Introduction
Diabetes is a metabolic disorder characterised by elevated blood sugar that results from defects in insulin production and/or insulin action, and impaired function in the metabolism of carbohydrates, lipids and proteins which leads to macro and microvascular complications [1]. There are emerging evidences that oxidative stress makes significant contribution to the progression of diabetes and its associated complications. Type 1 diabetes is a chronic illness, usually caused by an autoimmune destruction of insulin-producing β-cells in the islets of the pancreas. As a consequence, the body produces none to very little insulin resulting in a relative or absolute deficiency of insulin. T2DM also referred as Non-Insulin Dependent Diabetes Mellitus (NIDDM) or adult-onset diabetes, includes patients who have insulin resistance and usually have relative insulin deficiency [2]. However, these patients do not need insulin administration for their treatment and survival of the patient. Majority of the patients with T2DM are obese, and obesity itself further contributes to insulin resistance to some extent. The non-obese individuals with T2DM may have an increased percentage of body fat distributed predominantly in the abdominal region. According to American Diabetes Association (ADA), T2DM accounts for ~90-95 % of all diabetes mellitus patients worldwide [3]. T2DM goes undiagnosed for many years because hyperglycaemia related complications develop slowly. The risk of developing T2DM increases with age, obesity, physical inactivity, family history of diabetes, ethnicity and hyperglycaemia during pregnancy [4]. It is often related with stronger genetic predisposition as compared to the autoimmune form of type 1 diabetes.
Diabetes Mellitus in Indian Population
Diabetes is a global issue that kills and disables people at their most productive age or reduces their life expectancy. It is among the top 10 causes of death globally. Diabetes is a common threat and no country is immune to it. Diabetes mellitus is reaching an epidemic proportion in India. Diabetes is being implicated to a spectrum of complications and has shown a tendency to occur at a relatively younger age. The steady migration of people from rural to urban areas, the economic boom, and sedentary lifestyle are some of the major factors associated with increasing incidence of T2DM in India [5]. A recent study conducted by Indian Council of Medical Research (ICMR) to estimate the nationwide prevalence of diabetes in both urban and rural areas included three states and one Union Territory in the first phase. In this study, around 13000 subjects were studied with equal representation of the rural and urban population. The outcome of study projected national estimate of 62.4 million patients with diabetes and 77.2 million with pre-diabetes. The prevalence of diabetes was reported ranging from 5.3% to 13.6% in different areas. The highest prevalence was reported in Ernakulum in Kerala (19.5%) and the lowest in Kashmir valley (6.1%), while most of the other areas had the prevalence rate above 10% [6]. Indians appear to be at higher risk of development of diabetes. Apart from the conventional risk factors driven by urbanisation, industrialization, globalisation and aging, other factors such as obesity, regional adiposity, higher percentage of body fat and genetic factors also contribute to the cause. Urban migration has been linked to the increasing prevalence of diabetes in Indians [5]. Urban migrants have higher weight, Waist-Hip Ratio (WHR), Body Mass Index (BMI), Systolic Blood Pressure (SBP), cholesterol, HOMA-IR, fat intake and comparatively low physical activity. Diabetes and its complications pose a significant economic burden on countries, healthcare systems, society, individuals and their families. International Diabetes Federation (IDF) estimated that in year 2017, the total healthcare expenditure on diabetes would have been USD 727 billion (20-79 years), which represents an 8% increase compared to the 2015 estimates [7].
Genetics and Molecular Mechanism of T2DM
The genetics of T2DM is complex and not clearly defined. Insulin resistance is an important factor in T2DM that develops at several levels, with decreased receptor concentration and kinase activity, defect in phosphorylation of insulin receptor substrate-1& 2 (IRS-1 & 2), Phosphatidylinositide Kinase (PI-3-k) activity, translocation of Glucose Transporter-4 (GLUT 4) and the activity of intracellular enzymes [8]. Insulin binds to its receptors on adipocytes and skeletal muscle cells and increases the uptake of glucose from blood by stimulating the translocation of the cytosolic GLUT 4 to plasma membrane [9].
Oxidative Stress in Diabetes Mellitus
The relationship between oxidative stress and diabetes mellitus is shown in [Table/Fig-1]. Free radicals are short-lived reactive chemical species containing one or more unpaired electrons that induce damage to cells by oxidation of cell components and molecules [10]. These are usually very unstable reactive species and are said to be a necessary evil, as they play a cardinal role in origin and evolution of life. Free radicals are produced by both exogenous and endogenous substances in cells and their surroundings by non-enzymatic oxidation reactions of organic compounds and by reaction initiated by ionising radiations. This process may also occur in mitochondrion by oxidative phosphorylation [11]. Oxidative stress plays a key role in cellular injury. Hyperglycaemia can stimulate free radical production. When defense system of the body becomes impaired, it is unable to neutralise the Reactive Oxygen Species (ROS) generation resulting in an imbalance between ROS and cellular protection mechanism which leads to oxidative stress. Any imbalance between ROS and antioxidants is deleterious to health. ROS are also produced by neutrophils and macrophages during the process of respiratory burst in order to neutralise antigens [12]. Free radicals trigger the signaling pathways inside the cell, such as the Mitogen-Activated Protein Kinase (MAPK) and Extracellular-Signal-Regulated Kinase (ERK) pathways that alter gene expression, as well as promote Superoxide Dismutase (SOD) mediated cell death. They were mechanistically related to diminished insulin-dependent stimulation of insulin signaling elements and glucose transport activity that lead to insulin resistance [13]. In diabetes, ROS formed by non-enzymatic glycation of proteins, glucose oxidation, and increased lipid peroxidation lead to damage of enzymes, cellular machinery and also augment insulin resistance due to oxidative stress [14]. Evidences have emerged suggesting that oxidative stress plays a decisive role in systemic inflammation that contributes to the pathophysiology of several macro and micro vascular complications [15]. Diabetes mellitus significantly changes the lipid profile and makes cells more susceptible to lipid peroxidation. Literature suggests that oxidised Lipoproteins (Ox-LDL) contribute to the cardiovascular complications in diabetes and LDL oxidation in diabetic patients is significantly increased as compared to corresponding controls. The lipids and apolipoprotein component of LDL form insoluble aggregates oxidatively that are responsible for oxidative damage in diabetic complications. Free radicals also have a role in stimulating signals for increased expression of antioxidant enzymes [11]. Hyperglycaemia causes tissue damage via multiple mechanisms including formation of Advanced Glycation End products (AGEs), augmented expression of the receptor for AGEs and their activating ligands, activation of protein kinase-C isoforms, and increased activity of the hexosamine pathway [16]. Free radicals play a major role in the onset and progression of late diabetic complications due to their ability to damage lipids, proteins, and DNA. The disturbance in levels of oxidative markers makes the tissues more susceptible to oxidative stress leading to the development of diabetic complications. Oxidative stress induced complications in diabetes mellitus include coronary artery disease, neuropathy, nephropathy, retinopathy, and stroke. Moreover, diabetic mortalities can be elucidated particularly by an increase in vascular disease other than hyperglycaemia [17].
