Introduction
Due to the escalation of antibiotic resistant pathogens, non-antibiotic approaches to clinical treatment are being investigated, particularly phototherapy. In this study, 470 nm blue light was examined as an antimicrobial agent against various isolates of S. aureus and P. aeruginosa in vitro.
Aim
To determine the adequacy of blue light as an antimicrobial agent against the two pathogens infamous for rapid development of drug resistance.
Materials and Methods
ATCC strains of each organism, along with 25 strains of each from patient isolates, were collected. Isolates were suspended in peptone water at 0.5McF, and then inoculated on plates of Mueller-Hinton agar (control and experimental plate). Antibiotic sensitivity of each isolate was determined with 7 common antibiotics, following which, experimental plates were irradiated with a 470 nm Light Emitting Diode (LED) for 60 minutes. Plates were then placed in incubators overnight at 37°C. Zones of inhibition for each experimental plate were compared with the control to determine any action of the blue LED with the antibiotics. Results of the study were analysed using paired t-test where p<0.05 and was calculated for all drugs which showed significant increase in the zones of inhibition following 470 nm blue light irradiation.
Results
Results demonstrated an increase in zones by 0 to 6 mm, though this was predominantly seen in isolates of P.aeruginosa. The action of the LED was particularly significant with Linezolid for S.aureus and Imipenem for P.aeruginosa, where there was a mean increase of 2 mm and 3 mm, respectively. Comparison of Ciprofloxacin in both pathogens demonstrated a greater increase of zones in plates of P.aeruginosa (2.23 cm) as compared to S.aureus (1.27 cm), suggesting greater sensitivity of this organism to the 470 nm light.
Conclusion
This study determined that 470 nm blue light does demonstrate a species-specific inhibitory effect on S.aureus and P.aeruginosa and can act synergistically with antibiotics. With further research regarding its mechanism of action and safety, blue light therapy may be implemented into clinical treatment of skin, wound and burn infections as an adjunct to antibiotics.
Introduction
The development of multidrug resistance has popularised the study of phototherapy. Beginning in 1877, when Down and Blunt detected that light exposure inhibited fungal growth, modern medical phototherapy has grown to become a common tool in treatment of various conditions, such as psoriasis and neonatal jaundice [1]. Blue light, between 450-470 nm, has been known to demonstrate antimicrobial effect on Propionibacterium acnes. It acts by stimulating endogenous porphyrins and inducing the activation of radical oxygen species in bacteria [2].
Microorganisms studied under blue light are not limited to S. aureus and P. aeruginosa. Other studies observed the effects of 405 nm blue light on various gram positive bacteria, including S. epidermidis, S.aureus and gram negative bacteria, such as E. coli, P. aeruginosa, etc., [3,4]. All the organisms showed elimination by upto 4.2-log10 reduction in CFU counts, except for E. faecalis [4]. To compare species, a study described a 62% inactivation of S. aureus, and 96.5% inactivation of P. aeruginosa following irradiation of isolates with 15 J/cm2 of 470 nm light [5]. In another study, exposure of isolates to 405 nm blue light with 15 J/cm2 produced 90% and 91% inactivation of S.aureus and P.aeruginosa, respectively [6].
This study, in particular, focuses on the effect of 470 nm blue light. At this wavelength, blue light is safer for use on human tissue as compared to lower wavelengths closer to the UV spectra and is able to penetrate human skin deeper than shorter wavelengths of blue light. Additionally, 470 nm blue light has been shown to demonstrate improved effect on commonly drug resistant bacteria, such as Pseudomonas aeruginosa.
It has been noted from previous experiments that the effects of the 450-470 nm light on each organism may vary. This is particularly due to differences in porphyrin production and the wavelength absorption spectra of these individual porphyrins. Studies demonstrate that S.aureus predominantly produces coproporphyrins, while gram-negative bacteria show no primary porphyrin that is produced [2]. Alternatively, one experiment noted that P.aeruginosa produces coproporphyrin, uroporphyrin and carboxyporphyrinogens [7]. In one instance, a 405 nm LED was used to irradiate community acquired and hospital acquired strains of Methicillin-Resistant Staphylococcus aureus (MRSA) on tryptic soy agar [5,6]. An exposure of 100 mW/cm2 produced 92.1% and 93.5% eradication of the community-acquired and hospital-acquired strains, respectively [6]. The same two strains of MRSA were later irradiated with a 470 nm LED at a dose of 55 J/cm2, which demonstrated a 90.4% inactivation of bacteria of both strains [5]. In contrast, in a study where P. aeruginosa was irradiated at various energy doses, inactivation increased from 95.1% with the 420 nm LED to 96.5% with the 470 nm LED [8]. Notably, the prior study demonstrated a greater effect on S. aureus by lower wavelengths of light.
In a clinical setting, blue light irradiation must take into consideration tissue penetration of blue light. Wavelengths between 400-500 nm have the ability to penetrate human tissue up to epidermal level, with higher wavelengths showing further penetration [9]. This not only reinforces the preference of a longer wavelength of blue light, but also highlights the role of such treatment in superficial infections. In a particular study, 415 nm blue light irradiation of P.aeruginosa infected burns in mice, exhibited complete bacterial luminescence elimination when exposed to 55.8 J/cm2, while untreated mice died of severe infection within 72 hours [10]. Additionally, blue light has demonstrated acceleration of wound healing when excision wounds on rats were irradiated with a 470 nm LED for 10 minutes daily at 50 mW/cm2 [11].
It is important to take note of the potential effects that blue light may have on human tissue. One study utilised 390-450 nm light at 2.8W/cm2 on human primary retinal epithelium for three hours and produced mitochondrial DNA damage and release of reactive oxygen species [12]. As such, clinical use of blue light on skin may be limited to higher wavelengths in the blue spectrum to minimise harmful effects.
