An ideal root-end filling material should exhibit a number of properties, which includes sufficient radiopacity that can be distinguished from the surrounding structures [1]. The ISO-6876 specification for dental root canal sealing materials states that the radiopacity should be greater than that of 3-mm thick aluminum. Achieving this level of radiopacity can pose a challenge, mainly due to the small amounts of materials used. Radiodensity of dental materials is a physical property used to assess the position and location of dental materials, which is important for re-treatment.
Sometimes detecting biomaterials, particularly in low volume, is difficult. This impedes the decision-making process. In some clinical situations, including apicoectomy, (Cvek) pulpotomy and apexification, the volume of biomaterial used is low and may not be detected by x-ray; leading to treatment plan failure. It should be noted that radiopacifiers normally do not contribute to biomaterial chemical reactions. However, some studies show that the radiopacifiers’ particle size and distribution may affect physical properties [2,3]. Unfortunately, some radiopacifiers have drawbacks such as discolouring the surrounding tissues [4] and compromising the mechanical properties [5].
Bismuth oxide is a radiopacifier used in MTA, which interferes with the hydration mechanism of MTA [6]. The bismuth forms part of the structure of calcium silicate hydrate, replacing the silica in its structure. During the hydration process, approximately 5 wt% of bismuth attaches to calcium silicate hydrate structure [7]. This radiopacifier disrupts calcium hydroxide precipitation in hydrated paste and leaches out from the material along with calcium hydroxide [6,8].
Zirconium oxide is another radiopacifier which is widely used in dental cements. This commonly known material is a polymorph and consists of a tetragonal (T), cubic (C), and monoclinic (M) form which is stable at room temperature up to 1170°C [9].
The aim of this study was to evaluate the radiodensity of different commonly used endodontic biomaterials with different thicknesses and to determine the impact of different thicknesses on the physical parameters such as particle size of the radiopacifier and radiodensity.
Materials and Methods
Sample Selection and Preparation
This was an in-vitro study conducted between August 2018 to December 2019 in Rutgers biomaterials Lab at Rutgers School of Dental Medicine. The present research did not include any involvement of human subjects and it is based on Rutgers School Policy (https://orra.rutgers.edu/irb-review). So, the need of any approval from Institutional Review Board (IRB) was not needed.
Four rows of holes measuring 0.1 mm, 0.2 mm, 0.5 mm and 1 mm in depth were carved in a “Plexiglas” sheet (Ridout Plastics Company Inc., San Diego, CA) by using Gates-Glidden drills (Union Broach Corp, NY). Three plates with six columns were carved. Therefore, for each depth there were 3 holes in separate plates.
For the first part of the study, endodontic biomaterials (AH26 sealer (DeTrey Dentsply), EndoSequence BC sealer (Brasseler USA, Savannah, GA), Endoseal MTA (EndoSeal, Maruchi, Seoul, Korea), Nano-MTA, Endocem Zr (Maruchi, Wonju, Korea), and MTA without radiopacifier as a control group) were selected and mixed and condensed into the holes. The plates were stored overnight in an incubator. Seventy-two holes were packed and prepared radiographically.
For the second part, based on the results of the first part, two radiopacifiers (bismuth oxide and zirconium dioxide) were selected. For each radiopacifier three different particle sizes were selected and categorised as coarse, thin and fine. MTA (Dentsply Tulsa Dental Specialties, Tulsa, OK) without bismuth oxide was provided. Bismuth oxide and zirconium dioxide were added to the MTA as the following groups. A single operator weighed and mixed the samples at 23±2°C in the laboratory with water at 3:1 ratio and placed them into the holes. The plates were incubated over night at 37°C. After the incubation period, the plates underwent digital radiography.
All the Calcium-Silicate Cements (CSCs) were White Mineral Trioxide Aggregate (WMTA), manufactured by Dentsply (Tooth-coloured Formula, ProRoot MTA) with the same composition without bismuth oxide. A range including 10 μm, 200 μm, 120 nm sized particle of 20 wt% bismuth oxide was found in groups 1-3.
Zirconium dioxide was used for the fourth, fifth and sixth groups with 5 μm (Sigma-Aldrich 230693 CAS Number: 1314-23-4), 1 μm (Noah Technologies 11763 CAS 1314-23-4), and 20-40 nm (Alibaba, Brand: XFNANO Model: XFI01-1) particle sizes as shown in [Table/Fig-1]. For group 7, only pure MTA without radiopacifier was used as a control group.