Hypothetical scheme of the relationship between oxidative stress and diabetic complications. In diabetes, augmented auto-oxidation of glucose and the increased formation of Advanced Glycation End products (AGE) produce reactive oxygen species. The elevated flux of glucose through the first half of the polyol pathway consumes NADPH. This impairs the glutathione redox cycle so that antioxidant protection is reduced. These changes lead to endothelial dysfunction which is a key element in the progression of diabetic complications.
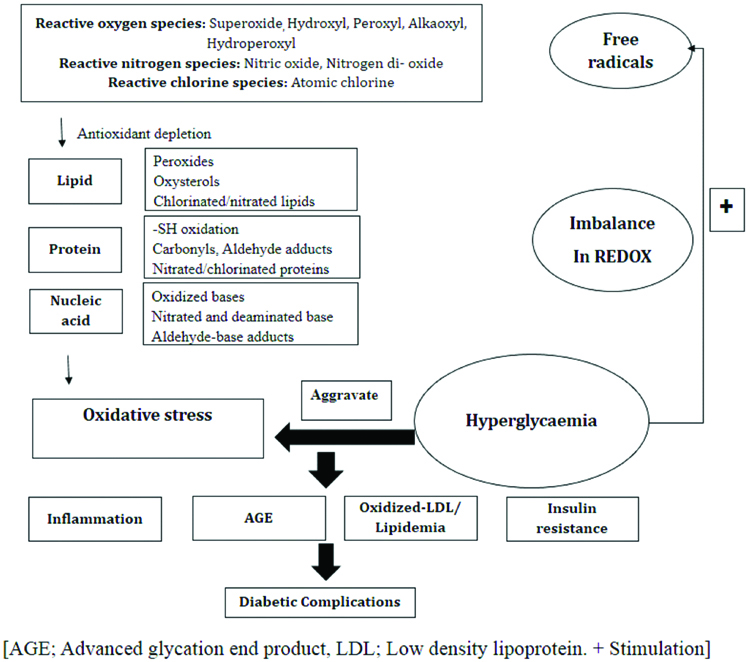
Oxidative Stress Induced Changes in Diabetes
In diabetes mellitus, oxidative stress adversely affects the cellular physiology and is predominantly relevant and dangerous for the islet of pancreas.
Lipid peroxidation: Lipids are reported to be one of the primary targets of ROS and peroxidation of lipids produces highly reactive aldehydes, including malondialdehyde (MDA), acrolein, 4-Hydroxynonenal (HNE), 4-Oxononenal (ONE), and isolevuglandins [18]. Significant alterations in lipid metabolism and structure have been reported in diabetes. The rise in lipid peroxidation is also a sign of weakening of defense mechanisms against enzymatic and non-enzymatic antioxidants. Hyperglycaemia is closely related to elevated lipid peroxidation, oxidative stress in diabetes mellitus and diabetes-induced secondary chronic complications including atherosclerosis and neural disorders [19].
Protein oxidation: Proteins are vital biomolecules of the cell and are involved in majority of the physiological functions including cell signaling and transport across the cell membrane. Proteins are potential target of ROS and their structure and function can be affected by modification. Carbonyl proteins are the potent biomarker of oxidative stress and their elevated levels have been recorded in different cells and plasma of diabetic patients [20]. Advanced Oxidation Protein Products (AOPPs) are formed by reactions between plasma proteins and chlorinated oxidants. However, further studies are required to investigate their role as potential marker of protein oxidation during oxidative stress [21].
Effect of oxidative stress on β-cells death and dysfunction: The β-cells are essential for glucose homeostasis due to their ability to secrete insulin in response to nutrient uptake. These are among the most metabolically active tissues of the body that rely on oxidative phosphorylation for the generation of ATP and therefore plays an important role in insulin synthesis and secretion. ATP is synthesised by ATP synthase in mitochondria through coupling of the electrochemical H+ gradient to the oxidative phosphorylation of fuel (glucose or fatty acids). Glucose metabolism in β-cells leads to an elevated intracellular ATP:ADP ratio, which closes the ATP-sensitive K+ (KATP) channels, resulting in depolarization of the cell membrane that activates voltage-dependent Ca2+ channels, leading to Ca2+ entry and insulin release from the β-cells through exocytosis [22,23]. Aerobic cells produce ROS such as superoxide (O2-) and H2O2 during oxidative phosphorylation in mitochondria as by-products [24]. When ATP levels are appropriate in the cells, an uncoupling process dissipates the electrochemical H+ gradient, which plays an important role in reducing the level of ROS [25]. Uncoupling Proteins (UCP) play a physiological role in thermoregulation of the body. Activation of uncoupling proteins leads to inhibition of ATP synthesis and ATP mediated release of insulin from the β-cells of the pancreas. There are five UCP homologues present in mammals namely, UCP1, UCP2, UCP3, UCP4 and UCP5. UCP1 is expressed in brown adipose tissue. UCP2 is found in tissues including heart, kidney, liver, brain, pancreas and white adipose tissue while UCP3 is expressed in skeletal muscles and brown fat cells. However, the function and regulation of these UCPs remain unclear. Specifically, UCP2 appears to play a role in the regulation of ROS production, inflammation, cell proliferation and death of β-cells of the pancreas [26]. Moreover, common causes of ROS generation in β-cells are hyperglycaemia, hyperlipidemia, hypoxia and endoplasmic stress. Besides, β-cells contain only 50% of the SOD and 5% of H2O2 scavenging enzymes as compared to liver cells. Thus, β-cells are highly susceptible to oxidative damage. UCP2 expression negatively regulates the insulin secretion due to its uncoupling activity which reduces mitochondrial ATP synthesis. Acute exposure of β-cells to high concentrations of glucose and free fatty acids stimulates insulin secretion, whereas chronic exposure results in desensitisation and suppression of insulin secretion [27] [Table/Fig-2].
