Endosseous dental implants is a popular treatment option in restorative and prosthodontic dentistry [1]. Successful implant therapy is not only a result of a well osseointegrated implant with beneficial effects on the bone and soft tissues. A large degree of success, especially in terms of aesthetics, quality of life, and patient satisfaction, depends on the connection between the implant and abutment, which is of great importance when it comes to long-term stability and the successful outcome of a restoration [2].
Implant-abutment connection is a critical area in the implant restoration. In two-stage implants, micro-gap between the fixture, the abutment and the superstructure are the potential areas wherein the bacteria can penetrate from the oral cavity, which can later cause peri-implantitis [3,4]. The sealing of the implant-abutment interface has been extensively investigated and remains as one of the most critical challenges to be overcome in long-term treatments with two-stage implants. Various in-vitro and in-vivo studies have reported the bacterial leakage along this interface constantly [5-8].
The bacterial flora around implants have been found to be similar to that of natural teeth, which predominantly include Streptococci, Veillonella species, Capnocytophaga species, Fusobacterium nucleatum etc. While, the bacteria associated with peri-implantitis are similar to those associated with periodontitis, which include Actinomyces species, Prevotella intermedia, Porphyromonas gingivalis etc., [4,6,9-11] Actinomyces species are considered as early colonisers, while Prevotella intermedia, and Prevotella gingivalis are considered as secondary colonisers on the surface of different prosthetic materials [4,6,10].
The adhesion and development of microbial biofilms depends not only upon the characteristics of the microbes which form it, but also on the host environmental conditions. Parameters like chemical composition, surface coatings, surface roughness, and surface free energy influence the adhesion of oral microorganisms to implant surfaces and the implant abutments [3,5,9,12-17].
The abutments are generally custom-designed/fabricated in a dental laboratory or prefabricated by the implant manufacturer. Various abutment materials are Titanium, Zirconia, Precious alloy, Surgical grade stainless steel and PEEK (Polyetherketone). The material composition also influences microbial adhesion affinity towards prosthetic surfaces. Zirconia has been introduced in implant dentistry as an alternative to Titanium materials mainly due to its aesthetic properties and potentially lower susceptibility to bacterial adhesion [5,18].
The elucidation of the mechanisms underlying bacterial adhesion, colonisation, and biofilm development on prosthetic devices and implant surfaces is currently an area of great interest in both clinical and biomedical research. Based on these findings, a possible approach to restrict biofilm formation involves the use of materials whose surface properties hinder biofilm development, particularly in the early stages of implantation [10,19].
A few studies have specifically highlighted the effect of the surface topography of dental implant abutment materials on surface bacterial interactions [3,5,13]. The most common failure associated with dental implant is peri-implantitis. The implant abutment is most susceptible for microbial adhesion because it is exposed to the various microflora in the oral cavity. The peri-implant microflora is mainly associated with aerobic and anaerobic microorganism [11].
The aim of this study was to evaluate the effect of surface characteristics like material composition, surface free energy and surface roughness of different implant abutment biomaterials on three commonly associated anaerobic microorganisms for peri-implantitis.
Materials and Methods
An analytical experimental in-vitro study was conducted in KAHER KLE VK Institute of Dental Sciences, Belagavi between January 2017-December 2017 Karnataka, India to evaluate the effect of surface characteristics of different implant abutment materials on the microbial adhesion. Three different implant abutment materials chosen were Titanium alloy, Surgical grade stainless steel and Zirconia. The selected abutment materials possess excellent mechanical properties, low colonisation potential and good biocompatibility. Ethical clearance was obtained from Institutional Ethical Committee (Certificate No 874 Dated on 30/10/2014).
Forty-five identical disc shaped specimens measuring 12 mm in diameter and 3 mm in thickness were fabricated using three different implant abutment materials and were divided into three sub-groups of n=15 each, namely; group A- Zirconia (n=15), group B- Titanium alloy (n=15) and group C- Surgical Grade Stainless Steel (n=15) [Table/Fig-1].
Disc shaped specimens among three groups.
