inhibitor based method using cefoxitin and boronic acid.
Cefoxitin-boronic acid disks were prepared by dissolving 120 mg of phenylboronic acid in 3 mL of dimethyl sulfoxide. A 3 mL of sterile distilled water was added to the prepared solution. A 20 μL (400 μg) of the stock solution was dispensed on the cefoxitin disks (30 μg). Mueller Hinton Agar plates were inoculated with the bacterial isolate. Cefoxitin and cefoxitin with boronic acid discs were placed on the inoculated plates and incubated overnight at 37°C. An increase in zone diameter of more than 5 mm in the presence of cefoxitin with boronic acid in comparison with cefoxitin alone was considered to be positive for AmpC β-lactamase production [Table/Fig-1] [9].
Phenotypic analyses of bacterial isolates. Representative result of the Inhibitor based assay to identify AmpC-producing isolates with FOX (cefoxitin alone) and FOX+BA (cefoxitin+boronic acid) test discs.
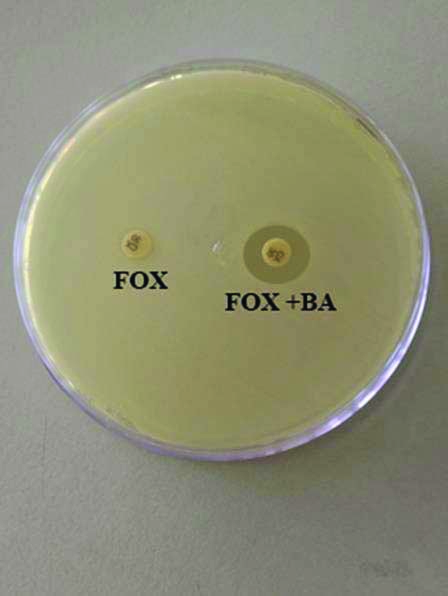
Molecular characterisation of AmpC β-lactamase: Multiplex Polymerase Chain Reactions (PCR) was used to detect the most common plasmid-mediated AmpC genes: ACC (expected amplicon size 346 base pairs), FOX (expected amplicon size 190 base pairs), MOX (expected amplicon size 520 base pairs), DHA (expected amplicon size 405 base pairs), CIT (expected amplicon size 462 base pairs), and EBC (expected amplicon size 302 base pairs) [9]. The set of PCR primers used which are specific to respective organisms is given in [Table/Fig-2].
Polymerase Chain Reaction (PCR) primers for amplification of AmpC genes.
Gene | PCR primer for amplification |
---|
MOX (520 base pair) | Forward: GCTGCTCAAGGAGCACAGGATReverse: CACATTGACATAGGTGTGGTGC |
CIT (462 base pair) | Forward: TGGCCAGAACTGACAGGCAAAReverse: TTTCTCCTGAACGTGGCTGGC |
DHA (405 base pair) | Forward: AACTTTCACAGGTGTGCTGGGTReverse: CCGTACGCATACTGGCTTTGC |
ACC (346 base pair) | Forward: AACAGCCTCAGCAGCCGGTTAReverse: TTCGCCGCAATCATCCCTAGC |
EBC (302 base pair) | Forward: TCGGTAAAGCCGATGTTGCGGReverse: CTTCCACTGCGGCTGCCAGTT |
FOX (190 base pair) | Forward: AACATGGGGTATCAGGGAGATGReverse: CAAAGCGCGTAACCGGATTGG |
DNA extraction was by Modified Proteinase K method [10]. For PCR assays (Department of Molecular Biology and Immunology, MMNGH Institute of Dental Sciences and Research Centre, Belgaum for Multiplex PCR), 2 μL cDNA was added to 23 μL master mixture of PCR reagents. The reaction was programmed with initial denaturation step at 94°C for three minutes; followed by 25 cycles of DNA denaturation at 94°C for 30 seconds, primer annealing at 64°C for 30 seconds, primer extension at 72°C for one minutes; and a final extension step at 72°C for seven minutes [9].
Detection of amplified products (Agarose gel Electrophoresis): Amplified products were subjected to electrophoresis through 3% Agarose gel containing 1x TAE (Tris Acetate EDTA buffer), 16 μL of each amplified product were loaded into each well. Electrophoresis was performed at 25V for two hour. The gel was visualised under UV light illuminator after staining with Ethidium bromide (0.5 μg/mL). The gel image was captured and analysed using Gel Documentation System (Major Science, USA). A 100 base-pair DNA ladder was used as the size reference and PCR mix with distilled water was used as negative control [Table/Fig-3].