Proposed theory for UCP2 activation in β-cells.
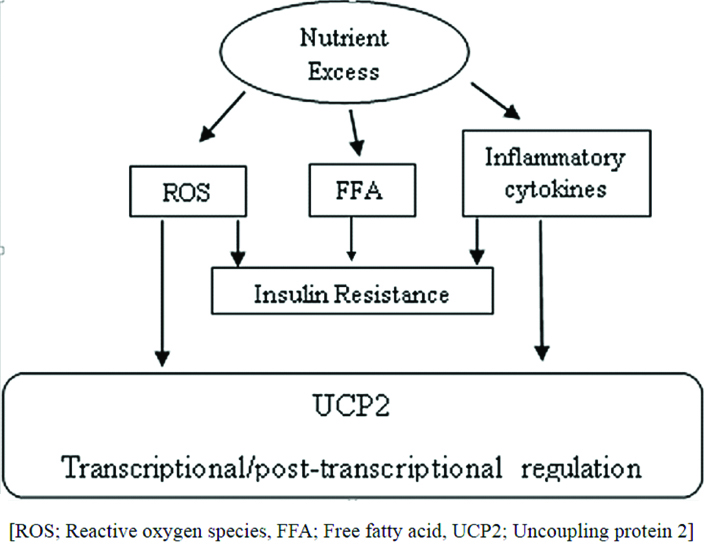
Effect of oxidative stress on β-cell proliferation: The number of β-cells in pancreas is usually constant after birth but some evidences indicate that under certain physiological or pathological conditions such as pregnancy, obesity or diabetes, these can regenerate. Some studies suggest that ROS, to some extent could stimulate β-cell regeneration. Forkhead box class O family member proteins (FOXOs) are transcription factors that regulate the β-cell proliferation and differentiation. Due to the increased production of ROS in β-cell, FoxO1 forms a complex with transcription factor along with promyelocyticleukaemia protein (Pml) and the NAD-dependent deacetylase sirtuin-1 (SIRT1), which helps in β-cell proliferation resulting in attenuation of β-cell development [Table/Fig-3] [28]. Elevated plasma glucose is clearly associated with induction of oxidative stress [11]. Moreover, recent study has established that lowering of the glucose concentration also reversibly increases ROS production in pancreatic β-cells [29]. These observations suggest that the production of ROS is both necessary and at the same time possibly hazardous for normal β-cell function [30].
Effect of oxidative stress on β-cell proliferation.
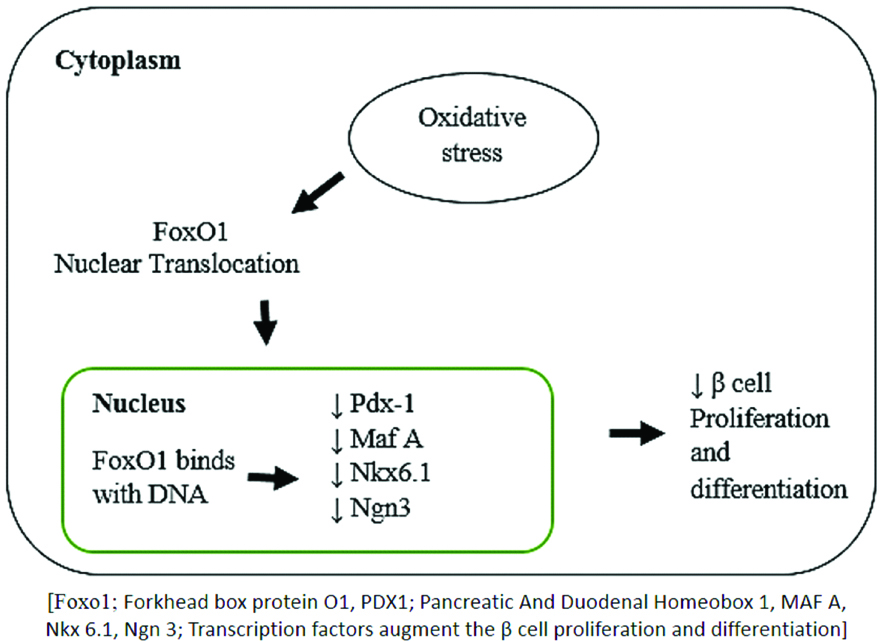
Diabetic Complications
Elevated blood glucose levels over prolonged period of time in uncontrolled diabetes mellitus can lead to a number of short and long-term macro and microvascular complications. Hyperglycaemia-induced oxidative stress in diabetic patients is the most explored hypotheses to explain the onset of complications. A study conducted in 2011 on 20,000 T2DM Indian patients showed the prevalence of various complications such as: neuropathy (33.6%), cardiovascular (23.6%), renal (21.1%), eye (16.6%) and foot ulcer (5.1%) [31].