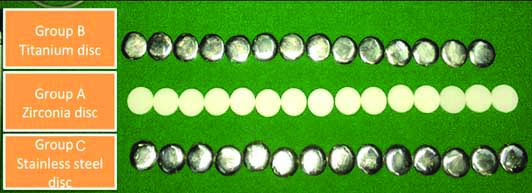
Inclusion criteria: Specimens with identical dimensions mentioned above were included in the study.
Exclusion criteria: specimens with surface defects and porosities were excluded from study.
Each titanium and stainless-steel disc specimen was precisely finished and polished using titanium and stainless-steel finishing and polishing kit (Metal polishing kit, Enkay Products Corp. Edgewood, N.Y-11717), respectively according to the manufacturer’s recommendations. Zirconia disc specimens were prepared from pre-sintered block of Yttrium-stabilised Tetragonal Zirconia Polycrystal (TZP) line material using a milling machine and finished and polished according to the manufacturer’s recommendations.
All the specimens from each sub-group were numbered from 1 to 15 (Group A: A-1 to A-15, Group B: B-1 to B-15, and Group C: C-1 to C-15), then cleaned with a steam cleaner under 0.3 Mpa pressure and then placed in ultrasonic cleaner (H&B Luxuries, Model No 8542028916, e-fortune pvt., ltd., India) for 180 seconds to remove any residues from their surfaces. All the specimens from each group were subjected to surface roughness evaluation quantitatively and qualitatively.
The quantitative measurements of Surface roughness of the specimens were carried out using contact stylus profilometer (Surtronic S-128-Taylor Hobson, Brasil) [Table/Fig-2].
Surface roughness evaluation of specimen using profilometer.
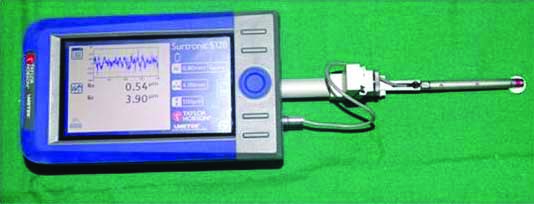
Each specimen was placed on a flat surface with surface to be tested facing upwards. The Profilometer determined the surface profile along three lines on the surface by means of a tracking device. The disc specimens were mounted and a diamond point-stylus was made to run with a transverse length of 4 mm and cut-off length of 0.8 mm and the arithmetical average value of all absolute distances of the roughness profile from the canter line within the measuring length were calculated. A total of five such readings were recorded for each specimen by a single operator. An average roughness profile of these five readings (Ra) was tabulated for each specimen to describe the overall roughness of the surface [14].
To evaluate the effects of polishing system on the disc surfaces qualitatively, specimens from each group were examined under field emission SEM; the SEM photographs were made with 550-fold and 800-fold magnification for better visualisation (SEM, JSM-6390, Jeol, Japan). One specimen from each sub-group was randomly selected and subjected to SEM. The surface topographic observations in all the specimens were tested qualitatively. These were useful in complimenting the observations by quantitative method used [Table/Fig-3].
Scanning Electron Microscopic (SEM) images of specimens in all three groups (550x800).
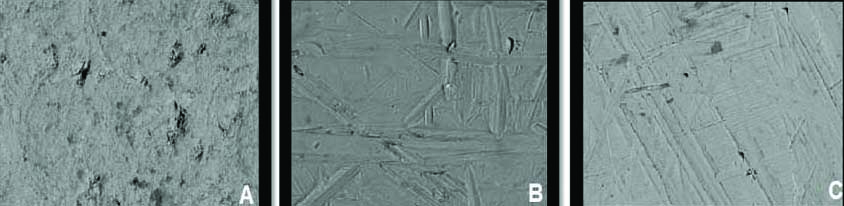
All the specimens were then cleaned with acetone and air-dried. The specimens from each sub-group were subjected to sessile drop method to evaluate surface free energy using Goniometer (Digidrop from GBX Instrumentation Scientifique, France). The Sessile Drop method was performed using distilled water (Rankem chemicals, Avantor, India) as the wetting agent to determine the WA for each disc specimen. Two microlitre single distilled water droplet was dispensed on the experimental disc surface with automatic syringe with a speed of 3 μL/sec. Two calibrated droplets were assessed on each specimen with two measurements of each droplet (right and left contact angle) precisely, till 15 seconds after careful deposition of the drop with a goniometer at room temperature by using vertical projection technique. The contact angles were calculated using the software Visiodrop (Surface Energy 2). Twenty images were taken for each droplet, with the help of which average WA was calculated for each specimen [Table/Fig-4].