The Plexiglas which was printed in 3D for making X-rays. a) Radiograph of the Plexiglas with holes and different depths; b) A radiograph of a single hole; c) Nominalising the images and making them binary; d) Calculating the histograms and intensity; e) The head of the X-ray machine; f) X-ray machine panel; g) Plexiglas filled with various endodontic biomaterials with different depths; h) Plexiglas loaded with two different radiopacifiers with different particle sizes.
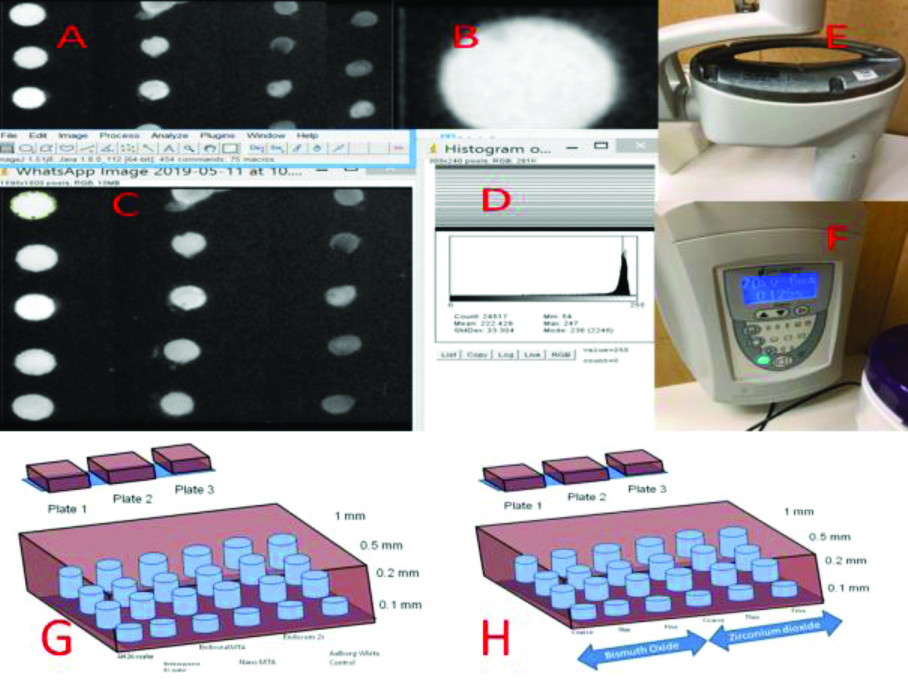
Evaluation of Radiopacity
This part was performed according to the method prescribed by the ISO 6876:2012 for dental root canal sealing materials and similar to the method used by Saghiri MA et al., [3]. Briefly, each Plexiglas was prepared and fixed with a transparent plastic tape. From each cement, six samples where gathered. Then each sample were mixed with water and set at 37°C with 98% humidity for 24 hours.
The part of incubation is similar to the previous study [10]. Briefly, the samples were stored in distilled water at 37°C for seven days after removal from the moulds. The radiographs were performed using a dental X-ray unit (MPS, Progeny Dental, Buffalo Grove, USA) at 70 kVp, 6 mA and 0.125s exposure time with a digital sensor (MPS, Progeny Dental, Buffalo Grove, USA). The selected exposure parameters (i.e., kVp, mA and exposure time) were based on a pilot exposure to detect all the materials at the lowest thickness by x-ray.
Image Preparation, Assessment and Acquisition
Grey values of the step wedge were measured using Image J software (National Institutes of Health, Bethesda, MD, USA) to standardise image brightness and contrast as proposed by Saghiri MA et al., [11]. Grey scale was measured by histogram of Image J. Digital images were recorded using Microsoft Picture Manager (Microsoft, Redmond, WA) to standardise each picture at 480X666 pixels.
Then, radiodensity was calculated with Image J program (Rasband WS, ImageJ; US National Institute of Health, Bethesda, MD) with a single operator [Table/Fig-1a-h]. Each figure was selected and the Region Of Interest (ROI) was selected. manually by operator around the material plug [Table/Fig-1b]. The histogram was measured and radiodensity for the area was determined [Table/Fig-1d]; the mean intensity for radiodensity was exported as indicator for radiodensity power of cement. The radiodensity for different thicknesses and materials were exported to an excel file.
Power Analysis
Due to the absence of any supporting data for sample size calculation for the first part of the research, we collected a pilot sample of 72 to provide three specimens for each combination of the thickness (four levels) and material (six levels). A factorial design with two factors achieved 100% power at a 5% significance level.