The need of this study was particularly significant as the clinical use of phototherapy in superficial infections requires further exploration, particularly when used in combination with antibiotics. In order to gain knowledge on this aspect, blue light was irradiated on antibiotic sensitivity tests performed with common anti-staphylococcal and anti-pseudomonal drugs. With the understanding of the bactericidal action of blue light, it was hypothesised that exposure of cultures to the 470 nm light would result in bacterial inactivation and increase in the zones of inhibition surrounding each antibiotic disc. This study was performed as part of the Indian Council of Medical Research Short Term Studentship programme, studied the effect of blue light on S. aureus and P. aeruginosa. As the duration of this study was limited to two months, as per the criteria for ICMR-STS, it was preferential to focus on these two pathogens, which are infamous for development of antibiotic resistance and known for their role in superficial infections.
The aim of this experiment was to examine the adequacy of 470 nm blue light as an antimicrobial agent for P.aeruginosa and S.aureus. Objectives for the study included confirming the antimicrobial effect of blue light on Pseudomonas aeruginosa and Staphylococcus aureus, and demonstrating any adjuvant effect of blue light with antibiotic drugs.
Materials and Methods
The following investigation was a non-randomized experimental study and has received required ethical approval by the responsible committee (DCGI Reg. no. ECR 518). It was carried out at Bharati Vidyapeeth Medical College, Pune, Maharashtra, India with the Department of Microbiology from July to August 2015.
The inclusion criteria comprised of strains of S.aureus and P.aeruginosa isolated from patient samples including pus, blood, urine, etc., while the exclusion criteria included any other isolate. A sample size of 25 strains of S.aureus and 25 strains of P.aeruginosa was used in this study. Control groups included one quality control strain of each organism, Staphylococcus aureus ATCC 25923 and Pseudomonas aeruginosa ATCC 27853, along with 25 patient sample strains of each organism; these were grown on Mueller-Hinton agar with respective antibiotic discs, without exposure to blue LED. The total sample size investigated, thus included 26 strains of S. aureus and 26 strains of Pseudomonasaeruginosa. Based on sample sizes used in previous studies, such as by Yin R et al., and the limitation of a two month period to complete this study, the decision was made to limit the sample size to 25 strains of each organism [4].
The light source used in this study [Table/Fig-1] includes an 8W LED emitting 450-470 nm wavelength of blue light (manufacturer: Art LED lighting Co., Ltd.,). It is incorporated into an apparatus consisting of a 40×30×25 cm cardboard box containing a 27×6 cm opening for adjustment of the LED tube. The apparatus was placed within a type II Biosafety Cabinet to maintain air ventilation and room temperature during irradiation [Table/Fig-2].
Features of blue light emitting diode.
Parameter | Symbol | Data | Unit |
---|
Max power dissipation | PM | 800 | mW |
Max continuous forward current | IFM | 150 | mA |
Max reverse voltage | VRM | 5 | V |
Peak forward current | IFP | 300 | mA |
Lead soldering temperature/Time | TSOL | 240;3 | °C; S |
Operating temperature range | TOPR | -25+85 | °C |
Storage temperature range | TSTR | -30+100 | °C |
Dominant wavelength | λd | 470 | Nm |
Luminous intensity | Iv | 15-20 | LM |
Apparatus consisting of a 40×30×25 cm cardboard box containing a 27×6 cm opening for adjustment of the LED tube. This was placed inside a Type II Biosafety cabinet.
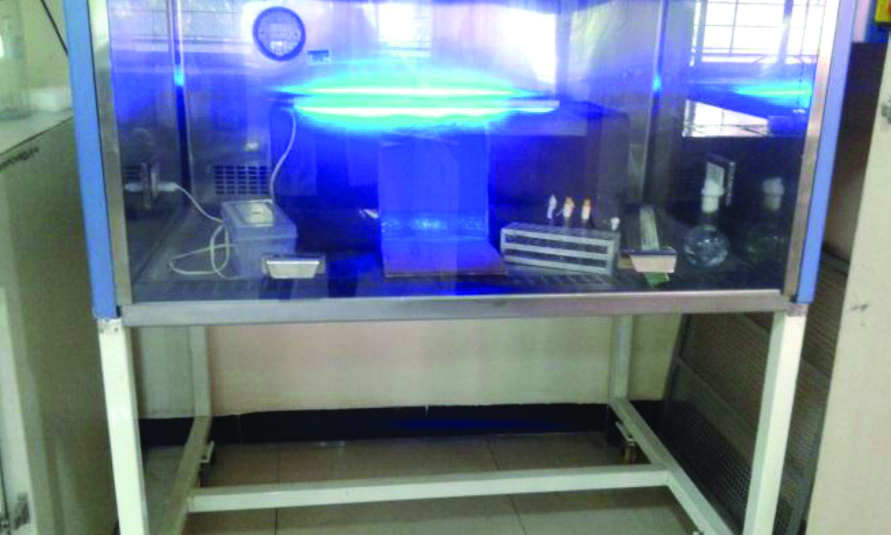
Procedure
Each isolate was inoculated in peptone water and incubated for 2 to 4 hours at 37°C. Bacterial density was adjusted to 0.5 McF using MacFarland’s standard solution to ensure uniformity [13,14].