Sessile drop method to evaluate the surface free energy of all the specimens using Goniometer.
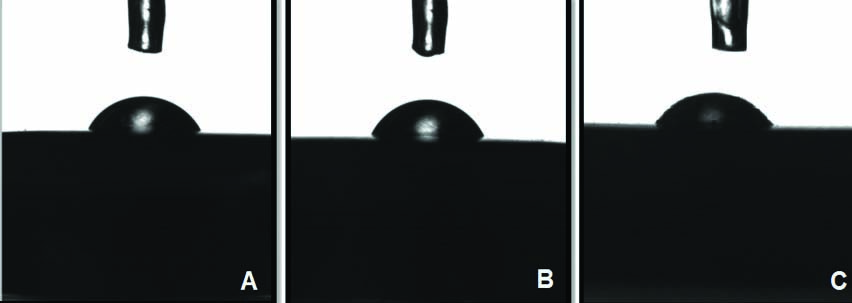
A mean WA was evaluated for each specimen to describe the overall surface energy of the surface [5].
After surface roughness and surface energy evaluation, all the specimens from each sub-group were subjected to microbial adhesion. Specimens to be subjected to the bacterial assays were sterilised in an autoclave (121°C for 15 min) (Romex, Niraj Sales Corporation, Delhi). Three bacterial strains were used to assess the adhesion properties of Gram-negative bacteria on the test materials namely; Porphyromonas gingivalis (Pg) strain ATCC 322, Prevotella intermedia (Pi) f strain ATCC 25611, and Actinobacillus actinomycetemcomitans (Aa) strain ATCC 33384 (HI Media Mumbai). These cultures were diluted in BHI (brain heart infusion, Hi Media, Mumbai, India) broth with horse serum to the desired concentration (Macferland Standard-1). To evaluate the adhesion, the discs were covered with a suspension of the bacterial culture and incubated at 37°C in an anaerobic jar for Pg and Pi and in CO2 jar for Aa for 48 hours [Table/Fig-5].
Specimens in the anaerobic incubator.
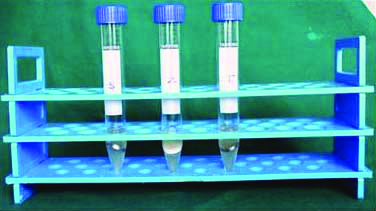
The samples were collected 48 hours later, the specimens were processed by washing them four times in Phosphate Buffer Saline (PBS) to remove unattached bacteria and then placing them in test tubes containing one ml of PBS. The tubes were submerged in an ultra-sonic water bath for three minutes, vigorously vortexed for one minutes, and then treated ultrasonically again for three minutes to release the surface-attached bacteria. Serial dilutions (101 to 106) of these suspensions were used to inoculate agar plates, which were incubated at 37°C in an anaerobic jar for Pg and Pi and in CO2 jar for Aa for 48 hours.
The colonies that had formed [Table/Fig-6] were then scored and counted by the colony counter (STUART, SC6PLUS, Arham Scientific Co, India). Colony-Forming Unit (CFU) count was calculated using the formula: CFU=No. of colonies×2n (n=Dilution factor) [9,10].
a) Colony Counter; b) Resultant Colonies (CFU) among three groups, groups A (Zirconia), B (Titanium) and group C (Stainless steel).