In the second part, 84 specimens were collected to provide three samples for each combination of the thickness (four levels) and material (seven levels). A factorial design with two factors achieved 100% power at a 5% significance level.
Statistical Analysis
Descriptive statistics were calculated and presented. General linear models were used to determine whether the thicknesses and materials affected the radiodensity for both parts of the study. Two-way interaction ANOVA Test was included in the model. Post-hoc Tukey test was used for multiple comparisons p<0.05 was considered statistically significant. IBM Statistical Package for the Social Sciences (SPSS) Statistics 25 software was used for data analysis. Significance level (2-sided test) was set at 0.05 for all the tests.
Results
The mean radiodensity value of the materials with different thicknesses and the mean radiodensity value of samples after adding radiopacifiers with different sizes and different thicknesses is depicted in [Table/Fig-2,3].
The mean radiodensity value of the materials with different thicknesses.
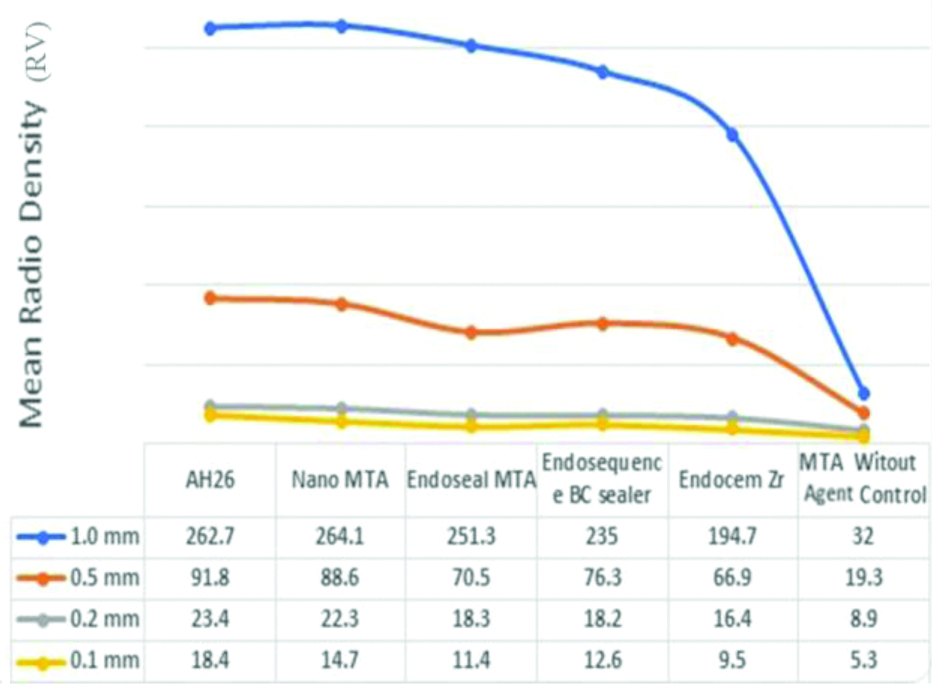
The mean radiodensity value of samples after adding radiopacifiers with different sizes and different thicknesses.
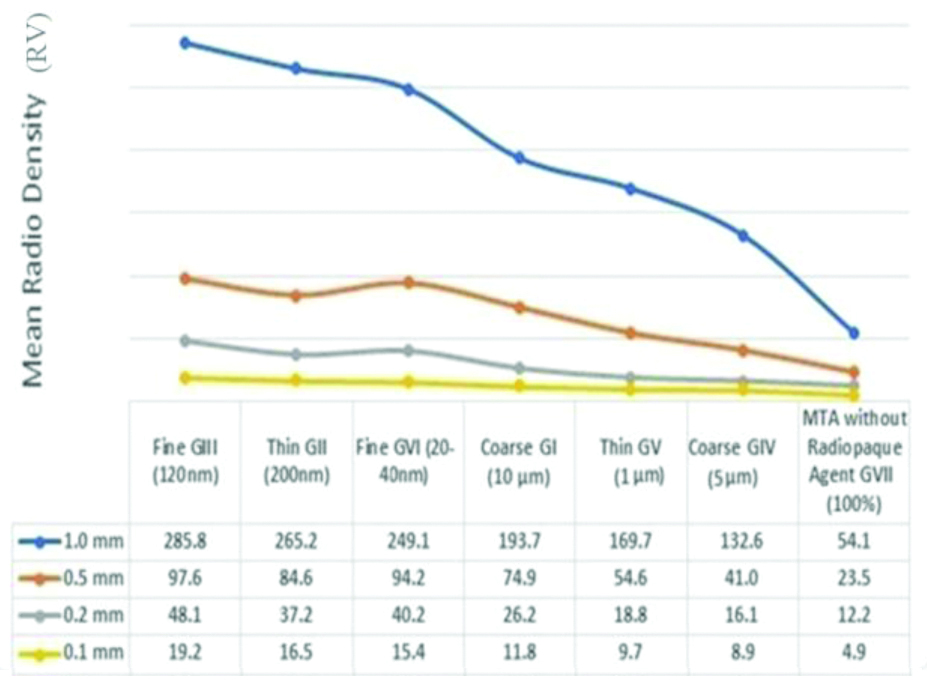
The radiopacifiers with different particle sizes used in the current study are shown in [Table/Fig-4].