Cardiovascular disease: There are ample evidences to show that patients with diabetes mellitus are at high risk for several Cardiovascular Disorders (CVDs). CVDs are the leading causes of diabetes-related morbidity and mortality [32]. Diabetes mellitus patients have elevated triglycerides, low HDL cholesterol and abnormalities in the structure of lipoprotein particles. The LDL cholesterol is predominantly present in small, dense form in diabetic patients and are more atherogenic than the large dense LDL particles present in healthy subjects because these can more easily penetrate which increases their susceptibility to oxidation and attachment to the arterial walls. Oxidised LDL is pro-atherogenic as it is recognised by the immune system as ‘foreign’, and causes several abnormal biological responses such as attracting leukocytes to the intima of vessel, improving the ability of leukocytes to ingest lipids and their differentiation into foam cells, and stimulating the proliferation of leukocytes, endothelial cells, and smooth muscle cells [33]. These episodes instigate the formation of atherosclerotic plaque. In diabetic patients, LDL particles can also become glycated that increases the half-life of LDL particles and therefore, increases the ability of the LDL to promote atherogenesis. Dyslipidemia in diabetes promotes endothelial dysfunction and affects vasodilatory, anti-atherogenic, and anti-inflammatory properties of healthy endothelium and thus leads to atherosclerosis [32].
Diabetic nephropathy: Diabetic nephropathy is a public health problem worldwide that affects millions of people. Diabetes is a leading cause of Chronic Kidney Disease (CKD). Approximately 43% of diabetes mellitus patients in the United States have microalbuminuria, a marker of progression of CKD [34]. Hyperglycaemia in diabetes mellitus causes progressive structural changes in mesangium, glomerular basement membrane, and tubulo-interstitial tissue of kidney and is considered as the driving factor for diabetic nephropathy. However, several other studies have demonstrated variability in the development of renal complications despite comparable hyperglycaemic control. The progression of diabetic nephropathy is complex and multifactorial [35]. Uncontrolled diabetes mellitus results in non-enzymatic interaction of glucose with amino acids, groups of proteins, lipoproteins, and nucleic acids that leads to the formation of Advanced Glycosylation End Products (AGE); which is associated with pathophysiology of diabetic nephropathy through mechanisms that are either receptor-dependent or receptor-independent. It modifies basement membrane proteins, cross-links extracellular components and increases expression of type IV collagen. These alterations lead to structural changes of the surface charge, membrane permeability, proteolytic digestion, and membrane stability and disrupt intercellular interactions. This leads to impairment of tissue function and maintenance [36]. AGEs interact with a wide array of receptors such as macrophage scavenger receptor type I and II, AGE-R1, AGE-R2, AGE-R3, Receptor for AGE (RAGE), and CD-36 on various cell types. RAGE activation leads to activation of several signal transduction pathways that lead to the generation of ROS and instigation of transcription factors, such as NF-kappa B that leads to the release of cytokines and growth factors including Transforming Growth Factor-β1 (TGF-β1), interleukin-1β and interleukin-6, insulin-like growth factor-1, Tumour Necrosis Factor (TNF-α), and platelet-derived growth factor that activate many pro-inflammatory conditions [35,37].
Diabetic neuropathy: A common complication of type 1 and type 2 diabetes is neuropathic pain. Distal Symmetrical Polyneuropathy (DSPN) is the most common clinical form of diabetic neuropathy. DSPN affects toes and distal foot and is found in more than 90% of the diabetic patients [38]. Diabetic Neuropathic Pain (DNP) is characterised by continuous tingling, burning, sharp, shooting, and lancinating pain, which can substantially affect the quality of life of the patients [39]. The pathogenesis of DNP is not fully understood but several risk factors are known to be associated with DNP including hyperglycaemia, older age, longer diabetes duration, drinking alcohol and cigarette smoking [38].
Diabetic Retinopathy: Chronic hyperglycaemia in diabetes mellitus triggers the several signaling pathways, including protein kinase C, activation of polyol pathway, elevated AGEs etc., that eventually lead to the functional and structural impairment to retinal cells [40]. Diabetic retinopathy is the most common microvascular complication of diabetes [41]. Currently, diabetic retinopathy affects almost 100 million people worldwide and is set to become an ever-increasing health burden. It falls into two categories: the earlier stage of Non-Proliferative Diabetic Retinopathy (NPDR) and the advanced stage of Proliferative Diabetic Retinopathy (PDR). The clinical features in NPDR patients are microaneurysm, retinal haemorrhage and intraretinal microvascular abnormalities, while PDR is characterised by the hallmark feature of pathologic pre-retinal neovascularization [42]. A major additional categorization in diabetic retinopathy is diabetic macular edema, which is an important manifestation that characterises the most common cause of vision loss in patients with diabetic retinopathy.
Diabetic foot ulcers: Approximately 6% of people with diabetes are affected by foot disease, that includes infection, ulceration, or destruction of tissues of the foot [43]. It can damage patients’ quality of life and affect social participation and livelihood.
Oxidative stress as a therapeutic target for management of diabetes mellitus: Oxidative stress is associated with many diseases linked with metabolic or vascular disorders. Thus, diabetes represents an ideal candidate for studying the significance of oxidative stress and its implication as potential target for treatment of the disease. Inhibiting the free radical formation by antioxidants serves as a novel therapeutic strategy to reduce oxidative stress and prevent diabetes-related vascular complications. The therapeutic efficacy of antioxidants has been evaluated in several randomised controlled trials and the study outcomes are summarised in [Table/Fig-4] [44-61]. Many adjuvants with strong pharmacotherapy potential for diabetes management have been shown to possess antioxidant properties in addition to their primary pharmacological actions. The antioxidant properties of these agents may be the contributing factor for their therapeutic efficacy.
Summary of randomised controlled trial on antioxidant therapy in diabetes mellitus patients [44-61].