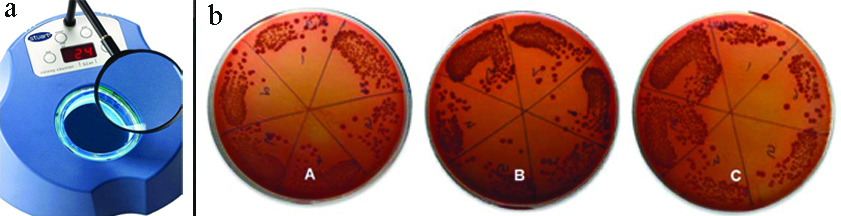
Statistical Analysis
The resultant values of three groups A, B and C (Zirconia, Titanium and Stainless steel) with respect to surface roughness and surface energy were subjected to statistical analysis to draw the conclusion from the experimental data. Descriptive statistical measures such as mean and standard deviation were computed for all the study groups. The data was analysed using SPSS software (Version 20). In order to collectively compare the means of the study groups, pair-wise comparison of the test group was done using paired t-test with (p<0.05), and correlation between the surface parameters and CFU counts was done using Karl Pearson’s correlation coefficient.
Results
[Table/Fig-7] showed that group A specimens showed the highest Ra values (0.4007±0.0299 μm), while group C specimens showed the least Ra values (0.3340±0.0223 μm). On pair-wise comparison of three groups (A, B, and C) with respect to surface roughness, there were statistically significant differences in surface roughness Ra values between all the groups.
Pair-wise comparison of three groups (Zirconia, Titanium and Stainless steel) with respect to surface roughness by using paired t-test. *p<0.05
Groups | Zirconia (n=15) | Titanium (n=15) | S.Steel (n=15) |
---|
Mean surface roughness (Ra) | 0.4007 | 0.3673 | 0.3340 |
SD | 0.0299 | 0.0464 | 0.0223 |
Zirconia | - | | |
Titanium | p=0.0293* | - | |
Steel | p=0.0001* | p=0.0293* | - |
[Table/Fig-8] showed that group C specimens showed the highest surface energy (WA) values (81.5213±2.1890), while group B specimens showed the least WA values (69.577±3.768). On pair-wise comparison of all the three groups with respect to surface energy, there were statistically significant differences in WA values between all the groups (p<0.001**) except between group B and A (p=0.15).
Pair-wise comparison of three groups (Zirconia, Titanium and Stainless steel) with respect to surface energy by using paired t-test. *p<0.05
Groups | Zirconia | Titanium | S.Steel |
---|
Mean surface energy | 72.3200 | 69.5767 | 81.5213 |
SD | 5.2749 | 3.7680 | 2.1890 |
Zirconia | - | | |
Titanium | p=0.1508 | - | |
S steel | p=0.0001* | p=0.0001* | - |
Results for pair-wise comparison of three groups (A, B, and C) with respect to CFU counts of Pg, Pi and Aa organisms are shown in [Table/Fig-9]. The mean CFU values were lower for group B (1610.67±1018.96) than the other two groups with respect to P.gingivalis; mean values were lower for group A (3272.53±1263.58) than the other two groups with respect to P. intermedia; and mean values were lower for group A (1634.13±716.30) than the other two groups with respect to Aa. The differences in CFU counts between the three groups (A, B, and C) were not statistically significant with respect microbial species.
Pair-wise comparison of three groups (Titanium, Stainless steel, and Zirconia) with respect to CFU counts of P.gingivalis, P.intermedia and A.actinomycetemcomitans organisms using paired t-test. *p<0.05
Organisms | Groups | Titanium | S.Steel | Zirconia |
---|
P.gingivalis | Mean CFU counts | 1610.667 | 2446.933 | 2553.6 |
SD | 1018.964 | 1227.725 | 962.3742 |
Titanium | - | | |
S Steel | p=0.0962 | - | |
Zirconia | p=0.0500 | p=0.9603 | - |
P.intermedia | Mean | 3622.40 | 3933.87 | 3272.53 |
SD | 1397.84 | 933.62 | 1263.58 |
Titanium | - | | |
S Steel | p=0.7634 | - | |
Zirconia | p=0.7117 | p=0.3052 | - |
A. actinomycet-emcomitans | Mean | 2016.00 | 2188.80 | 1634.13 |
SD | 845.17 | 1318.77 | 716.30 |
Titanium | - | | |
S Steel | p=0.8831 | - | |
Zirconia | p=0.5491 | p=0.2886 | - |
The results for correlation between surface roughness CFU counts, surface energy and CFU counts showed a strong positive correlation which was statistically highly significant for P.gingivalis (p<0.0001) P.intermedia (p<0.0001), and A.actinomycetemcomitans (p<0.0001) organisms in three groups (A, B, and C) as shown in [Table/Fig-10,11], respectively.