Radiopacifiers with different particle sizes used in the current study.
Group number | Radiopaque agent | Particle size | Supplier |
---|
G I | Bismuth oxide (coarse) | 10 μm | Sigma-Aldrich |
G II | Bismuth oxide (thin) | 200 nm | Inframat Advanced Materials |
G III | Bismuth oxide (fine) | 120 nm | US Research Nanomaterials Inc. |
G IV | Zirconium oxide (coarse) | 5 μm | Sigma-Aldrich |
G V | Zirconium oxide (thin) | 1 μm | Noah Technologies |
G VI | Zirconium oxide (fine) | 20-40 nm | Alibaba |
G VII | MTA without radiopacifier (100%) | N/A | ProRoot MTA |
The mean and standard deviation of radiodensity for six endodontic biomaterials (AH26 sealer, EndoSequence BC sealer, Endoseal MTA, Nano-MTA, Endocem Zr and Aalborg White Control) and four different thicknesses of materials (0.1 mm, 0.2 mm, 0.5 mm and 1 mm) are shown in [Table/Fig-5]. The general linear model showed significant differences in radiodensity values between the six endodontic biomaterials (p<0.001) and between four different thicknesses (p<0.001).
Descriptive statistics of the effect of different endodontic material on radiodensity, the result of the general linear model, and the post-hoc Tukey’s test.
Factors | | n | Mean | Standard deviation | p-value | Post hoc** (Tukey HSD) |
---|
Thickness | 1.0 mm | 18 | 206.64 | 83.99 | <0.001* | A |
0.5 mm | 18 | 68.92 | 24.64 | B |
0.2 mm | 18 | 17.90 | 4.91 | C |
0.1 mm | 18 | 11.97 | 4.37 | D |
Materials | AH26 | 12 | 99.10 | 103.24 | <0.001* | A |
Nano MTA | 12 | 97.43 | 104.93 | A |
Endoseal MTA | 12 | 87.86 | 101.42 | B |
Endosequence BC sealer | 12 | 85.51 | 93.87 | B |
Endocem Zr | 12 | 71.88 | 77.67 | C |
Aalborg White Control | 12 | 16.38 | 10.85 | D |
Thickness *Materials | | | | | <0.001* | Refer to [Table/Fig-2] |
*Statistically significantly different when p-value<0.05.
**Multiple comparisons: Sample letter means no significant difference. A>B>C>D.
Tests applied: Two-way ANOVA and Post-hoc Tukey’s test
[Table/Fig-5] revealed that AH26 and Nano-MTA groups had significantly higher radiodensity than the other groups; however, there was no significant difference between the AH26 and Nano-MTA groups.
The four different thicknesses of endodontic biomaterials were significantly different in radiodensity values, with the highest radiodensity in the groups with 1 mm thickness (206.6±83.99), followed by 0.5 mm thickness (68.9±24.6), 0.2 mm thickness (17.9±4.9) and 0.1 mm thickness (11.97±4.3). [Table/Fig-6] also revealed that all four different thicknesses of endodontic biomaterials were significantly different in radiodensity values. The 1 mm thickness had highest radiodensity (192.88±78.5), followed by 0.5 mm thickness (67.2±26.8), 0.2 mm thickness (28.4±13.0), and 0.1 mm (12.35±4.8). The interaction of the thickness and endodontic biomaterials variables in general linear model was also statistically significant (p<0.001).
Descriptive statistics of the effect of particle size on radiodensity, the results of the General Linear Model, and the post-hoc Tukey’s test.
