Human dental pulp represents an easily accessible source of stem cells for the purpose of cell based regenerative therapies [1]. DPSCs are found within the “Mesenchymal Stem Cells (MSCs) rich zone” of the dental pulp. Gronthos S et al., in 2000 isolated DPSCs from the pulp for the first time, and since then, a number of investigators have attempted to optimise the protocols for the isolation, establishment, culture expansion and maintenance of both research and clinical grade DPSCs [2-6]. In addition, a few attempts have also been made to evaluate the phenotype, growth characteristics and multilineage potential of DPSCs [7,8].
Notably, the production of clinically safe DPSCs in larger numbers requires the strict adherence to the compliance of therapeutic protocols. Under in-vitro cultivation of DPSCs, the standard isolation and expansion of these cells involve the usage of FBS as it provides binding factors, growth factors and a host of other nutrients essential for cell proliferation and maintenance. However, its inclusion poses many possible concerns for clinically relevant procedures as it is animal-derived and can cause transmission of animal pathogens and elicit xeno-immunological reactions against bovine origin antigens [9]. Further, the possible risk of contamination with mycoplasma, endotoxins and viral agents is also not ruled out with FBS. Hence, these critical issues compel the use of Xenogeneic-Free (XF) or human-origin alternatives to FBS for culture expansion and differentiation of DPSCs under in-vitro conditions [5,6,8]. Further, a chemically defined XF medium which is supplemented with variety of known essential growth factors reduce the dependence on animal derived sera [3,10]. Most of these chemically-defined XF or serum-free culture media are normally comprised of organic nutrients, such as, amino acids, carbohydrates, lipids and vitamins, inorganic nutrients, such as salts and trace elements, and non-nutrient factors, including hormone supplements, extracellular matrix proteins and growth factors to replace the components of FBS [3,4,7,8,10].
It is well understood that the major factor under in-vitro that has direct impact on the quality and consistency of stem cells is the culture medium composition [3,5,10]. In this direction, recent studies have employed the usage of xenogeneic serum-free culture media for enhancing the proliferation and multipotency of various types of dental stem cells [1,4,7,11]. However, additional studies are required to assess the cellular properties, stemness and lineage potential of DPSCs that may be helpful to determine the usefulness in regenerative medicine and tissue engineering application [5,6].
Key biological factors relevant to cellular therapy are self-renewal ability and potency. Although DPSCs possess transdifferentiation ability and broader plasticity [1], no definitive inference has been arrived on the expression patterns of OCT4, SOX2, NANOG and SSEA4 markers that are critical for the maintenance of self-renewal and pluripotency [1,12-14]. Therefore, the present in-vitro study evaluated the cellular characteristics and plasticity of DPSCs established and expanded in conventional serum-containing and serum-free media. The parameters analysed were the morphology, viability, proliferation rate, PDT, ALP activity, cell surface markers expression, osteogenic and adipogenic potential, and the relative abundance of pluripotent genes.
Materials and Methods
Isolation and Culture of DPSCs
This in-vitro descriptive study was conducted at NITTE (Deemed to be University), Mangaluru, Karnataka, India, from June 2019 to August 2020. Further, the study followed the Declaration of Helsinki on medical protocol and ethics, and was approved by the Central Ethics Committee and Institutional Committee for Stem Cell Research (NU/CEC/2017/0116B). The pulp tissues were acquired from impacted third molars of healthy donors (n=3) after obtaining an informed consent.
The tissues were separated and minced into ~2 mm fragments. Sliced tissue was subjected to 0.1% collagenase type II (Sigma-Aldrich, St. Louis, USA) enzymatic digestion for two hours at 37°C to release the cells from tissue and adhere to cell culture dish in alpha-Minimum Essential Medium (alpha-MEM, Gibco, Life Technologies, Grand Island, NY, USA) with 10% FBS, 2 mM glutamine and 100μg/ml penicillin/streptomycin (all from Gibco) and designated as serum containing medium-DPSCs (SCM-DPSCs). Another part of digested pulp tissue fragments were placed in a 35 mm culture dish with SFM (StemPro® MSC SFM XenoFree, Gibco, Life Technologies) and labeled as SFM-DPSCs. Cells were cultured at 37°C and 5% CO2 in a humidified atmosphere and maintained by renewing the medium every three days. Primary cells, thus obtained, were sub-cultured using 0.1% (w/v) trypsin-Ethylenediamine Tetraacetic Acid (EDTA) (Gibco) or nonenzymatic dissociation solution trypLE (Gibco) and made into pellets at 1200 rpm for five minutes. Cells were then re-grown and passaged 3-5 times for further analysis. During the culture, cells were observed for attachment and morphology under a phase-contrast microscope (Olympus, Tokyo, Japan).