Isolates were inoculated on Mueller-Hinton agar plates, following which antibiotic sensitivity was tested using Kirby-Bauer disc diffusion as per Clinical and Laboratory Standards Institute (CLSI) [13] protocols and recommendation. Mueller-Hinton agar was prepared with a pH of 7.2-7.4 at room temperature, and with minimal thymidine content. The media was moist, but without the presence of water droplets on its surface. Bacterial suspensions were inoculated as a lawn culture utilising sterile cotton swab. Plates were dried at 37°C for 30 minutes. With sterile forceps, 7 antibiotic discs for each organism were applied on the 90 mm plates, 24 mm apart from one another. The experimental plates of each isolate were then irradiated with the blue LED for 60 minutes, while the remaining plates were not exposed to LED. With a maximum power dissipation of 800 mW [Table/Fig-1], the intensity of irradiation of the 90 mm plates (with an area of 63.62 cm2) were measured to be approximately 12.57 mW/cm2 [15].
While previous experiments generally utilised a stronger intensity of irradiation, upto 100 mW/cm2 in some instances [6], it has been noted that human foreskin keratinocytes exposed to 450 nm light at 6.3 mW/cm2 for 20 minutes caused Reactive Oxygen Species (ROS) production and cellular damage [16]. Keeping in mind the clinical relevance of this study, a lower dose of 12.5 mW/cm2 over the period of one hour was decided upon due to its easy measurability and therapeutic safety. Plates were irradiated at a distance of 25 cm to ensure uniform exposure of the light across the surface of the cultures. All plates were then incubated overnight at 37°C.
Antibiotics tested for S. aureus were Penicillin, Chloramphenicol (30 μg), Ciprofloxacin (5 μg), Cotrimoxazole (1.25/23.75 μg), Levofloxacin (5 μg), Linezolid (30 μg) and Cefoxitin (30 μg). P. aeruginosa was exposed to Piperacillin (100 μg), Aztreonam (30 μg), Ceftazidime (10 μg), Ciprofloxacin (5 μg), Amikacin (30 μg), Gentamycin (10 μg) and Imipenem (10 μg).
Antibiotic sensitivity of each isolate was examined by measurement of the zones of inhibition surrounding each disc to the nearest millimetre. The results were then compared with standards released by CLSI to determine sensitivity of the bacteria to each drug [13]. The zones of inhibition found in the control plates were then compared to those in the experimental plates that were irradiated with the 470 nm LED to detect any change in zone size.
Statistical Analysis
Statistical analysis of the data was performed by measuring the mean, median and range zones of inhibition for each antibiotic disc amongst the 25 strains of each organism. The results were then analysed for significance using paired t-test for each drug with p<0.05 and N=26. The results for ciprofloxacin, which was utilised with all 52 strains used in this study were analysed with unpaired T-test, where p<0.05 was considered significant. Data was analysed using SPSS version 20.0.
Results
[Table/Fig-3] depicts number of observations demonstrating an increase in zone of inhibition by 0-7 mm following LED exposure with the total number of observations being 175 per organism; this figure includes 7 antibiotic sensitivity observations per plate for each of the 25 test strains. Both S.aureus and P.aeruginosa exhibited a number of isolates displaying no effect of the blue LED, specifically 55 and 42 observations, respectively. However, in both cases, majority of observations demonstrated an increase in zones of inhibition. For S. aureus, 55 zones of inhibition were seen to increase by 2 mm, making this the most common observance. Alternatively, P. aeruginosa displayed 2 mm and 3 mm increase in zones by 43 observations each. Overall, 68.5% of observations for S. aureus and 76.0% of observations for P. aeruginosa demonstrated an increase in size of zones of inhibition following LED exposure.
Observations demonstrating increase in zones of inhibition of drugs following LED exposure.
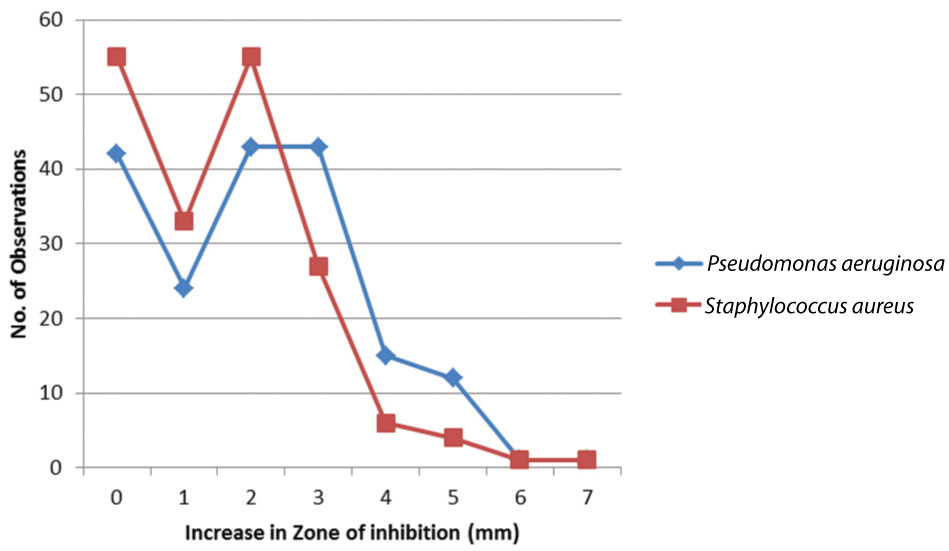
On the other hand, increase in drug sensitivity from resistant to intermediate or susceptible, as per CLSI standards, demonstrated minimal alterations following LED irradiation, with Cefoxitin demonstrating the largest number with 7 isolates. In case of P. aeruginosa, 4 isolates displayed increased sensitivity from resistant to intermediate to susceptible for as per CLSI standards for imipenem and 6 isolates for ceftazidime [Table/Fig-4,5]. Samples of S. aureus and P. aeruginosa demonstrating antibiotic sensitivity with and without LED exposure were shown in [Table/Fig-6,7], there was significant increase in the zones of inhibition when exposed to 470 nm light.
Increase in drug sensitivity to Anti-staphylococcal antibiotics following LED exposure.