Genotypic analyses of bacterial isolates. Representative gel electrophoresis result of Multiplex PCR products derived from bacterial isolates.
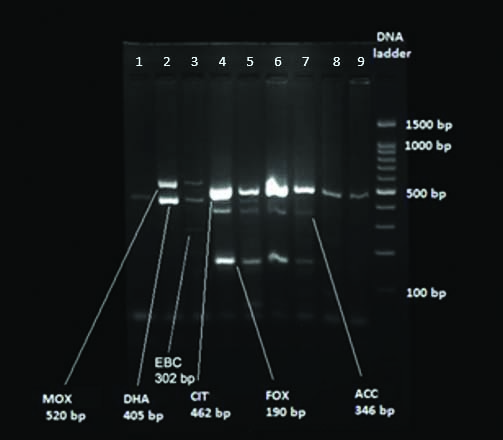
Statistical Analysis
Statistical Package for the Social Sciences (SPSS) version 20.0 (IBM, Armonk, NY, United States of America) were employed to obtain descriptive data.
Results
Escherichia coli represent the dominant AmpC-β-lactamase-positive bacterial strain among urine isolates.
Among 60 Enterobacteriaceae isolates, 13 urine isolates from female patients and three isolates from male patients were AmpC producers. Among them seven isolates were from patients within the age group of 20-30 years followed by three each from 0-10 years and above 50 years, two isolates from 30-40 years and one isolate from 10-20 years of age group. Cefoxitin resistance was documented in 23 (38.3%) isolates by the disc diffusion test. AmpC β-lactamase production was phenotypically confirmed in 12 (20%) and genotypically confirmed by PCR analysis in 16 (26.6%) of all the urine isolates. Genotypic AmpC-β-lactamase producers could further be assigned to the six genes as shown in [Table/Fig-4]. In the present study, 3 (13%), 2 (8.6%) of cefoxitin resistant isolates harboured the DHA, EBC gene and 1 (4.3%) each harboured FOX and CIT gene, and 9 (39.1%) harboured a combination of the genes. Notably, 17 (73.9%) of cefoxitin-resistant isolates contain Escherichia coli strain while the remaining samples consist of Klebsiella pneumoniae (n=5) and Proteus mirabilis strains (n=1). Multi-drug resistance is an important feature of cefoxitin resistant and AmpC-positive isolates.
Distribution of 16 AmpC-related genes among Enterobacteriaceae isolates.
Sample No. | Strain | FOX | EBC | ACC | DHA | CIT | MOX |
---|
1 | Klebsiella pneumoniae | + | - | - | - | + | - |
2 | Escherichia coli | + | - | + | + | - | - |
3 | Escherichia coli | - | - | - | + | - | - |
4 | Escherichia coli | + | - | - | - | + | - |
5 | Klebsiella pneumoniae | - | - | - | + | - | + |
6 | Escherichia coli | + | - | + | - | + | + |
7 | Escherichia coli | - | - | - | + | - | - |
8 | Escherichia coli | - | - | - | - | + | - |
9 | Klebsiella pneumoniae | - | + | - | - | - | - |
10 | Escherichia coli | + | + | - | - | - | - |
11 | Escherichia coli | - | + | - | - | - | - |
12 | Klebsiella pneumoniae | + | - | + | + | + | - |
13 | Escherichia coli | + | - | - | + | + | - |
14 | Escherichia coli | + | - | - | + | + | + |
15 | Escherichia coli | + | - | - | - | - | - |
16 | Klebsiella pneumoniae | - | - | - | + | - | - |
Antibiotic resistance analysis was carried out for several types of antibiotics, including ampicillin, amikacin, gentamicin, piperacillin/tazobactam, nitrofurantoin, norfloxacin, ciprofloxacin and cotrimoxazole as detailed in [Table/Fig-5]. The frequencies of cefoxitin-resistant and genotypically AmpC-positive isolates that are resistant to ampicillin and amikacin are same as compared to AmpC-negative isolates [Table/Fig-5]. Furthermore, while multiple-drug-resistance was observed in both genotypically AmpC-positive isolates and AmpC-negative isolates, the frequency of genotypically AmpC-positive isolates 7 (43.7%) that are resistant to 3 or more antibiotics is higher than that of AmpC-negative isolates 14 (31.8%) [Table/Fig-6a,b].
Antibiotic resistance pattern of cefoxitin resistant and AmpC-producing genotypic isolates.