Cell Viability Assay
Percentage of live cells was calculated at every passage of SCM- and SFM-DPSCs from Passage 1 (P1) to P4. Cell viability was assessed by 0.4% trypan blue (Gibco) staining using haemocytometer. Cells stained blue were considered as dead cells and transparent cells were counted as live cells [11].
Proliferation and Population Doubling Time (PDT) Analysis
Both DPSCs were plated at an initial density of 1.0×104 in 12-well plate (Thermo Fisher Scientific, USA) in triplicates. During the culture duration of 12 days, cells from each well at 3rd, 6th, 9th and 12th days were detached and counted with a haemocytometer. The culture medium was changed every three days. PDT of DPSCs was calculated using a formula; PDT=t (log2)/(log Nt-log No) where ‘t’ represents the culture time, and No and Nt are the cell numbers before and after seeding, respectively.
Analysis of Alkaline Phosphate (ALP) Activity
ALP activity of SCM- and SCF-DPSCs was assessed at Passage 3 (P3) by seeding the cells at a density of 3×104 in a 35-mm culture plate using BCIP/NBT kit (Promega, Madison, WI, USA) according to the manufacturers’ instructions. Cells were grown for 12 days with a change of fresh media every third day. The cells were fixed with 4% paraformaldehyde for 20 minutes and stained cells were observed under a light microscope.
Flow Cytometry Analysis of Cell Surface Markers
Both SCM- and SFM-DPSCs at 80% confluence were rinsed twice in DPBS and analysed for the expression of stem cell markers (CD29, CD73, and CD90) and the absence of CD34 and CD45 using flow cytometry (BD FACSCalibur, Becton Dickinson, NJ, USA). Cells were incubated in primary antibodies of unconjugated CD29 (eBioscience, CA, USA), CD73 (Biolegend, CA, USA), CD90 (eBioscience), CD34 (Biolegend) and CD45 (eBioscience) for 2 hours at 37°C. Fluorescein Isothiocyanate (FITC)-conjugated anti-mouse Immunoglobulin G (IgG) (eBioscience) was used as a secondary antibody and incubated for one hour at room temperature. Isotype-matched negative control was used (eBioscience). A total of 10,000 cells were acquired for each sample and analysed by a BD FACS Calibur with Cell Quest software (Becton Dickinson).
Osteogenic and Adipogenic Differentiation
SCM-DPSCs and SFM-DPSCs were independently induced to differentiate into osteogenic and adipogenic lineages. For osteogenic differentiation, cells at a density of 1.5×104 cells/well in a 12-well plate were cultured either in alpha-MEM and 10% FBS or StemPro® MSC SFM supplemented with 0.1 μM dexamethasone (Sigma-Aldrich), 10 mM β-glycerol phosphate (Sigma-Aldrich) and 0.2 mM ascorbic acid (Sigma-Aldrich) for 21 days. The medium was changed twice a week. Osteogenesis was assessed with Alizarin Red S (40 mM, pH 4.2, Sigma-Aldrich) staining method. Stained cells were observed using an inverted phase-contrast microscope (Olympus).
For adipogenesis, cells at a density of 1.5×104 cells/well in a 12-well plate were cultured either in alpha-MEM and 10% FBS or StemPro® MSC SFM supplemented with 0.5 mM 3-isobutyl-1-methylxanthine (Sigma-Aldrich), 1 μM dexamethasone (Sigma-Aldrich), 0.1 mM indomethacin (Sigma-Aldrich), and 10 μg/ml insulin (Sigma-Aldrich) for 21 days. Medium changes were carried out twice weekly. The presence of lipid droplets was evaluated by 0.5% w/v Oil Red O (Sigma-Aldrich) staining and imaged with an inverted phase-contrast microscope (Olympus).