Correlation between surface roughness and CFU counts of P.gingivalis, P.intermedia and A.comitans organisms in three groups by Karl Pearson’s correlation coefficient; *p<0.05
Groups | Organism | Correlation between surface roughness and CFU counts |
---|
r-value | t-value | p-value |
---|
Titanium | P.gingivalis | 0.9211 | 8.5313 | ≤0.0001 |
P.intermedia | 0.9512 | 11.1186 | ≤0.0001 |
A.comitans | 0.9225 | 8.6183 | ≤0.0001 |
S Steel | P.gingivalis | 0.9139 | 8.1170 | ≤0.0001 |
P.intermedia | 0.9578 | 12.0082 | ≤0.0001 |
A.comitans | 0.8858 | 6.8837 | ≤0.0001 |
Zirconia | P.gingivalis | 0.8236 | 5.2362 | ≤0.0001 |
P.intermedia | 0.8887 | 6.9885 | ≤0.0001 |
A.comitans | 0.9440 | 10.3141 | ≤0.0001 |
Correlation between surface energy and CFU counts of P.gingivalis, P.intermedia and A. comitans organisms in three groups; *p<0.05
Groups | Organism | Correlation between surface energy and CFU counts |
---|
r-value | t-value | p-value |
---|
Titanium | P.gingivalis | 0.9080 | 7.8140 | ≤0.0001 |
P.intermedia | 0.9513 | 11.1288 | ≤0.0001 |
A.comitans | 0.9350 | 9.5051 | ≤0.0001 |
S Steel | P.gingivalis | 0.9155 | 8.2071 | ≤0.0001 |
P.intermedia | 0.9622 | 12.7384 | ≤0.0001 |
A.comitans | 0.9399 | 9.9219 | ≤0.0001 |
Zirconia | P.gingivalis | 0.8195 | 5.1554 | ≤0.0001 |
P.intermedia | 0.8987 | 7.3899 | ≤0.0001 |
A.comitans | 0.8539 | 5.9154 | ≤0.0001 |
There was statistically significant correlation observed between the values of surface roughness, surface energy and CFU counts in all the three groups with respect to all the microbial species (p<0.001**).
Discussion
Bacterial leakage at the implant-abutment interface can negatively affect the outcome of treatment and interfere with the long-term results. Physico-chemical characteristics such as surface roughness and surface energy play a major role in microbial adhesion process [3,7,13,14]. However, Quirynen M et al., Elter C et al., and Busscher HJ et al., demonstrated that surface roughness plays a predominant role in microbial adhesion out of the two parameters [13,20,21].
In the present study, quantitative and qualitative evaluation of all three abutment materials showed the mean average surface roughness (Ra) values within the range of 0.334 to 0.401 μm. Group A showed the highest Ra value, followed by group B and group C. Pair-wise comparisons of all the three groups with respect to surface roughness were statistically significant p<0.001**, The variability in Ra value in the present study can be attributed to different surface finish of the tested specimen, wherein, the titanium and stainless-steel discs were manually finished, and zirconia discs were machine finished. The difference in the material composition and grain-size of metals and zirconia samples could have attributed higher surface roughness values [22,23].
Rough surfaces promote plaque formation and maturation, while surfaces with high energy are known to select specific bacteria and adhere them more strongly [13]. The results obtained by goniometric evaluation showed that group C had the highest mean WA, followed by groups A and group B. The differences were statistically significant between groups A and C (p<0.001**) and between groups B and C (p<0.0001**). Changes in solid surface Ra below 0.1 μm have no effect on contact angle, and above 0.1 μm the effect depends on the initial contact angle as measured on a smooth surface. If the initial contact angle is below 600°, surface roughness will further decrease this angle; if the initial contact angle is above 860°, surface roughness will further increase this angle, and for surfaces with initial contact angles between 600° and 860°, surface roughness has no influence [13]. This suggested that the influence of surface roughness and surface free energy on the specimens in all three groups tested did not show any influence on the WA.