Study | Study outcome | Reference |
---|
T2DM patients with symptomatic peripheral neuropathy (n=328) | Alpha-lipoic acid was effective in reducing neuropathic symptoms. | [44] |
30-year follow-up survey (n=338) | Inverse relationship between Vitamin C consumption and onset of T2DM. | [45] |
Diabetic patients were supplemented with alpha-tocopherol capsules (orally, 100 IU/d) or placebo (n=35) | Vitamin E supplementation significantly lowered lipid peroxidation and lipid levels in diabetic patients. | [46] |
T2DM patients infused with vitamin C (n=10) | Endothelial dysfunction in T2DM patients improved. | [47] |
T2DM patients (n=74) | Oral administration of lipoic acid significantly increased insulin-mediated glucose uptake (Improved insulin sensitivity). | [48] |
Type 1 and Type 2 diabetic patients with symptomatic polyneuropathy (n=50) | Antioxidant thioctic acid has beneficial effect on several attributes of nerve conduction. | [49] |
T2DM patients were given vitamin C (1250 mg) and vitamin E (680 IU) per day or matching placebo (n=30) | Short-term treatment with vitamin C and E in pharmacological doses lowered the rate of albumin excretion in T2DM patients with micro/macroalbuminuria. | [50] |
T2DM patients received alpha-tocopherol (200 mg/day) (n=98) | Alpha-tocopherol supplementation was beneficial in decreasing blood lipid peroxide concentrations. | [51] |
Diabetes subjects were enrolled in treatment group [aspirin (100 mg/day) and vitamin E (300 mg/day)] and placebo group (n=1,031). | No beneficial effects were reported in prevention of cardiovascular disease in diabetic patients. | [52] |
Diabetic subjects were randomised to receive vitamin C (1,000 mg) and vitamin E (800 IU) daily or matching placebo (n=49) | Oral antioxidant therapy improved endothelium-dependent vasodilation in type 1 but not in type 2 diabetes. | [53] |
T2DM patients received 1000 mg of vitamin C daily (n=84) | Showed beneficial effects in T2DM patients by decreasing blood glucose and lipids and thus reducing the risk of complications. | [54] |
Diabetes mellitus patients were randomised to vitamin E (400 U/day) or placebo (n=1434) | Reduced the cardiovascular events in individuals with diabetes mellitus. | [55] |
Adults aged 40 years or more with type 1 or type 2 diabetes were supplemented with tocopherol (n=1276) | Revealed no significant beneficial effects. | [56] |
Middle-aged male smokers received either vitamin E (50 U/day) or β-carotene (20 mg/day), or both, or placebo for a median of 6.1 years (n=29,133) | At base line, 1700 men had type 2 diabetes, of which, 662 were diagnosed with first ever macrovascular complication, and 1142 died during the 19-year follow-up. Alpha-tocopherol or β-carotene supplementation has no protective effect on macrovascular outcomes or total mortality of diabetic male smokers. | [57] |
T2DM patients were supplemented with vitamin C (n=70) | Oral supplementation of vitamin C with metformin reverses ascorbic acid levels, reduces FBS, and improves HbA1c. | [58] |
Effects of vitamin C supplementation (1g/day) among diabetic patients (n=30) | Vitamin C was effective in improving hyperglycaemia and hyperlipidemia | [59] |
Newly diagnosed T2DM patients treated with vitamin C and vitamin E (n=34). | Antioxidant therapy with vitamin C and vitamin E in addition to oral hypoglycaemic agent reduced oxidative stress in patients. | [60] |
Patients with newly diagnosed type 2 diabetes and supplemented with vitamin C (n=90). | Vitamin C in a dose of 2g/day as an add on therapy to oral hypoglycaemic agent produced improvement in lipid profile to desirable levels and thus reducing the risk of diabetic complications | [61] |
Conclusion
Free radical production in body is a continuous process as part of normal function. Though, excess free radical production instigating from endogenous or exogenous sources might play a role in onset of diabetes and its complications. Oxidative stress in diabetes causes over production of mitochondrial superoxide in endothelial cells of both large and small vessels, as well as in the myocardium and leads to many micro and macro-vascular complications. The inhibition and scavenging of reactive oxygen species and reactive nitrogen species formation offer novel therapeutic strategy for management of oxidative stress and prevention of subsequent pathologic complications associated with diabetes mellitus. Antioxidants play important role in neutralisation of free radicals and reduce the associated tissue damage by scavenging them and promote their decomposition. Therefore, supplementation of antioxidants may be a valuable strategy for controlling diabetes complications and enhancing antioxidant capacity. Despite the potential advantages of antioxidant pharmacotherapy, additional systematic randomised trial is required to explore and assess the efficacy and safety scores of the current therapeutic strategy. In future, more research into the pathophysiology of oxidative stress and well-planned antioxidant therapy, especially on mechanistic approach in diabetic patients will be the main target area of research to get any fruitful conclusion.
Conflict of interest: The authors declare no conflict of interest regarding the publication of this paper.