Quantitative Real-Time Polymerase Chain Reaction (qRT-PCR) Analysis
qRT-PCR was performed to detect the expression levels of pluripotent markers in both SCM- and SFM-DPSCs. Total Ribonucleic Acid (RNA) from cultured cells was isolated with TRIzol (Invitrogen, Life Technologies) and cDNA was synthesised using a TaKaRa PrimeScript™ RT Reagent Kit (TaKaRa, Japan) according to the manufacturer’s instructions. The mRNA expression levels of target genes, such as OCT4, SOX2, NANOG and SSEA4 were quantified by real time PCR (Applied Biosystems, StepOnePlusTM) using SYBR® Premix Ex Taq TM II (Takara) according to the protocol provided with conditions: denaturation at 95°C for 5 minutes and 94°C for 30 seconds, annealing at 60°C for 30 seconds, elongation at 72°C for 90 seconds and final extension at 72°C for 10 minutes with 35 cycles. ΔΔCT method was used to evaluate relative mRNA expression of target genes, which was normalised against β-actin for every sample. The primer sequences for the analysed genes are shown in [Table/Fig-1].
Sequence of primers used in qRT-PCR.
Gene | Sense and anti-sense sequences (5’-3’) | Product size (bp) |
---|
OCT4 | GTGGAGGAAGCTGACAACAAATTCTCCAGGTTGCCTCTCA | 119 |
SOX2 | ACACCAATCCCATCCACACTGCAAACTTCCTGCAAAGCTC | 224 |
NANOG | CCTGTGATTTGTGGGCCTGGACAGTCTCCGTGTGAGGCAT | 77 |
SSEA4 | TGGACGGGCACAACTTCATCGGGCAGGTTCTTGGCACTCT | 118 |
β-ACTIN | TCCTTCCTGGGCATGGAGAGGAGGAGCAATGATCTTGATCTT | 207 |
Statistical Analysis
All experiments were performed in triplicates and the data are expressed as the mean±Standard Deviation (SD). Analysis of variance (ANOVA) was performed by GraphPad Prism software (GraphPad, CA, USA) with Tukey’s post-hoc test and the level of significance was tested at p-value <0.05.
Results
Morphology
The primary cultures of DPSCs were established in conventional SCM and SFM. In both culture conditions, cells were released from the enzyme digested dental pup and reached 80% confluence by day 15 in primary culture (P0). The adherent DPSCs showed more number of clusters in SFM with a small, spindle-shaped morphology, whereas, SCM-DPSCs exhibited a characteristic fibroblast-like morphology in culture [Table/Fig-2]. Further, no major morphologic differences were observed between SCM- and SFM-DPSCs after passage 1 (P1) and both types of cells later acquired relatively homogeneous morphology.
Establishment of primary culture and morphology of DPSCs. Images showing the establishment of primary culture of DPSCs (Passage 0- Day 2 and 5) in SCM and Serum-Free Medium (SFM). At passage 1, both DPSCs at sub-confluent stage exhibited fibroblast-like morphology.
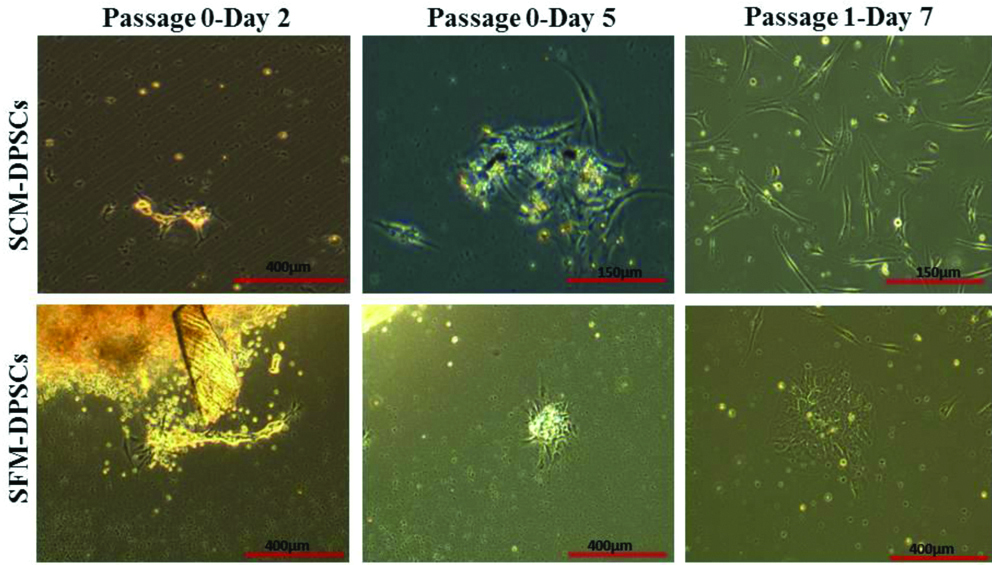
Viability
Viability of SCM- and SFM-DPSCs at different passages was determined and any changes in viability during culture expansion were also recorded. Except at P1, no significant (p-value >0.05) differences in percentage viability between SCM-DPSCs and SFM-DPSCs were observed [Table/Fig-3a]. Cell viability was found more than 90% at passages from P2 to P4, whereas at P1, the values recorded were 88% and 82.66% for SCM-DPSCs and SFM-DPSCs, respectively.