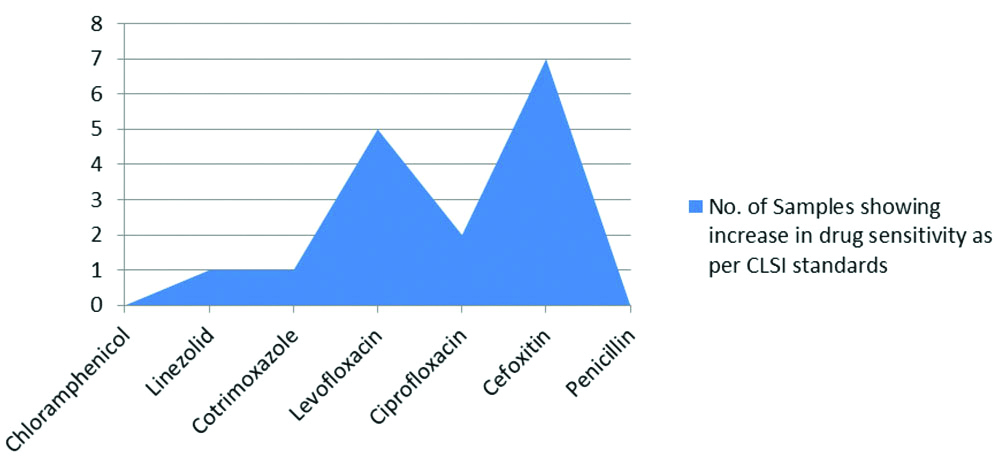
Increase in drug sensitivity to Anti-pseudomonal antibiotics following LED exposure.
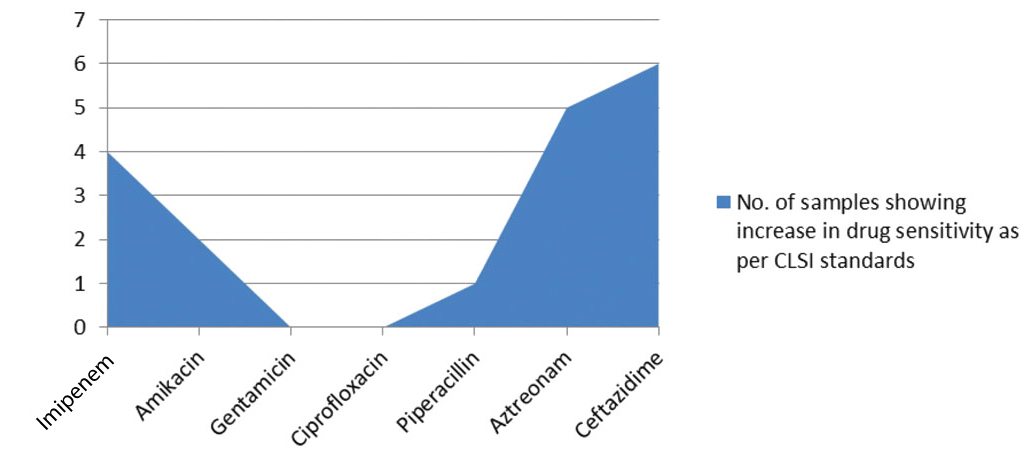
Sample of S. aureus demonstrating antibiotic sensitivity with and without LED exposure. Note the significant increase in the zones of inhibition for linezolid and chloramphenicol when exposed to 470 nm light.
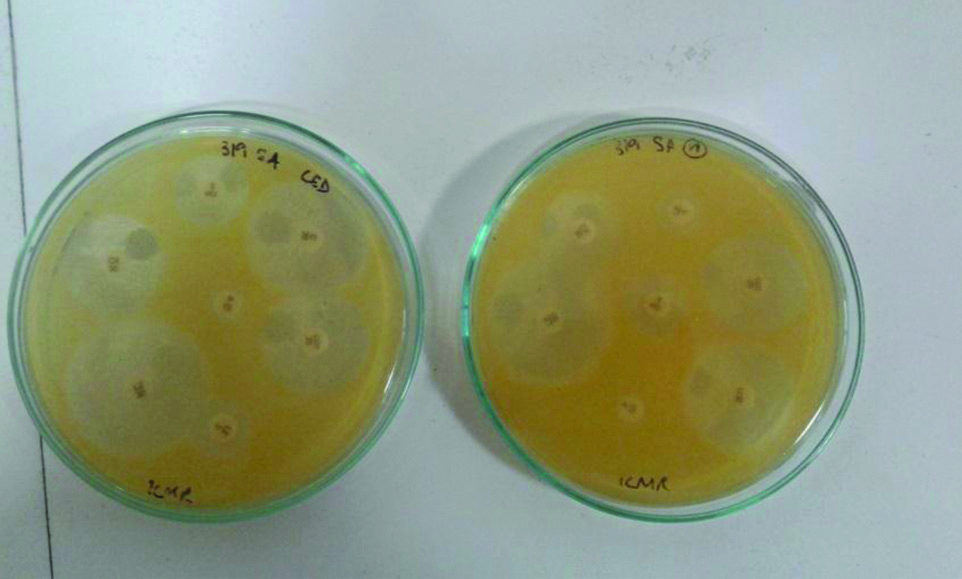
Sample of Pseudomonas aeruginosa demonstrating antibiotic sensitivity with and without LED exposure. Note the increase in zones of inhibition for piperacillin/tazobactam when exposed to 470 nm light.