[1]. American Diabetes AssociationDiagnosis and classification of diabetes mellitus Diabetes Care 2010 33:S62-69.10.2337/dc10-S06220042775 [Google Scholar] [CrossRef] [PubMed]
[2]. Hales C, Barker D, Type 2 (non-insulin-dependent) diabetes mellitus: the thrifty phenotype hypothesis Int J Epidemiol 2013 42:1215-22.10.1093/ije/dyt13324159065 [Google Scholar] [CrossRef] [PubMed]
[3]. American Diabetes Association ADClassification and diagnosis of diabetes: Standards of medical care in diabetes-2018 Diabetes Care 2018 41:S13-27.10.2337/dc18-S00229222373 [Google Scholar] [CrossRef] [PubMed]
[4]. WHO. Definition, diagnosis and classification of diabetes mellitus and its complications: report of a WHO consultation. Part 1, Diagnosis and classification of diabetes mellitus. Geneva: 1999 [Google Scholar]
[5]. Kaveeshwar SA, Cornwall J, The current state of diabetes mellitus in India Australas Med J 2014 7:45-48.10.4066/AMJ.2014.1979 [Google Scholar] [CrossRef]
[6]. Anjana RM, Pradeepa R, Deepa M, Datta M, Sudha V, Unnikrishnan R, Prevalence of diabetes and prediabetes (impaired fasting glucose and/or impaired glucose tolerance) in urban and rural India: Phase I results of the Indian Council of Medical Research-INdia DIABetes (ICMR-INDIAB) study Diabetologia 2011 54:3022-27.10.1007/s00125-011-2291-521959957 [Google Scholar] [CrossRef] [PubMed]
[7]. IDF Diabetes Atlas. 2017. International Diabetes Fedration 8th ed [Google Scholar]
[8]. Kido Y, Burks DJ, Withers D, Bruning JC, Kahn CR, White MF, Tissue-specific insulin resistance in mice with mutations in the insulin receptor, IRS-1, and IRS-2 J Clin Invest 2000 105:199-205.10.1172/JCI791710642598 [Google Scholar] [CrossRef] [PubMed]
[9]. Pessin JE, Thurmond DC, Elmendorf JS, Coker KJ, Okada S, Molecular basis of insulin-stimulated GLUT4 vesicle trafficking J Biol Chem 1999 274:2593-96.10.1074/jbc.274.5.25939915783 [Google Scholar] [CrossRef] [PubMed]
[10]. Asmat U, Abad K, Ismail K, Diabetes mellitus and oxidative stress-A concise review Saudi Pharm J 2016 24:547-53.10.1016/j.jsps.2015.03.01327752226 [Google Scholar] [CrossRef] [PubMed]
[11]. Pham-Huy LA, He H, Pham-Huy C, Free radicals, antioxidants in disease and health Int J Biomed Sci 2008 4:89-96. [Google Scholar]
[12]. Freitas M, Gomes A, Porto G, Fernandes E, Nickel induces oxidative burst, NF-κB activation and interleukin-8 production in human neutrophils JBIC J Biol Inorg Chem 2010 15:1275-83.10.1007/s00775-010-0685-320632048 [Google Scholar] [CrossRef] [PubMed]
[13]. Henriksen EJ, Diamond-Stanic MK, Marchionne EM, Oxidative stress and the etiology of insulin resistance and type 2 diabetes Free Radic Biol Med 2010 51:993-99.10.1016/j.freeradbiomed.2010.12.00521163347 [Google Scholar] [CrossRef] [PubMed]
[14]. Maritim AC, Sanders RA, Watkins JB, Diabetes, oxidative stress, and antioxidants: A review J Biochem Mol Toxicol 2003 17:24-38.10.1002/jbt.1005812616644 [Google Scholar] [CrossRef] [PubMed]
[15]. Lugrin J, Rosenblatt-Velin N, Parapanov R, Liaudet L, The role of oxidative stress during inflammatory processes Biol Chem 2014 395:203-30.10.1515/hsz-2013-024124127541 [Google Scholar] [CrossRef] [PubMed]
[16]. Brownlee M, The pathobiology of diabetic complications: a unifying mechanism Diabetes 2005 54:1615-25.10.2337/diabetes.54.6.161515919781 [Google Scholar] [CrossRef] [PubMed]
[17]. Ayepola OR, Brooks NL, Oguntibeju OO, Oxidative Stress and Diabetic Complications: The Role of Antioxidant Vitamins and Flavonoids InTech 2014 [Google Scholar]
[18]. Guo L, Chen Z, Amarnath V, Davies SS, Identification of novel bioactive aldehyde-modified phosphatidylethanolamines formed by lipid peroxidation Free Radic Biol Med 2012 53:1226-38.10.1016/j.freeradbiomed.2012.07.07722898174 [Google Scholar] [CrossRef] [PubMed]
[19]. Ramesh B, Karuna R, Sreenivasa RS, Haritha K, Sai MD, Sasis BRB, Effect of Commiphora mukul gum resin on hepatic marker enzymes, lipid peroxidation and antioxidants status in pancreas and heart of streptozotocin induced diabetic rats Asian Pac J Trop Biomed 2012 2:895-900.10.1016/S2221-1691(12)60249-4 [Google Scholar] [CrossRef]
[20]. Suzuki D, Miyata T, Carbonyl Stress in the Pathogenesis of Diabetic Nephropathy Intern Med 1999 38:305-14.10.2169/internalmedicine.38.30910361902 [Google Scholar] [CrossRef] [PubMed]
[21]. Savini I, Catani M, Evangelista D, Gasperi V, Avigliano L, Obesity-associated oxidative stress: Strategies finalized to improve redox state Int J Mol Sci 2013 14:10497-538.10.3390/ijms14051049723698776 [Google Scholar] [CrossRef] [PubMed]
[22]. Koster JC, Permutt MA, Nichols CG, Diabetes and insulin secretion: the ATP-sensitive K+ channel (K ATP) connection Diabetes 2005 54:3065-72.10.2337/diabetes.54.11.306516249427 [Google Scholar] [CrossRef] [PubMed]
[23]. Garlid KD, Jabůrek M, Jezek P, Varecha M, How do uncoupling proteins uncouple? Biochim Biophys Acta 2000 1459:383-89.10.1016/S0005-2728(00)00175-4 [Google Scholar] [CrossRef]