Viability, proliferation, PDT and ALP activity of DPSCs in SCM and Serum-Free Medium (SFM). a) Percentage of cell viability at different passages from P1 to P4. * (p-value=0.05). b) Proliferation rate from day 0 to day 12 at different time points. a and b) (p-value <0.05). c) PDT in hours. *(p-value <0.05). d and e) Expression of ALP in SCM-DPSCs and SFM-DPSCs, respectively.
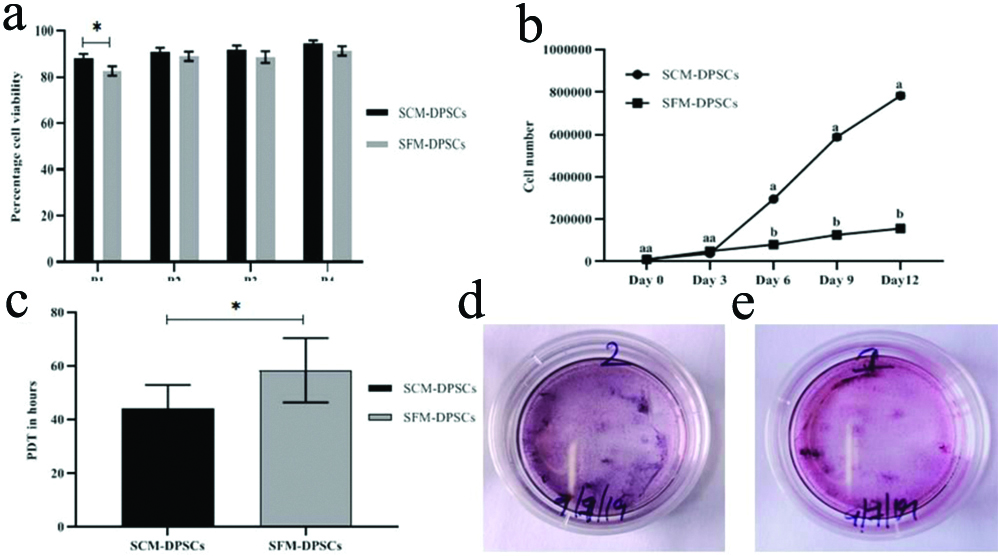
Proliferation Rate and PDT
Cell proliferation assay was performed at P3 of both types of DPSCs by counting the cells at 0, 3, 6, 9 and 12 days using a haemocytometer. The results of proliferation rate and PDT are represented in [Table/Fig-3b,c], respectively. There was a significant (p-value<0.05) difference in the proliferation rates between SCM-DPSCs and SFM-DPSCs from day 6 onwards, and the cell density on day 12 was higher in SCM-DPSCs than in SFM-DPSCs (Day 6: 3×105 vs 0.8×105; Day 9: 5.8×105 vs 1.27×105; Day 12: 7.8×105 vs 1.56×105, respectively). While comparing the expansion ability of cells, SCM showed a higher average fold increase than in the SFM. In terms of PDT values, a significant (p-value<0.05) difference was observed between SCM-DPSCs and SFM-DPSCs. The average PDT values of 44.33 hours and 58.41 hours were recorded for SCM-DPSCs and SFM-DPSCs, respectively.
ALP Activity
ALP assay was performed for both types of DPSCs at P4, and the results clearly indicated a positive dark purple staining in a 35 mm dishes cultured with an initial seeding density of 3×104/dish [Table/Fig-3d,e]. The results showed that a slightly higher expression of ALP activity was observed in SCM-DPSCs than in SFM-DPSCs.
Cell Surface Markers Expression
Flow cytometry analysis showed that DPSCs expanded in SCM and SFM at P3 exhibited positive expression for Mesenchymal Stem Cells (MSCs)-specific markers (CD29, CD73 and CD90), and negative expression for haematopoietic cell markers (CD34 and CD45) [Table/Fig-4]. However, the phenotypic profile of DPSCs cultured in SCM had the higher expression of MSC-positive markers, CD29 (97.27%), CD73 (90.81%) and CD90 (86.89%) than the DPSCs cultured in SFM with CD29 (65.61%), CD73 (69.50%), and CD90 (75.16%).
