Manual Weight Lifting (MWL) is an unavoidable task that needs to be performed in every profession along with daily schedule of household activities. Health hazards due to MWL are established facts pertaining to almost every industrial sector [1-5]. Past studies established LBP as the most frequent occupational health risk caused by ‘lifting weight’ [6-10]. According to Punnett Let al., an estimated 37% cases out of total LBP found in adults were attributed to occupational exposures resulting in an annual loss of about 818,000 disability-adjusted life years worldwide [11]. The injury risks due to MWL have been a topic of interest since long and previous studies [12-15], have either examined the effects of MWL by carrying out real-time human trials or used biomechanical models for assessing the MWL activities. In last 5-6 decades, many researchers designed 2D biomechanical prediction models to assess biomechanical stress due to MWL tasks [1,14-18]. Shearing and compressive forces of lower lumbar spine during simulated Manual Material Handling (MMH) tasks [14] were assessed and maximum strength applied to lift objects while assuming different postures were predicted [15]. Clincinnati OH et al., developed models to evaluate biomechanical hazards due to MMH [1], worked on prediction of load lifting limits [16] and compared between static and dynamic evaluation of biomechanical stress due to lifting [17]. They also carried out biomechanical evaluation of five lifting techniques through estimation of predicted L5/S1 disc compression force and low back strain, strengths of shoulder, hip and knee joints [18]. These studies eventually led to development of both static and dynamic biomechanical models [19,20] to evaluate lifting.
Globally, no reported studies are available on simultaneous evaluation of real-time kinetic responses along with 2D prediction biomechanics for identifying an optimised combination of MWL tasks with least physical workload for any population. It was hypothesised that for lifting even lower load magnitudes at lower frequency of lift and lifting height would be an important criterion where trunk forward bending is more. It was further hypothesised that the 2D predicted kinetics data would corroborate with the results of 3D realtime kinetics data simultaneously collected while undertaking given MWL tasks. Accordingly, the present study was designed to investigate the effects of lifting heights, weights and frequencies on kinetic responses for identifying an optimum combination of load-height-frequency for lifting. Simultaneously, the data generated would also be used to predict the risk of LBP in terms of TCF and TSF for the MWL activities with reference to the NIOSH 1980 guidelines and to validate the predicted 2D kinetic responses with the real-time kinetic data [25].
Materials and Methods
A cross-sectional pilot study was conducted at the Ergonomics laboratory of Defence Institute of Physiology and Allied Sciences (DIPAS), Delhi, India, during May-June 2015, which was supposed to look into different aspects of MWL simultaneously, including real-time kinematics, kinetics, electromyography, physiology along with 2D predicted kinematics and kinetics on large number of subjects. Currently reported study was the pilot study carried out on eight participants only, as observed in past researches [26], to understand the trend of data.
Participants
The inclusion criteria for the study were that the participants should be young, healthy adults between age range 20-30 years without prior exposure to load lifting tasks and exclusion criteria were history of surgery or incidents of musculoskeletal disorders. Eight (8) randomly selected physically fit and healthy young adult University students volunteered for the study.
Instrumentation
Hand grip strength was recorded with Hand Grip Dynamometer (M/s Jamer, USA). Videos were recorded with Sony Handy Cam PD170 and still photographs were extracted and analysed using Ergonomics evaluation software (ErgoMaster) to get 2D prediction kinetics [27]. Kistler Force Plate (model no. 9286 AA, Kistler Instrument AG, Winterthur, Switzerland) along with BioWare (R) software {Type 2812A1-3, version 3.24 (7648)} was used to collect realtime kinetic data at sampling rate of 200 Hz [28].
Experimental Protocol
The experimental protocol approved by the Institutional Ethics Committee [Ref. No: IEC/DIPAS/D-1/2] was explained to each of the participants and they were familiarised with the laboratory condition, experimental design and data collection procedure. Informed consent was signed by them before commencement of the study.
The volunteers reported one hour prior to the experiment, i.e., at 0900 h after partaking of light food at least one hour before coming to the laboratory. Basic information like name, height and weight were noted and Hand Grip Strengths were recorded.