[24]. Forman HJ, Torres M, Reactive oxygen species and cell signaling Am J Respir Crit Care Med 2002 166:S4-8.10.1164/rccm.220600712471082 [Google Scholar] [CrossRef] [PubMed]
[25]. Liang J, Wu SY, Zhang D, Wang L, Leung KK, Leung PS, NADPH oxidase-dependent reactive oxygen species stimulate β-cell regeneration through differentiation of endocrine progenitors in murine pancreas Antioxid Redox Signal 2016 24:419-33.10.1089/ars.2014.613526464216 [Google Scholar] [CrossRef] [PubMed]
[26]. Saleh MC, Wheeler MB, Chan CB, Uncoupling protein-2: evidence for its function as a metabolic regulator Diabetologia 2002 45:174-87.10.1007/s00125-001-0737-x11935148 [Google Scholar] [CrossRef] [PubMed]
[27]. Nolan CJ, Madiraju MSR, Delghingaro-Augusto V, Peyot M-L, Prentki M, Fatty Acid Signaling in the β-Cell and Insulin Secretion Diabetes 2006 55:S16-23.10.2337/db06-S00317130640 [Google Scholar] [CrossRef] [PubMed]
[28]. Roma LP, Duprez J, Takahashi HK, Gilon P, Wiederkehr A, Jonas J-C, Dynamic measurements of mitochondrial hydrogen peroxide concentration and glutathione redox state in rat pancreatic β-cells using ratiometric fluorescent proteins: confounding effects of pH with HyPer but not roGFP1 Biochem J 2012 441:971-78.10.1042/BJ2011177022050124 [Google Scholar] [CrossRef] [PubMed]
[29]. Robertson RP, Harmon JS, Pancreatic islet β-cell and oxidative stress: The importance of glutathione peroxidase FEBS Lett 2007 581:3743-48.10.1016/j.febslet.2007.03.08717433304 [Google Scholar] [CrossRef] [PubMed]
[30]. Pi J, Zhang Q, Fu J, Woods CG, Hou Y, Corkey BE, ROS signaling, oxidative stress and Nrf2 in pancreatic beta-cell function Toxicol Appl Pharmacol 2010 244:77-83.10.1016/j.taap.2009.05.02519501608 [Google Scholar] [CrossRef] [PubMed]
[31]. Mohan V, Shah S, Saboo B, Current glycaemic status and diabetes related complications among type 2 diabetes patients in India: data from the A1chieve study J Assoc Physicians India 2013 61:12-15. [Google Scholar]
[32]. Orasanu G, Plutzky J, The Pathologic Continuum of Diabetic Vascular Disease J Am Coll Cardiol 2009 53:S35-42.10.1016/j.jacc.2008.09.05519179216 [Google Scholar] [CrossRef] [PubMed]
[33]. Celermajer DS, Endothelial dysfunction: does it matter? Is it reversible? J Am Coll Cardiol 1997 30:325-33.10.1016/S0735-1097(97)00189-7 [Google Scholar] [CrossRef]
[34]. Bohlender JM, Franke S, Stein G, Wolf G, Advanced glycation end products and the kidney Am J Physiol Physiol 2005 289:F645-59.10.1152/ajprenal.00398.200416159899 [Google Scholar] [CrossRef] [PubMed]
[35]. Tan ALY, Forbes JM, Cooper ME, AGE, RAGE, and ROS in diabetic nephropathy Semin Nephrol 2007 27:130-43.10.1016/j.semnephrol.2007.01.00617418682 [Google Scholar] [CrossRef] [PubMed]
[36]. Miura J, Yamagishi S, Uchigata Y, Takeuchi M, Yamamoto H, Makita Z, Serum levels of non-carboxymethyl lysine advanced glycation endproducts are correlated to severity of microvascular complications in patients with Type 1 diabetes J Diabetes Complications 2003 17:16-21.10.1016/S1056-8727(02)00183-6 [Google Scholar] [CrossRef]
[37]. Braga Gomes K, Fontana Rodrigues K, Fernandes AP, The role of transforming growth factor-beta in diabetic nephropathy Int J Med Genet 2014 2014:1-6.10.1155/2014/180270 [Google Scholar] [CrossRef]
[38]. Tesfaye S, Boulton AJM, Dickenson AH, Mechanisms and management of diabetic painful distal symmetrical polyneuropathy Diabetes Care 2013 36:2456-65.10.2337/dc12-196423970715 [Google Scholar] [CrossRef] [PubMed]
[39]. Bansal V, Kalita J, Misra UK, Diabetic neuropathy Postgrad Med J 2006 82:95-100.10.1136/pgmj.2005.03613716461471 [Google Scholar] [CrossRef] [PubMed]
[40]. Safi SZ, Qvist R, Kumar S, Batumalaie K, Ismail IS Bin, Molecular mechanisms of diabetic retinopathy, general preventive strategies, and novel therapeutic targets Biomed Res Int 2014 2014:80126910.1155/2014/80126925105142 [Google Scholar] [CrossRef] [PubMed]
[41]. Antonetti DA, Klein R, Gardner TW, Diabetic retinopathy N Engl J Med 2012 366:1227-39.10.1056/NEJMra100507322455417 [Google Scholar] [CrossRef] [PubMed]
[42]. Stitt AW, Curtis TM, Chen M, Medina RJ, McKay GJ, Jenkins A, The progress in understanding and treatment of diabetic retinopathy Prog Retin Eye Res 2016 51:156-86.10.1016/j.preteyeres.2015.08.00126297071 [Google Scholar] [CrossRef] [PubMed]
[43]. Lazzarini PA, Hurn SE, Fernando ME, Jen SD, Kuys SS, Kamp MC, Prevalence of foot disease and risk factors in general inpatient populations: a systematic review and meta-analysis BMJ Open 2015 5:e00854410.1136/bmjopen-2015-00854426597864 [Google Scholar] [CrossRef] [PubMed]
[44]. Ziegler D, Hanefeld M, Ruhnau KJ, Meissner HP, Lobisch M, Schütte K, Treatment of symptomatic diabetic peripheral neuropathy with the anti-oxidant alpha-lipoic acid. A 3-week multicentre randomized controlled trial (ALADIN Study) Diabetologia 1995 38:1425-33.10.1007/BF004006038786016 [Google Scholar] [CrossRef] [PubMed]