Cell surface markers expression in DPSCs in Serum-Containing Medium (SCM) and Serum-Free Medium (SFM) by flow cytometry. The histograms show the percentage expression of markers as indicated.
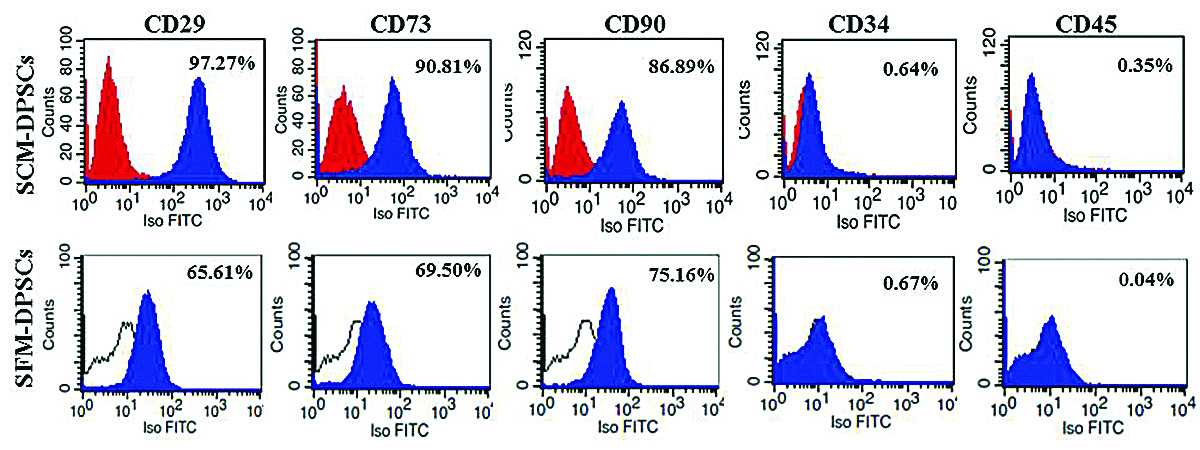
Osteogenic and Adipogenic Differentiation
Both types of DPSCs displayed typical mesenchymal differentiation ability along the osteogenic and adipogenic lineages [Table/Fig-5,6]. Alizarin red staining of induced cells revealed the deposition of mineralised matrix. The accumulation of lipid droplets was confirmed by Oil red O staining [Table/Fig-5b,d]. However, a slightly less intense calcium deposition and lipid deposition was noticed in SFM-DPSCs compared to SCM-DPSCs. In control, no osteogenic or adipogenic induction was observed [Table/Fig-5a,c,6a,c].
Osteogenic differentiation ability of DPSCs cultured in Serum-Containing Medium (SCM) and Serum-Free Medium (SFM). The deposition of mineralised matrix was demonstrated by Alizarin red staining (arrows). No osteogenic induction was observed in control.
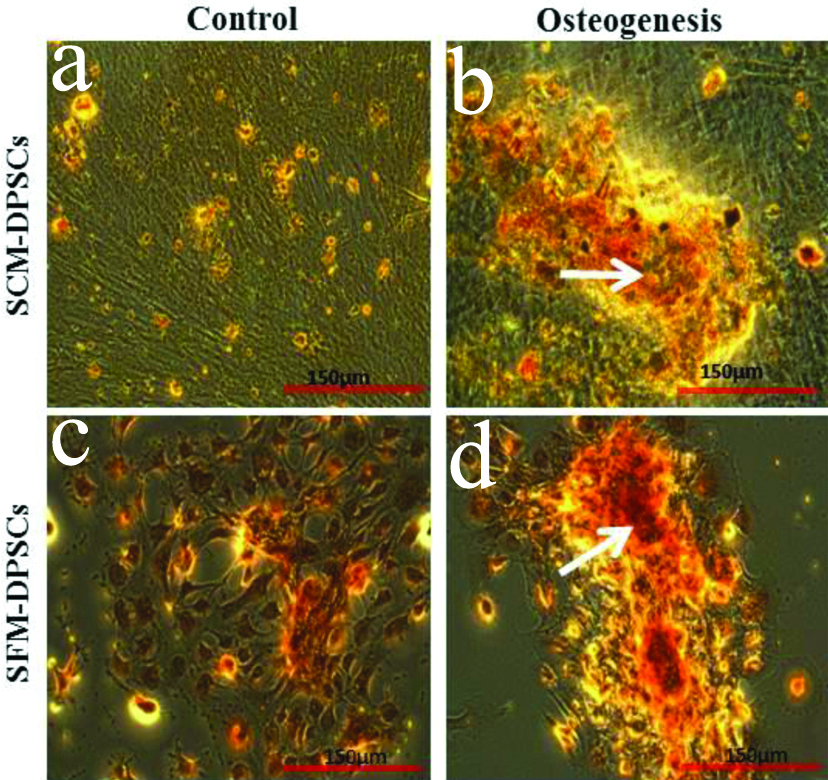
Adipogenic differentiation ability of DPSCs cultured in Serum-Containing Medium (SCM) and Serum-Free Medium (SFM). The accumulation of lipid droplets was showed by Oil red O staining (arrows). No adipogenic induction was observed in control.