Antibiotic type | Cefoxitin resistant strains (n=23) n (%) | AmpC producing strains (n=16) n (%) | AmpC negative strains (n=44) n (%) |
---|
Ampicillin | 23 (100) | 16 (100) | 44 (100) |
Amikacin | 0 | 0 | 0 |
Gentamicin | 1 (4.3) | 0 | 0 |
Piperacillin/Tazobactam | 1 (4.3) | 1 (6.25) | 0 |
Nitrofurantoin | 9 (39) | 7 (43) | 8 (18) |
Norfloxacin | 3 (13) | 2 (12) | 16 (36) |
Ciprofloxacin | 9 (39) | 6 (37) | 14 (31) |
Cotrimoxazole | 7 (30) | 3 (18) | 13 (30) |
Prevalence of multi-drug-resistance among Enterobacteriaceae. Frequencies of clinical isolates that is resistant to 3 or more antibiotics in genotypic AmpC β-lactamase (a) producers and (b) nonproducers.
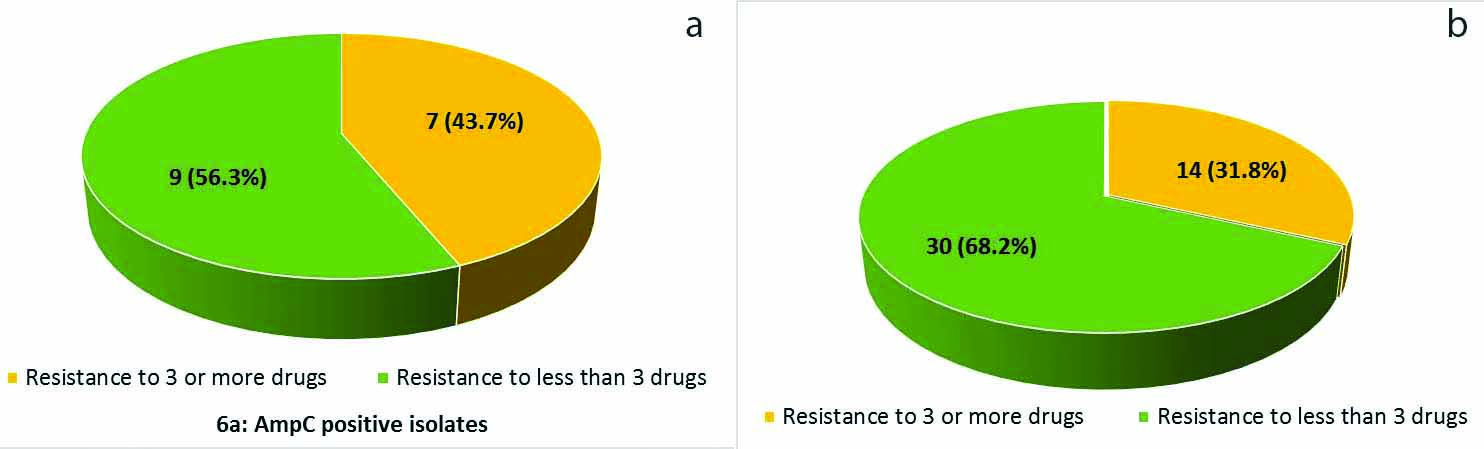
Discussion
Resistance to broad spectrum β-lactam antibiotics in Enterobacteriaceae, notably Escherichia coli, Klebsiella pneumoniae, and Proteus mirabilis, and Enterobacter aerogenes, has been increasing worldwide. Even though there are currently no CLSI guidelines for AmpC detection, reduced cefoxitin susceptibility has been used as an indicator for AmpC producers among clinical isolates [6].
In the present study, 13 isolates from female patients and three isolates from male patients were AmpC producers. Among them seven isolates were from patients within the age group of 20-30 years followed by three each from 0-10 years and above 50 years, two isolates from 30-40 years and one isolate from 10-20 years of age group. Similar results were also reported by Aryal SC et al., where they reported (51.6%) were females and (49.4%) of male patients bacterial isolates were AmpC producers [11]. Another study by Noor-ul-Ain J EH et al., reported that E.coli AmpC β-lactamase producers isolated from male and female patients were 52 (61.2%) and 33 (38.8%), respectively [12]. A 23 (38.3%) of all the isolates in the present study are cefoxitin resistant. A high prevalence of cefoxitin resistance (77.5%) has been documented by Mohamudha P et al., [13]. Manoharan A et al., reported 34.3% of gram-negative isolates cefoxitin resistant [5]. Differentiating cefoxitin resistant AmpC producers from cefoxitin resistant non AmpC producers is very important for therapeutic options as cefoxitin resistant AmpC producers can be treated with carbapenems and nonAmpC producers can be treated with extended spectrum cephalosporins [13]. Thus, distinguishing these organisms would further prevent unnecessary usage of carbapenems and cephalosporins. The reduced susceptibility to cefoxitin among Enterobacteriaceae serves as an important alarm for the spread of plasmid derived ampC genes. Cefoxitin resistance in AmpC nonproducers may be due to some other antibiotic resistance mechanism such as, lack of porins permeability [14].