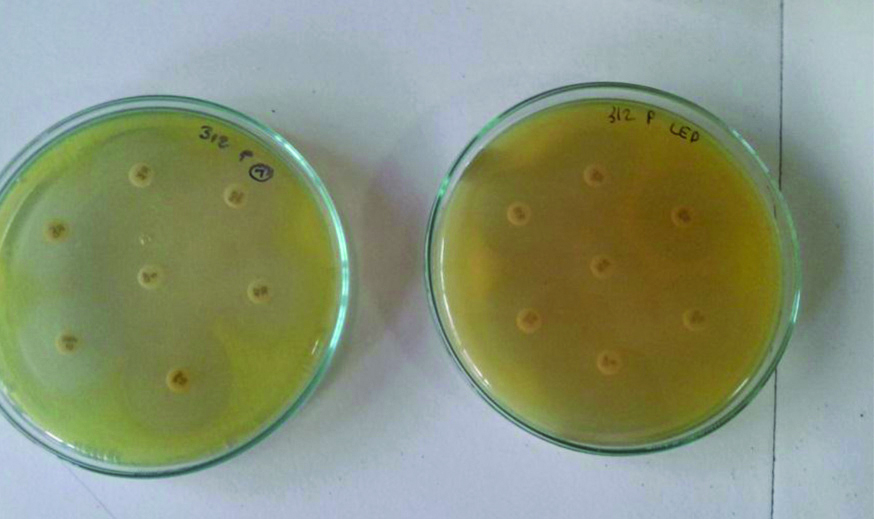
S.aureus Results
Effect of the blue LED irradiation on each drug in the standard and isolate strains was also determined. Linezolid demonstrates a markedly higher mean value, at 2.00 mm, as compared to other drugs [Table/Fig-8]. Ranges were broader as most drugs showed increase in zones as much as 6 mm. Isolate 23 of S. aureus displayed a zone of inhibition increasing from 29 mm to 33 mm in Linezolid. The same isolate showed a Cotrimoxazole zone improving from 17 mm to 23 mm and no increase in Cefoxitin. Despite an average increase in drug sensitivity, Levofloxacin, Cotrimoxazole and Cefoxitin still demonstrated no increase in zone of inhibition as the mean observance. However, significant improvement in drug sensitivity was also seen for these antibiotics. For example, isolate 1 of S. aureus showed increase in Cefoxitin zones from 20 mm to 27 mm. Of all antibiotics tested, Levofloxacin and Cotrimoxazole also displayed the lowest mean increase in the zones. Significance of these results were examined with paired t-test where p<0.05 and N=26. T-values calculated for all drugs showed significant increase in zones of inhibition following 470 nm blue light irradiation.
Sensitivity of Staphylococcus aureus to various antibiotics with and without LED exposure.
| | Mean zone of inhibition to all 25 isolates (mm) | Range of zone of inhibition (mm) | Standard deviation | Paired t-test | p-value |
---|
Levofloxacin | No LED | 18.23 | 6.0-30.0 | 6.68 | 5.156 | 0.05 |
LED | 19.46 | 6.0-30.0 | 6.59 |
Increase in zone of inhibition | 1.23 | 0.5-0 | 1.28 |
Chloramphenicol | No LED | 23.69 | 8.0-29.0 | 4.03 | 7.573 | 0.05 |
LED | 25.50 | 11.0-30.0 | 4.12 |
Increase in zone of inhibition | 1.81 | 0-4.00 | 1.22 |
Ciprofloxacin | No LED | 17.65 | 6.0-32.0 | 7.35 | 5.400 | 0.05 |
LED | 18.92 | 6.0-34.0 | 7.61 |
Increase in zone of inhibition | 1.27 | 0-5.0 | 1.21 |
Cotrimoxazole | No LED | 15.08 | 6.0-25.0 | 8.05 | 4.022 | 0.05 |
LED | 16.31 | 6.0-26.0 | 8.68 |
Increase in zone of inhibition | 1.23 | 0-6.0 | 1.56 |
Penicillin | No LED | 10.38 | 6.0-32.0 | 6.93 | 5.537 | 0.05 |
LED | 12.04 | 6.0-35.0 | 7.59 |
Increase in zone of inhibition | 1.65 | 0-6.00 | 1.52 |
Linezolid | No LED | 25.88 | 6.0-34.0 | 7.82 | 8.439 | 0.05 |
LED | 27.88 | 6.0-36.0 | 8.24 |
Increase in zone of inhibition | 2.00 | 0-5.00 | 1.21 |
Cefoxitin | No LED | 18.54 | 6.0-26.0 | 5.70 | 5.113 | 0.05 |
LED | 20.11 | 6.0-27.0 | 6.26 |
Increase in zone of inhibition | 1.58 | 0-4.0 | 1.57 |
P. aeruginosa Results
There was a stark increase in zones surrounding Imipenem particularly following LED illumination. Along with an average of 3.00 mm and a mode of 4.00 mm seen on [Table/Fig-9], this drug also demonstrated the widest range of results, extending up to 7 mm increase in zones of inhibition. Isolate 22 of P. aeruginosa demonstrated an increase in zone of inhibition surrounding Imipenem from 11 mm to 18 mm. The same isolate displayed 4 mm increase in Amikacin and Piperacillin, and 5 mm increase in Gentamycin. Isolate 7 showed an increase in Imipenem zone from 14 mm to 19 mm and no improvement in zones of inhibition surrounding any other drugs. Lowest mean increase in zones of inhibition were seen with Piperacillin, at 1.69 mm, despite a mode value of 3 mm. Aztreonam and Ceftazidime, on the other hand, showed higher mean increase in zones despite a mode of 0 mm. Isolate 18 of Pseudomonas demonstrated an increase in Aztreonam zone from 22 mm to 27 mm, and 24 mm to 27 mm for Ceftazidime. Overall, values obtained from pseudomonal isolates displayed greater improvement in drug sensitivity.