Factors | | n | Mean | Standard deviation | p-value | Tukey HSD Post hoc |
---|
Thickness | 1.0 mm | 21 | 192.88 | 78.51 | <0.001 | A |
0.5 mm | 21 | 67.20 | 26.83 | B |
0.2 mm | 21 | 28.40 | 13.02 | C |
0.1 mm | 21 | 12.35 | 4.80 | D |
Materials | Fine GIII (120 nm) | 12 | 112.68 | 108.47 | <0.001 | A |
Thin GII (200 nm) | 12 | 100.86 | 102.42 | B |
Fine GVI (20-40 nm) | 12 | 99.74 | 95.14 | B |
Coarse GI (10 μm) | 12 | 76.66 | 74.75 | C |
Thin GV (1~3 μm) | 12 | 63.19 | 67.32 | D |
Coarse GIV (5 μm) | 12 | 49.66 | 51.59 | E |
MTA without Radiopaque agent GVII (100%) | 12 | 23.67 | 19.68 | F |
Thickness *Materials | | | | | <0.001 | Refer to [Table/Fig-3] |
*Statistically significantly different when p-value<0.05
**Multiple comparisons: Sample letter means no significant difference. A>B>C>D>E>F
Tests applied: Two-way ANOVA and Post-hoc Tukey’s test
[Table/Fig-6] represents the ANOVA results showing the effect of the thickness and the particle size on the radiodensity of the material. This revealed the radiodensity values for seven experimental groups: Fine GIII (120 nm), Thin GII (200 nm), Fine GVI (20-40 nm), Coarse GI (10 μm), Thin GV (1 μm), Coarse GIV (5 μm) and MTA without GVII radiopacifier (100%) with four different thicknesses (0.1 mm, 0.2 mm, 0.5 mm and 1 mm) of MTA. The general linear model showed significant differences in radiodensity values between the seven endodontic biomaterials (p<0.001) and between the four different thickness groups (p<0.001).
Post-hoc tests revealed that the Fine GIII (120 nm) group had significantly higher radiodensity value compared to the other biomaterial groups. The Thin GII (200 nm) and Fine GVI (20-40 nm) had significant higher radiodensity than the rest of the other 4 groups, however, there was no significant difference between the Thin GII (200 nm) and Fine GVI (20-40 nm) groups.
For 0.1 mm and 1 mm thick material, one-way ANOVA test showed that the Fine GIII (120 nm) and Thin GII (200 nm) materials had significantly higher radiodensity than the other materials, however, there was no significant difference between the Fine GIII (120 nm) and Thin GII (200 nm) materials. For 0.2 mm material, the Fine GIII (120 nm) material the radiodensity was significantly higher than the other materials. Same comparison for 0.5 mm showed that the GIII (100 nm) and Fine GVI (20-40 nm) had significant higher radiodensity than the other groups, but no significant difference between the GIII (120 nm) and Fine GVI (20-40 nm) materials.
Discussion
In current study, the radiodensity of some endodontic biomaterials with different thicknesses was evaluated to ensure that all the materials were detectable even with the lowest thickness. In addition, the effect of radiopacifier particle size on radiodensity of MTA without its radiopacifier was evaluated in different thicknesses.
Different endodontic materials contain different radiopacifiers to enhance their radiopacity and make these materials easily distinguishable from adjacent anatomical structures on the radiograph.
Bismuth oxide is added to MTA to increase the radiopacity to higher levels than the equivalence of 3 mm of aluminum suggested by ISO 6876 (2002). For ProRoot, an observation from A1 was made between 5.34 mm and 6.92 mm [12,13]. White ProRoot MTA exhibited higher radiopacity than the grey version [13]. Modifications in the MTA formula and/or the development of new CSC materials have been proposed [14]. The low radiopacity of Portland Cement (PC) [14] makes it necessary to add a radiopacifier. Radiopacifiers such as zirconium oxide [15] are materials with a high relative molecular mass. Similar properties are seen when comparing MTA to gold powder, barium sulfate, and silver/tin alloy [7].