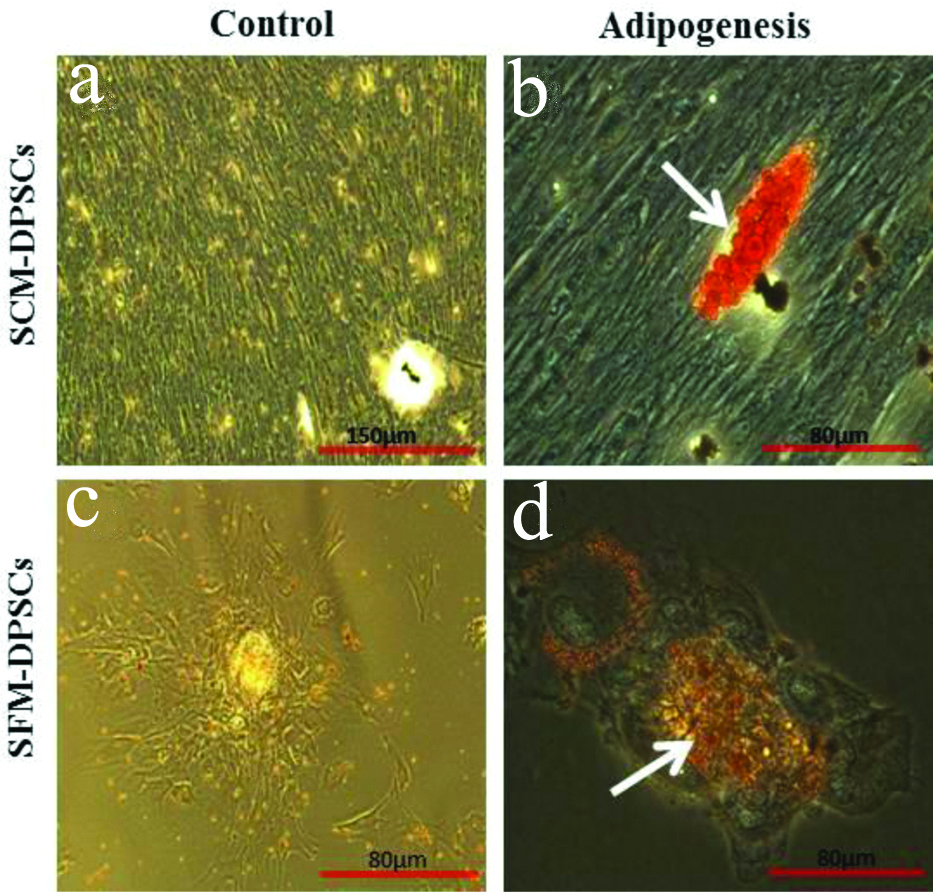
Expression of Pluripotent Markers
The expression of pluripotent stem cell markers (OCT4, SOX2, NANOG and SSEA4) was analysed in DPSCs cultured separately in SCM and SFM by qRT-PCR. In this method, the relative expression of stem cell marker genes was normalised against β-actin as a reference gene for each sample using specific oligonucleotide primers. The results showed a significantly (p-value <0.05) higher relative expression of OCT4 and NANOG in SCM-DPSCs compared to SFM-DPSCs [Table/Fig-7]. Further, though not statistically significant (p-value>0.05), there was a higher relative expression of SOX2 and SSEA4 in SCM-DPSCs than in SFM-DPSCs.
Expression levels of genes related to pluripotency in DPSCs cultured in Serum-Containing Medium (SCM) and Serum-Free Medium (SFM) analysed by qRT-PCR. (*p-value<0.05). The expression of target genes was normalised to that of reference gene, β-actin.