Listgarten MA and Lee A et al., in their study concluded that bacterial flora around implant is found similar to that of microbial flora around natural teeth [4,6]. There are various methods to assess and evaluate microbial adhesion on different prosthetic materials. Studies conducted by Nascimento CD et al., and Bundy KJ et al., had used fluorescence microscopy, Drake DR et al., had used SEM, and Rimondini L et al., had used molecular methods, to assess microbial adhesion [3,24-26].
Molecular methods such as DNA checkerboard hybridisation and Polymerase chain reaction have recently reported higher contamination indexes. Also, this method presents as the main drawback of reduced sensitivity, where the presence of microorganisms in a concentration lower than 104 cells does not result in detectable or reproducible results [5]. Evaluation by SEM and fluorescent microscopy is technique sensitive and the procedure is cumbersome [10,27-29]. Culture of peri-prosthetic tissue samples, which include Sonicating and Vortexing method, is a standard method to assess the microbiological adhesion on different prosthetic materials [30].
The present study used Sonicating and Vortexing method for microbial adhesion. The main advantage of this technique is its high accuracy and the simplicity of the procedure. The result obtained from this method is reliable, accurate, and reproducible [12]. The microbial adhesion was performed in strict anaerobic condition. This was in accordance with previous study conducted by Yamane K et al., where anaerobic bacteria were cultured in aerobic environment, which could have affected the results [11] or study conducted by Etxeberria M et al., in which aerobic organisms were used, which were least known to cause peri-implantitis [12]. The results of CFU counts of the present study demonstrated that group A (zirconia) showed the least mean CFU counts amongst all the groups with respect to Prevotella intermedia) and Aa. While group B (titanium) showed the least mean CFU count amongst all the groups with respect to Porphyromonas gingivalis). The experimental samples among all the groups with variable surface roughness and surface energy showed the least microbial adhesion towards Aa, followed by Pg and Pi.
Material composition also influences plaque accumulation and bacterial colonisation. Titanium and its alloys are used extensively in oral and orthopaedic implants due to their mechanical properties and biocompatibility with human tissues. There are, however, aesthetic problems associated with the titanium implant abutments due to their grey colouring. Zirconia has drawn attention as a potential alternative to titanium, as it allows avoidance of both allergic reactions and aesthetic. TZP, in particular, yttria-stabilised zirconia (Y-TZP) have been applied to the frameworks for fixed prostheses and dental implants as metal-free restorations offering not only higher strength, but also higher fracture toughness [18,26].
Surface roughness evaluation of a material depends upon various factors, which include material composition and grain size, method of fabrication/preparation, and instrument and technique used for measurement [21].
The correlation between different implant abutment materials and bacterial adhesion can also be explained by the antibacterial and bacteriostatic properties of prosthetic materials [31-33]. Zirconia samples showed the lowest CFU counts, which can be attributed to low colonisation potential of zirconia material [3,5,20,31-33].
Limitation(s)
The study had some limitations as only three different implant abutment materials were studied, inclusion of various other implant abutment materials might have shown different results group B and C displayed flat surfaces with random finishing pattern and low surface roughness values. On the other hand, group A showed more regular and arranged finishing pattern with overall rough surface. The difference could have arisen as the titanium and stainless-steel discs were manually finished and zirconia discs were machine finished.
Conclusion(s)
The results of the study concluded that Zirconia abutment material showed the lowest CFU counts as compared to other implant abutment materials with respect to P. intermedia and Aa. The material composition, surface roughness and surface energy of implant abutment materials play a significant role in microbial colonisation. Zirconia can be considered as a promising material for implant abutment compared to Titanium alloy and Surgical Grade Stainless steel. This study will help the clinician to choose a better implant abutment material, in terms of microbial affinity.