Sensitivity of Pseudomonas aeruginosa to various antibiotics with and without LED exposure.
| | Mean zone of inhibition to all 25 isolates (mm) | Range of zone of inhibition (mm) | Standard deviation | Paired t-test | p-value |
---|
Gentamycin | No LED | 17.31 | 6.0-24.0 | 6.14 | 6.130 | 0.05 |
LED | 19.23 | 6.0-27.0 | 6.83 |
Increase in zone of inhibition | 1.92 | 0-5.0 | 1.59 |
Amikacin | No LED | 20.04 | 6.0-25.0 | 2.98 | 7.809 | 0.05 |
LED | 22.00 | 6.0-28.0 | 4.98 |
Increase in zone of inhibition | 1.96 | 0-5.00 | 1.28 |
Piperacillin | No LED | 19.54 | 6.0-25.0 | 5.59 | 6.926 | 0.05 |
LED | 21.11 | 6.0-28.0 | 5.42 |
Increase in zone of inhibition | 1.69 | 0-4.0 | 1.24 |
Aztreonam | No LED | 18.19 | 6.0-29.0 | 6.77 | 5.227 | 0.05 |
LED | 19.92 | 6.0-32.0 | 7.61 |
Increase in zone of inhibition | 1.73 | 0-5.0 | 1.69 |
Ceftazidime | No LED | 17.73 | 6.0-26.0 | 6.73 | 5.836 | 0.05 |
LED | 19.57 | 6.0-27.0 | 7.63 |
Increase in zone of inhibition | 1.85 | 0-5.0 | 1.62 |
Ciprofloxacin | No LED | 26.50 | 6.0-35.0 | 10.70 | 7.458 | 0.05 |
LED | 28.73 | 6.0-38.0 | 11.04 |
Increase in zone of inhibition | 2.23 | 0-5.00 | 1.53 |
Imipenem | No LED | 23.04 | 11.0-30.00 | 5.17 | 10.169 | 0.05 |
LED | 25.96 | 18.0-34.0 | 4.87 |
Increase in zone of inhibition | 3.00 | 0-7.0 | 1.51 |
For observations of P.aeruginosa, the mean increase in zones ranged from 1.69 mm to 3.00 mm, which is markedly greater than seen in the anti-staphylococcal drugs [Table/Fig-10,11]. Following paired t-test of each drug with p<0.05 and N=26, all drugs demonstrated significant increase in zones of inhibition following blue light irradiation.
Average increase in zones of inhibition of anti-pseudomonal drugs following blue light irradiation.
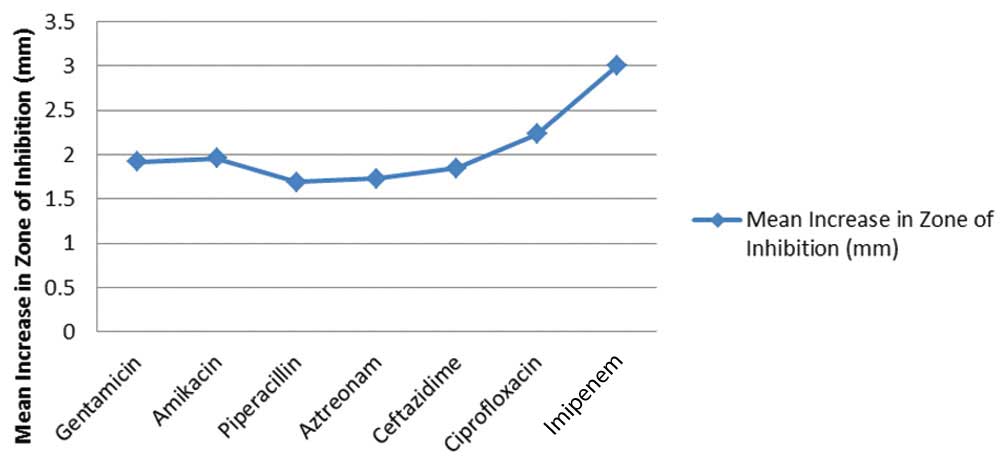
Average increase in zones of inhibition of anti-staphylococcal drugs following blue light irradiation.
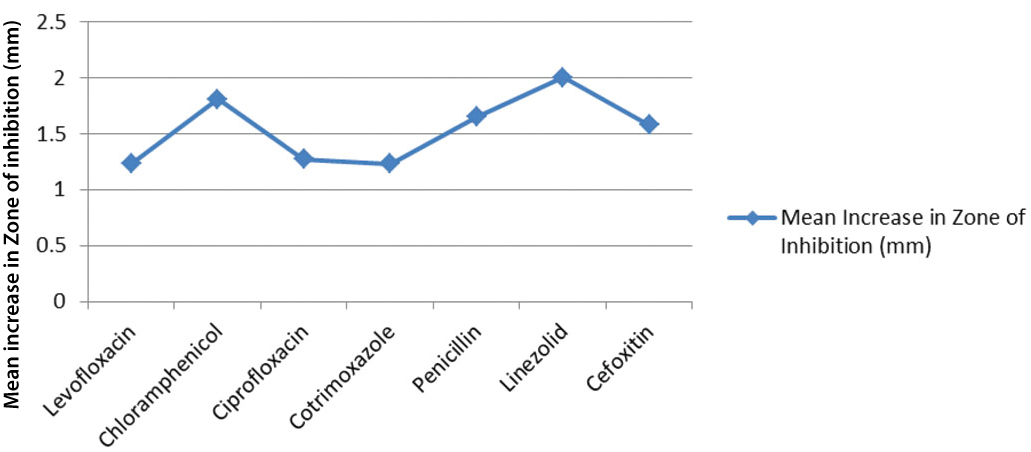
To compare the effects of the 470 nm LED on S.aureus and P.aeruginosa, the effect of irradiation on sensitivity to Ciprofloxacin in each organism was examined. This was a secondary aim of the study at hand, as its primary purpose was to determine the effect of blue light on sensitivity to commonly used anti-staphylococal and anti-pseudomonal drugs. As such, it was decided to utilise only ciprofloxacin for comparison between the organisms. [Table/Fig-12] displays that the average increase in zone of inhibition of Ciprofloxacin was 2.23 mm in P. aeruginosa as compared to the 1.27 mm in S. aureus. A 95% confidence interval of each mean was also determined and once again, improved drug sensitivity in Pseudomonas proved far greater than in Staphylococcus. Significance was determined with unpaired t-test, where p<0.05 was considered significant. Calculated T-value suggests that difference in the values obtained in P.aeruginosa and S.aureus was significant.