The effect of using nano-size bismuth oxide was studied previously [16]. CSC with 10% nano-bismuth oxide showed lower radiopacity values while significant differences in radiopacity where not shown when comparing CSC with 20% to regular bismuth oxide groups [17]. It is possible that agglomeration may occur due to Nano-form materials, resulting in lower radiopacity to X-rays. Lower radiopacity among nano-mixture groups compare to nano-WMTA has been reported in addition to an almost equal radiopacity between CSC with 20% bismuth oxide and nano-WMTA group [17]. Zirconium Oxide (ZrO2), a crystalline dioxide of zirconium, is a bioinert material with high mechanical strength, excellent resistance to corrosion, and good biocompatibility [18]. Its characteristics, such as tooth-like colour, ability to be milled, and low affinity to plaque, make ZrO2 suitable for esthetically important areas of the oral cavity [19]. ZrO2 has no cytotoxic effects on fibroblasts [20]. ZrO2 implants had no adverse responses when inserted into bone or muscle in in-vivo models [21]. Different concentrations of ZrO2 were added to PC and it was observed that adding 30% ZrO2 to PC resulted in radiopacity, compressive strength, setting time, water absorption and solubility similar to ProRoot MTA [22]. It has been demonstrated that all percentage replacements of cements mixed at both water/powder and water/cement proportions of 0.3 had radiopacities greater than 3 mm of Aluminium. The 40% and 50% replacements mixed at a water/powder proportion of 0.3 and the 30-50% replacements mixed at water/cement proportion of 0.3 had comparable radiopacities to MTA. This indicates that the water/cement proportion had an effect on the results of radiopacity testing. This is in accordance with previous research where dilution of MTA resulted in a reduction in radiopacity of the material [23].
All the materials used in this study exhibited some level of radiodensity; however, the less the thickness, the harder the detection and the less the radiodensity. However, the materials could be detected even at the lowest thickness (0.1 mm). Some materials such as AH26 and nano-modified MTA were more detectable than the others, which might be due to the nature of their radiopacifiers [24]. The effect of thickness on radiodensity was different for each material and showed significant differences from the control group. Radiodensity is greatly affected by the size and probably the shape of the particles as well. Moreover, the effect depends on the nature of the radiopacifier. It was reported that the increase in radiopacity was approximately linear when related to the increase in the percentage of zirconium oxide in samples [22], which is somehow consistent with the present study results. A materials water absorption is shown to be impacted by the cement particle shape and size [7]. Greater water absorption was seen in one study. This was due to an increased surface area from mixing with distilled water and adding fine-particle radiopacifiers [25].
In this study, the CSCs used to formulate the experimental cements were produced by the same manufacturer; thus, there was no interference in the results. The materials used in this study had no major contaminants; therefore, the radiopacity changes were due to the radiopacifier itself and not caused by any other contamination. The alternative radiopacifiers replaced the cement portion by weight. This was carried out to standardise the amount of radiopacifier added to the cement. These radiopacifiers had different relative atomic masses, which affected the amount of material added to make up the necessary weight of the material used. Radiopacifiers that do not absorb water cause a decrease in the water/cement ratio [26]. Specimen size can also affect the resultant radiopacity of the material. ISO 6876 (2002) suggests the use of specimens 10 mm in diameter and 1 mm in thickness.
This method allows the use of a lower dose of radiation since digital image receptors are more sensitive than conventional films [27], avoids the errors involved with film processing and does not require the need for an optical densitometer. Any variables in film processing might also hinder interpretation of clinical radiographs [28]. Variations may also arise from differences in the techniques used to evaluate radiopacity; however, the ISO 6876 (2002) does not provide any details in this regard. Some researchers used linear regression [12] or converted the radiographs to digital images [29] and measured the grey pixel values. In most publications, no details are provided whether the base and fog values decreased in optical density calculations. No technical detail is given on parameters used [13,30].
Limitation(s)
In the present study, all the parameters were similar to avoid any interference with radiodensity evaluation. The main limitation of the study was that from more than six radiopaque agents, only two Bismuth and Zirconia oxide were evaluated. In addition, current study doesn’t focus on the effect of particle size of radiopaque agents and the setting reaction mechanism of Biomaterials which is strongly recommended for future research.
Conclusion(s)
It can be concluded that particle size of radiopacifier is an effective factor that can influence the radiodensity of radiopacifier agents. However, Bismuth and zirconia oxides lowest particle size indicated the highest level of radiodensity, which can result in better detection in clinical scenarios. Future studies in this area are needed to assess the effect of particle radiopacifier agents on setting reaction of endodontic biomaterials.
*Statistically significantly different when p-value<0.05.**Multiple comparisons: Sample letter means no significant difference. A>B>C>D.Tests applied: Two-way ANOVA and Post-hoc Tukey’s test*Statistically significantly different when p-value<0.05**Multiple comparisons: Sample letter means no significant difference. A>B>C>D>E>FTests applied: Two-way ANOVA and Post-hoc Tukey’s test