Prevalence of AmpC producers among Enterobacteriaceae have been reported with great heterogeneity globally. This study revealed that 16 (26.6%) of the uropathogens are genotypically AmpC positive. The prevalence of AmpC genes among Enterobacteriaceae had been reported in the United States, Korea, China, India with ranges between (1.2%) and (2.79%) [15]. A study from Nepal reported the prevalence of these strains to be (27.8%) [16]. In India, Nakaye M et al., reported the prevalence to be (39.6%) [17]. This study also showed that 17 (73.9%) of Escherichia coli, 5 (21.7%) of Klebsiella pneumoniae and 1 (4.3%) of Proteus mirabilis cefoxitin resistant isolates exhibited AmpC production. In contrast, Baral P et al., reported the presence of AmpC producers in 59.7% of Escherichia coli and none of Klebsiella pneumoniae and Proteus mirabilis samples were AmpC producers [16]. Additionally, Manoharan A et al. reported frequencies of AmpC in Escherichia coli and Klebsiella pneumoniae are (43.7%) and (16.6%), respectively [5]. These diverse prevalence rates might result from differences in antimicrobial susceptibility as well as phenotypic or genotypic detection methods differences. More importantly, the frequency of clinical isolates that are resistant to three or more antibiotics is higher in AmpC producers than in nonproducers in this study. These findings are of epidemiological concern in supporting the occurrence and spread of MDR pathogenic bacteria.
With regard to diagnosis, the understanding about the prevalence and the molecular subtypes of plasmid derived AmpC in different geographical areas is censoriously important for an effective antimicrobial therapy and efficient infection control. The present study revealed that the prevalence of AmpC producers is 12 (20%) by inhibitor-based assay and 16 (26.6%) by PCR analysis. In Egypt, El-Hady SA and Adel LA, reported the prevalence of AmpC producers to be 50 (33.8%) out of 148 by phenotypic test and 46 (92%) out of 50 by multiplex PCR [18]. A study from Turkey reported (39.5%) and (8.7%) of the isolates are AmpC producers by phenotypic tests and molecular assay [19]. The discrepancy is due to the characteristic features of the selected isolates that is the geographical differences, the antimicrobial resistance phenotype of the bacterial isolates and the lack of specificity of phenotypic methods of AmpC detection [19]. Therefore, the molecular methods should be considered the gold standard method for AmpC β-lactamase detection [20].
In the present study, 3 (13%), 2 (8.6 %) of cefoxitin resistant isolates harboured the DHA, EBC gene and 1 (4.3%) each of FOX and CIT gene, and 9 (39.1%) harboured a combination of the genes. CMY followed by DHA are the most common plasmid mediated Amp C subtypes reported from different areas of the world [21]. A study from Iran revealed the presence of CMY predominance among Enterobacteriaceae isolates [22]. Another study from China reported DHA and ACT types predominance of AmpC producers [23]. There are limited reports on AmpC subtypes in Indian isolates [24]. Studies by Govindaswamy A et al., and Nakaye M et al., reported FOX gene as the predominant AmpC subtype [17,25]. In contrast to these studies, Varghese D et al., reported CIT, DHA and EBC predominance among AmpC producers [21]. Mohamudha P et al., reported plasmid Amp C genes in 38.1% isolates and the molecular subtypes were DHA, CIT followed by MOX and ACC types [13]. There are studies reporting expression of more than one plasmid mediated AmpC genotypes among the clinical isolates. In the present study, 9 (39.1%) of the cefoxitin resistant isolates harboured combination of the genes. A study by Nakaye M et al., reported 39.6% of the clinical isolates expressed a combination of AmpC subtypes [17]. The reason for this observation could be due to lack of specific phenotypic tests that can differentiate the AmpC subtypes and the limit of the bacterial pathogens to accommodate the subtypes.
Limitation(s)
Various ESBL genes and other possible resistance mechanisms such as Metallo Beta Lactamases (MBL) and efflux pumps were not investigated.
Conclusion(s)
The present study findings highlight the predominant existence of plasmid mediated AmpC producers in MDR Escherichia coli and Klebsiella pneumoniae. We suggest continuous meticulous surveillance and effective therapeutic intervention are important to effectively control the dissemination of these multidrug resistant strains and for optimal clinical outcome.