[45]. Feskens EJ, Virtanen SM, Räsänen L, Tuomilehto J, Stengård J, Pekkanen J, Dietary factors determining diabetes and impaired glucose tolerance. A 20-year follow-up of the Finnish and Dutch cohorts of the Seven Countries Study Diabetes Care 1995 18:1104-12.10.2337/diacare.18.8.11047587845 [Google Scholar] [CrossRef] [PubMed]
[46]. Jain SK, McVie R, Jaramillo JJ, Palmer M, Smith T, Meachum ZD, The effect of modest vitamin E supplementation on lipid peroxidation products and other cardiovascular risk factors in diabetic patients Lipids 1996 31(Suppl)10.1007/BF026370578729100 [Google Scholar] [CrossRef] [PubMed]
[47]. Ting HH, Timimi FK, Boles KS, Creager SJ, Ganz P, Creager MA, Vitamin C improves endothelium-dependent vasodilation in patients with non-insulin-dependent diabetes mellitus J Clin Invest 1996 97:22-28.10.1172/JCI1183948550838 [Google Scholar] [CrossRef] [PubMed]
[48]. Jacob S, Ruus P, Hermann R, Tritschler HJ, Maerker E, Renn W, Oral administration of RAC-alpha-lipoic acid modulates insulin sensitivity in patients with type-2 diabetes mellitus: a placebo-controlled pilot trial Free Radic Biol Med 1999 27:309-14.10.1016/S0891-5849(99)00089-1 [Google Scholar] [CrossRef]
[49]. Reljanovic M, Reichel G, Rett K, Lobisch M, Schuette K, Möller W, Treatment of diabetic polyneuropathy with the antioxidant thioctic acid (alpha-lipoic acid): a two year multicenter randomized double-blind placebo-controlled trial (ALADIN II). Alpha Lipoic Acid in Diabetic Neuropathy Free Radic Res 1999 31:171-79.10.1080/1071576990030072110499773 [Google Scholar] [CrossRef] [PubMed]
[50]. Gaede P, Poulsen HE, Parving HH, Pedersen O, Double-blind, randomised study of the effect of combined treatment with vitamin C and E on albuminuria in Type 2 diabetic patients Diabet Med 2001 18:756-60.10.1046/j.0742-3071.2001.00574.x11606175 [Google Scholar] [CrossRef] [PubMed]
[51]. Park S, Choi SB, Effects of α-tocopherol supplementation and continuous subcutaneous insulin infusion on oxidative stress in Korean patients with type 2 diabetes Am J Clin Nutr 2002 75:728-33.10.1093/ajcn/75.4.72811916760 [Google Scholar] [CrossRef] [PubMed]
[52]. Sacco M, Pellegrini F, Roncaglioni MC, Avanzini F, Tognoni G, Nicolucci A, Primary prevention of cardiovascular events with low-dose aspirin and vitamin E in type 2 diabetic patients: results of the Primary Prevention Project (PPP) trial Diabetes Care 2003 26:3264-72.10.2337/diacare.26.12.326414633812 [Google Scholar] [CrossRef] [PubMed]
[53]. Beckman J, Goldfine A, Oral antioxidant therapy improves endothelial function in Type 1 but not Type 2 diabetes mellitus Am J Physiol Hear Circ Physiol 2003 10:1-8.10.1152/ajpheart.00403.200312881209 [Google Scholar] [CrossRef] [PubMed]
[54]. Afkhami-Ardekani M, Shojaoddiny-Ardekani A, Effect of vitamin C on blood glucose, serum lipids & serum insulin in type 2 diabetes patients Indian J Med Res 2007 126:471-74. [Google Scholar]
[55]. Milman U, Blum S, Shapira C, Aronson D, Miller-Lotan R, Anbinder Y, Vitamin E supplementation reduces cardiovascular events in a subgroup of middle-aged individuals with both type 2 diabetes mellitus and the haptoglobin 2-2 genotype: A prospective double-blinded clinical trial Arterioscler Thromb Vasc Biol 2007 28:341-47.10.1161/ATVBAHA.107.15396518032779 [Google Scholar] [CrossRef] [PubMed]
[56]. Belch J, MacCuish A, Campbell I, Cobbe S, Taylor R, Prescott R, The prevention of progression of arterial disease and diabetes (POPADAD) trial: factorial randomised placebo controlled trial of aspirin and antioxidants in patients with diabetes and asymptomatic peripheral arterial disease BMJ 2008 337:a184010.1136/bmj.a184018927173 [Google Scholar] [CrossRef] [PubMed]
[57]. Kataja-Tuomola MK, Kontto JP, Männistö S, Albanes D, Virtamo JR, Effect of alpha-tocopherol and beta-carotene supplementation on macrovascular complications and total mortality from diabetes: Results of the ATBC Study Ann Med 2010 42:178-86.10.3109/0785389090350888720350251 [Google Scholar] [CrossRef] [PubMed]
[58]. Dakhale GN, Chaudhari HV, Shrivastava M, Supplementation of vitamin C reduces blood glucose and improves glycosylated hemoglobin in type 2 diabetes mellitus: a randomized, double-blind study Adv Pharmacol Sci 2011 2011:19527110.1155/2011/19527122242019 [Google Scholar] [CrossRef] [PubMed]
[59]. Vaksh S, Pandey M, Zingade US, Seshadri Reddy V, The effect of vitamin-C therapy on hyperglycaemia, hyperlipidemia and non high density lipoprotein level in type 2 diabetes mellitus Int J LifeSc Bt Pharm 2013 2013:1-8. [Google Scholar]
[60]. Desai NK, Bhabhor PH, Domadia D, Bhatt J D, Role of antioxidant therapy in management of type-2 diabetes mellitus Natl J Integr Res Med 2013 4:128-33. [Google Scholar]
[61]. Nayaka R, A comparative trial on the efficacy of vitamin C as add on therapy to the oral hypoglycaemic agent on serum lipid level in newly diagnosed type 2 diabetes mellitus IJPR 2015 5:115 [Google Scholar]