Comparison of effect of LED irradiation on zones of inhibitions of Ciprofloxacin in S. aureus and P. aeruginosa.
| Pseudomonas aeruginosa | Staphylococcus aureus |
---|
N | 26 | 26 |
Average increase in zone of inhibition (mm) | 2.23 | 1.27 |
Standard deviation | 1.53 | 1.21 |
Confidence interval of mean | 0.955-3.205 | 0.318-2.132 |
Pooled standard deviation | 0.497 |
Unpaired t-test | 6.966 |
p | 0.05 |
Discussion
The inhibitory role of blue light was demonstrated by the overall improvement in drug sensitivity following irradiation by the light, particularly in P.aeruginosa. The effect of blue light resulted in improved effects of various antibiotics, especially with Linezolid for S.aureus and Imipenem for P.aeruginosa.
The inhibitory effect of 470 nm blue light was demonstrated by the increase in zones of inhibition surrounding antibiotic discs. A study examined levels of ROS in various strains of S.aureus treated with 420 nm light and found higher levels of ROS following irradiation [2]. The increased zones of inhibition following irradiation are likely caused by photo-inactivation of the bacteria, improving the effect of the drugs. This finding is similar to another studies performed on S.aureus and P.aeruginosa with 470 nm LED which observed MRSA inactivation with 470 nm at 55 J/cm2 irradiation to find that 90.4% inactivation of the pathogen took place [5]. In other studies, 470 nm irradiation of P.aeruginosa at 5 J/cm2 inactivated 96.5% of growth [17].
Similar to other experiments, the action of the blue light was predominantly displayed in plates of P.aeruginosa rather than S.aureus. This is demonstrated by the mean increase in zones of inhibition of Ciprofloxacin being 2.23 mm in the former and 1.27 mm in the latter. Overall, the mean increase in zones of inhibition per drug was greater in P.aeruginosa, with the exception of Chloramphenicol and Linezolid, 1.81 mm and 2.00 mm, respectively, whose results were comparable to that of Pseudomonas. Similar results were found in other studies, where 470 nm light was used to irradiate the same two pathogens and demonstrated a 96.5% and 62% kill rate of P.aeruginosa and S.aureus, respectively [8]. Hypothetically, this variation may be caused by differing porphyrin levels in the two pathogens. A study examined porphyrin levels in various bacteria following treatment with aminolevulinic acid to find that gram positive S.aureus produced 2 times more coproporphyrin than gram-negative species, which showed no predominant porphyrin [2]. While these compounds are known to have peak absorption at approximately 400 nm, this may vary for individual porphyrins. Further studies regarding the porphyrin production in various pathogens will allow blue light therapy to be made species-specific.
The zones of inhibition showed the greatest mean increase with Linezolid, by 2.00 mm, and Imipenem, by 3.00 mm, for S.aureus and P.aeruginosa, respectively. This suggests a greater synergistic effect of blue light with these antibiotics as compared to other drugs used in this study. While observations regarding the synergistic action of blue light and antibiotics have been noted, information regarding the reason for its selectivity is unknown. Such selectivity suggests that the action of blue light affects multiple factors of microbial metabolism, resulting in a varied response to specific antibiotics.
Despite the confirmation of its bactericidal action, blue lights possible adverse effects on human tissue must be considered. Examination of mice 3T3 fibroblasts, human foreskin keratinocytes and monkey kidney epithelial cells following irradiation with 450 nm light at 6.3 mW/cm2 for 20 minutes caused ROS production and cellular damage [16]. Other studies found that the blue light on normal skin, produced only transient melanogenesis and vacuolization without apoptosis [3]. This makes 470 nm blue light therapy preferable as an adjuvant to antibiotics for Pseudomonal infections at this time.
Limitation(s)
Certain limitations found in this study may be responsible for the smaller scale action of the blue light than was expected. Most importantly, the 800 mW light source produced a relatively low dose of irradiance of 12.57mW/cm2 at the 63.63 cm2 plates. In one study, a 405 nm and 880 nm light source was utilised for irradiation of S.aureus and P.aeruginosa in doses of 1, 3, 5, 10 and 20 J/cm2. Colony counts performed thereafter depicted a dose dependant bactericidal effect of the blue light, with 20 J/cm2 proving most effective in both organisms [8]. Therefore, usage of a higher intensity light source at a closer distance may produce more significant results of improved drug sensitivity. Further, due to the time limitation of two months to complete the study at hand as per the rules of ICMR-STS, a decision was made to limit the investigation to two organisms with 25 isolates of each.
Conclusion(s)
Overall, the study performed concludes that 470 nm blue light acts synergistically with traditional antibiotics by photo inactivation of bacteria. This effect is markedly noticed in gram-negative P.aeruginosa, which is notorious for rapid development of drug resistance. The irradiation of bacteria with this blue light resulted in increased drug sensitivity, particularly with Linezolid for S.aureus and Imipenem for P.aeruginosa, however the mechanism for this increase must be further investigated. Other factors regarding blue light therapy that required additional understanding is individual porphyrin sensitivity to 470 nm blue light, mechanism of specificity of blue light to various microorganisms and in vivo examination of the effect of blue light on various human tissues.