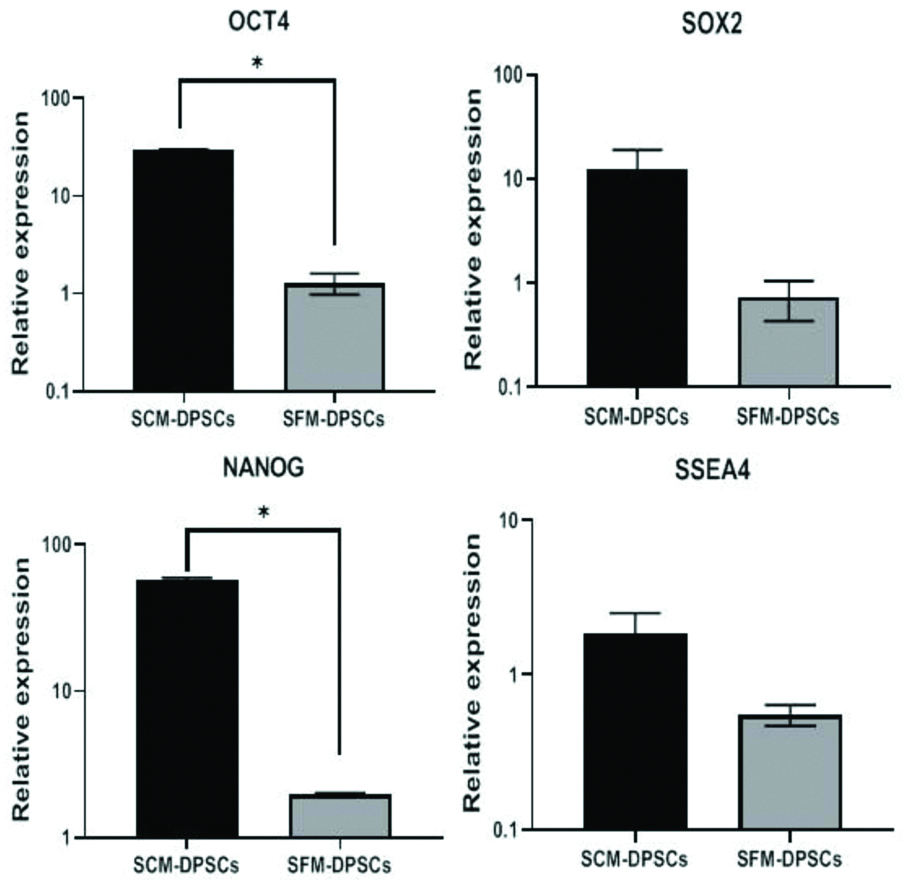
Discussion
The generation of large numbers of DPSCs for cellular therapies requires their culture establishment and expansion in Xenogeneic-Free (XF) or Serum-Free Medium (SFM) conditions. A few studies have already used the xenogeneic serum-free culture media for assessing the proliferation and potency of various types of MSCs [1]. As the efforts are ongoing to develop effective serum-free cell culture media, an understanding of potential influence on cellular and potency properties was necessitated for the eventual production of clinical-grade DPSCs in order to fulfill the compliance.
In the present study, DPSCs were successfully isolated from impacted third molars. Authors followed the partial enzyme digestion in collagenase followed by explant culture, and obtained sufficient numbers of DPSCs. Till date, no isolation technique has been proved superior to others based on the proliferative ability and clinical use of DPSCs. Earlier studies suggest that both the enzymatic digestion and outgrowth or explant method could be applied to generate DPSCs with no major differences [15,16].
The attached DPSCs exhibited typical fibroblast-like morphology along with small, spindle-shaped cells when cultured in SCM and SFM. Cells were observed as a homogenous population with no noticeable differences in morphology. Similarly, SFM-DPSCs showed a thin, spindle-shaped morphology with longer processes as compared with SCM-DPSCs [7]. However, in another study, DPSCs in serum-free conditions revealed neuronal-like cells [8]. Further, both DPSCs showed >80% viability at passage 1 (P1) to P4. Except at P1, no significant (p-value >0.05) differences in percentage viability between SCM-DPSCs and SFM-DPSCs were observed. The results showed that SFM supported significant numbers of viable DPSCs in culture.