[1]. Jarrett P, Scragg R, A short history of phototherapy, vitamin D and skin disease Photochemical & Photobiological Sciences 2017 16(3):283-90.10.1039/C6PP00406G27892584 [Google Scholar] [CrossRef] [PubMed]
[2]. Lubart R, Lipovski A, Nitzan Y, Friedmann H, A possible mechanism for the bactericidal effect of visible light Laser Therapy 2011 20(1):17-22.10.5978/islsm.20.1724155508 [Google Scholar] [CrossRef] [PubMed]
[3]. Kleinpenning MM, Smits T, Frunt MH, van Erp PE, van de Kerkhof PC, Gerritsen RM, Clinical and histological effects of blue light on normal skin Photodermatol, Photoimmunol Photomed 2010 26(1):16-21.10.1111/j.1600-0781.2009.00474.x20070834 [Google Scholar] [CrossRef] [PubMed]
[4]. Yin R, Dai T, Avci P, Jorge AE, de Melo WC, Vecchio D, Light based anti-infectives: Ultraviolet C irradiation, photodynamic therapy, blue light, and beyond Curr Opin Pharmacol 2013 13(5):731-62.10.1016/j.coph.2013.08.00924060701 [Google Scholar] [CrossRef] [PubMed]
[5]. Enwemeka CS, Williams D, Enwemeka SK, Hollosi S, Yens D, Blue 470-nm light kills Methicillin-Resistant Staphylococcus Aureus (MRSA) in vitro Photomed Laser Surg 2009 27(2):221-26.10.1089/pho.2008.241319196103 [Google Scholar] [CrossRef] [PubMed]
[6]. Enwemeka CS, Williams D, Hollosi S, Yens D, Enwemeka SK, Visible 405 nm SLD light photo-destroys Methicillin-Resistant Staphylococcus Aureus (MRSA) in vitro Lasers Surg Med 2008 40(10):734-37.10.1002/lsm.2072419065556 [Google Scholar] [CrossRef] [PubMed]
[7]. Sailer R, Strauss WSL, König K, Rück A, Steiner R, Correlation between porphyrin biosynthesis and photodynamic inactivation of Pseudomonas aeruginosa after incubation with 5-aminolaevulinic amid Journal of Photochemistry and Photobiology B: Biology 1997 39(3):236-42.10.1016/S1011-1344(96)00019-X [Google Scholar] [CrossRef]
[8]. Guffey JS, Wilborn J, In Vitro bactericidal effects of 405-nm and 470-nm blue light Photomed Laser Surg 2006 24(6):684-88.10.1089/pho.2006.24.68417199466 [Google Scholar] [CrossRef] [PubMed]
[9]. Moreira MC, Prado R, Campos A, Application of high brightness LEDs in the human tissue and its therapeutic response Applied Biomedical Engineering 2011 10.5772/19510 [Google Scholar] [CrossRef]
[10]. Dai T, Gupta A, Huang Y, Yin R, Murray C, Vrahas M, Blue light rescues mice from potentially fatal Pseudomonas aeruginosa burn infection: efficacy, safety, and mechanism of action Antimicrobial Agents and Chemotherapy 2012 57(3):1238-45.10.1128/AAC.01652-1223262998 [Google Scholar] [CrossRef] [PubMed]
[11]. Adamskaya N, Dungel P, Mittermayr R, Hartinger J, Feichtinger G, Wassermann K, Light therapy by blue LED improves wound healing in an excision model in rats Injury 2011 42(9):917-21.10.1016/j.injury.2010.03.02322081819 [Google Scholar] [CrossRef] [PubMed]
[12]. Bumah VV, Masson-Meyers DS, Cashin SE, Enwemeka CS, Wavelength and bacterial density influence the bactericidal effect of blue light on Methicillin-Resistant Staphylococcus aureus (MRSA) Photomedicine and Laser Surgery 2013 31(11):547-53.10.1089/pho.2012.346123621894 [Google Scholar] [CrossRef] [PubMed]
[13]. CLSI. Performance Standards for Antimicrobial Susceptibility Testing; Twenty-Third Informational Supplement. CLSI Document M100-S23. Wayne, PA: Clinical and Laboratory Standards Institute; 2013 [Google Scholar]
[14]. Miyabe M, Junqueira JC, Costa AC, Jorge AO, Ribeiro MS, Feist IS, Effect of photodynamic therapy on clinical isolates of Staphylococcus spp Brazilian Oral Research 2011 25(3):230-34.10.1590/S1806-8324201100500000621359491 [Google Scholar] [CrossRef] [PubMed]
[15]. Intensity Conversion Chart [Internet]. Dymax.com. 2010 [cited 14 July 2015]. Available from: https://www.dymax.com/images/pdf/technical_bulletins/lit135_intensity_conversion_chart_tb.pdf [Google Scholar]
[16]. Hockberger PE, Skimina TA, Centonze VE, Lavin C, Chu S, Dadras S, Activation of flavin-containing oxidases underlies light-induced production of H2O2 in mammalian cells Proc Natl Acad Sci USA 1999 96(11):6255-60.10.1073/pnas.96.11.625510339574 [Google Scholar] [CrossRef] [PubMed]
[17]. Dai T, Gupta A, Murray CK, Vrahas MS, Tegos GP, Hamblin MR, Blue light for infectious diseases: Propionibacterium acnes, Helicobacter pylori, and beyond? Drug Resis Updat 2012 15(4):223-36.10.1016/j.drup.2012.07.00122846406 [Google Scholar] [CrossRef] [PubMed]