Following cell proliferation assay, the highest cell number was recorded on day 12 in SFM-DPSCs and showed significant (p-value <0.05) increase than in SCM-DPSCs. Further, the PDT values were in supportive of proliferation rates. In previous reports, higher levels of proliferation were observed with DPSCs cultured in XF or SFM [3,7]. Particularly, a cocktail of basic fibroblast growth factor, insulin-transferring-selenium, vitamin C, beta-mercaptoethanol and cholesterol as a supplement to XF/SFM showed a significant improvisation of DPSCs along with enhancement of stemness properties [4]. The reason for the differences in growth pattern of SFM- and SCM-DPSCs could be related to the composition of the media manufactured for multi-source MSCs-expansion [13].
Identification of phenotypic markers in DPSCs is a pre quisite for the selection of appropriate cell population [5]. In this study, flow cytometry data of both DPSCs indicated the presence of MSCs markers, such as CD29, CD73 and CD90, and the absence of hematopoietic markers, such as CD34 and CD45. Expanded DPSCs did not display significant differences in terms of the phenotypic markers expression as proposed by ISCT [17]. Considering that DPSCs largely share a phenotypic profile of MSCs, the expression levels are in accordance with previous reports on DPSCs [4-6,8,16]. However, the expression levels of positive markers were higher in SCM compared to SFM-DPSCs. This observation is in contrast with the higher expression in DPSCs grown with serum-free culture system [3]. The marker expression patterns in DPSCs differ among the studies and high diversity of antigen expression has been demonstrated [5,16]. Further, DPSCs are known to possess ALP activity, which is considered as a marker for undifferentiated stem cells as well as a marker of osteogenic/odontogenic cells. Our present results showed a slightly higher expression of ALP activity in SCM-DPSCs than in SFM-DPSCs. These variations suggested that there is an influence of cell culture conditions and media composition as observed in this study.
The osteogenic induction of DPSCs in FBS and SFM stimulated the formation calcium producing cells on the tightly packed monolayer over induction time. The long-term cultures resulting in the formation of bone nodules with calcium phosphate deposits by staining with von Kossa and/or Alizarin red S solutions indicated the osteogenic/odontogenic differentiation of DPSCs in FBS [5,18,19]. In this study, osteogenesis based on extracellular matrix mineralisation was observed by dark brown nodule formation of calcium with Alizarin red staining in DPSCs cultured in FBS and SFM with no noticeable differences. Similarly, the accumulation of lipid droplets following adipogenic induction was confirmed by Oil red O staining. However, a slightly less intense calcium deposition and lipid deposition was observed in SFM-DPSCs compared to SCM-DPSCs. DPSCs cultured in E8 as a chemically defined SF medium induced to form osteocytes and adipocytes [3,7]. However, in an earlier study, XF/SFM failed to induce any osteogenic differentiation of DPSCs, but did result in adipogenesis [13]. The present study observations along with earlier results indicate that, similar to cell proliferation and phenotypes, the lineage differentiation was dependent on the supplements in the culture medium used.
The pluripotent markers such as OCT4, SOX2, NANOG and SSEA4 have been known as key regulators of self-renewal and differentiation process of pluripotent stem cells. The importance of these markers in generating and preserving the potency of cells has necessitated to be considered as stemness markers. In this study, qRT-PCR results showed a relatively higher expression of OCT4 and NANOG in SCM-DPSCs compared to SFM-DPSCs. Further, SCM-DPSCs showed the higher levels of SOX2 and SSEA4, but did not exhibit any significant differences in their expression levels. These results indicated the presence of stem cells representing the status of both pluripotency and multipotency.
Supporting this, earlier report suggested that OCT4 and NANOG signaling acts as a regulatory switch to maintain properties in DPSCs [14]. The expression of all stemness markers revealed the maintenance of self-renewal and ability for lineage differentiation in DPSCs regardless of the presence or absence of serum in the culture media [13]. Further, the presence of stemness markers in the lineage restricted adult tissue, such as dental pulp indicates their likely role in the regeneration of damaged cells or tissue. Collectively, all these observations suggest that DPSCs expanded in FBS and SFM retained their proliferation ability, plasticity and ability to undergo directed differentiation into specific lineage cells. Hence, the SFM can be used for ex-vivo expansion of DPSCs for potential clinical applications.
Limitation(s)
The present study qualitatively assessed the osteogenic and adipogenic differentiation of DPSCs in SCM and SFM conditions, and requires quantitative evaluation in future studies.
Conclusion(s)
The present in-vitro study showed that SFM can be used to establish and expand DPSCs with characteristic features of multipotent stem cells. However, DPSCs in FBS displayed greater proliferation ability and higher cell surface and stemness markers expression along with more propensities towards lineage differentiation. Therefore, further research on the optimisation of serum-free culture conditions is necessary for translating into clinical applications.