Each participant lifted 10 kg and 20 kg loads at frequency of one lift per minute and four lifts per minute, through different heights, i.e., Floor to Knuckle (F-K), Knuckle to Shoulder (K-S) and Floor to Shoulder (F-S), respectively, standing on a force plate. Videography was accomplished with Sony Handy Cam and still photographs were extracted at predetermined positions during the lifting experiments. Lifting tasks were performed on a wooden rack with two wooden shelves corresponding to average knuckle and shoulder heights of the participants (72.0 cm, 141.0 cm, respectively). Total number of 30 trials was performed by each volunteer, including three repetitions for one lift per minute and two repetitions for four lifts per minute, as given below:
[{(2 Weight×3 Height)=6 Trials×3 Repetition for one lift per minute}=18 Trials]+[{(2 Weight×3 Height)=6 Trials×2 Repetition for four lift per minute}=12 Trials]=30 Trials
Data Analysis
Real-time kinetic data including Vertical Ground Reaction Force (VGRF), Anterior Posterior Moment (M A-P), Work and Power from force platform was processed in BioWare (R) software and exported to excel worksheet. For statistical analysis, peak values obtained in each trial was used. The average value obtained from three trials for one lift per minute and two trials for four lifts per minute were used.
Images extracted from videos for each trial were analysed to obtain prediction kinetics (i.e., Total Compressive Forces, Total Shearing Forces, Compressive Force due to Load, Shearing Force due to Load exerted on L5/S1 disc of spine) from Lift Analysis tool of ErgoMaster software [Table/Fig-1]. To achieve this, information like volunteers’ height, weight and lifting distance were keyed in manually. Four frames at different points on timeline for 3rd trial of one lift per minute were used. The frequency of lifting of four lifts per minute included four times lifting in one minute, which was considered as one trial. This trial was repeated twice at an interval of five minutes i.e., number of repetition was two. In order to get maximum response due to MWL under this condition, the 2D images at four different points on timeline were extracted from 4th lift of 2nd repetition of four lift per minute.
Prediction tool of ErgoMaster® software for 2D image analysis.
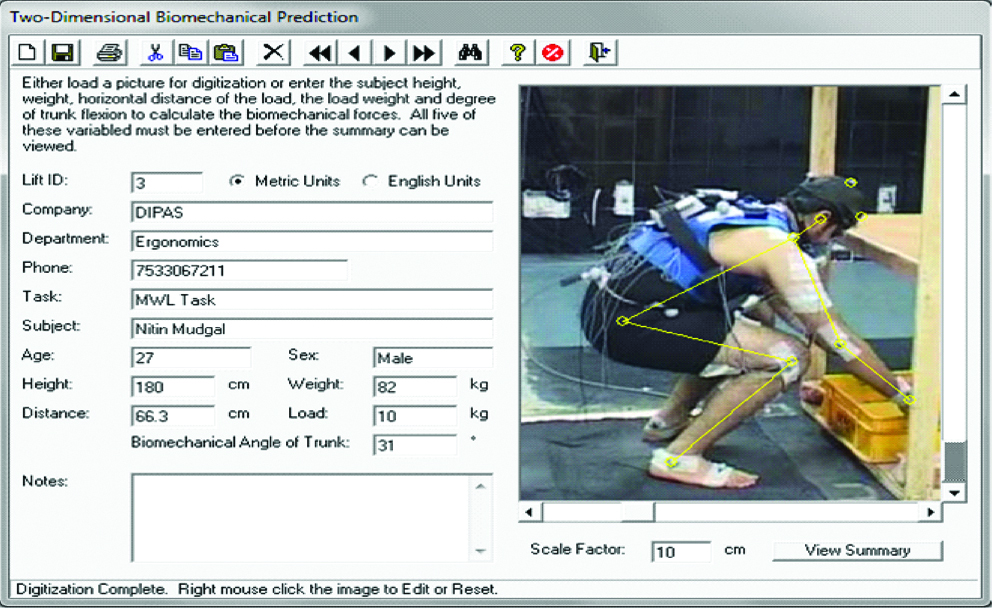
A total of 48 photographs were processed for each volunteer as given below:
{(2 Weights×2 Frequencies×3 Heights)=12 Trials×4 Frames per trial}=48 Photographs for each volunteer
According to National Institute of Occupational Safety and Health (NIOSH) guideline [25] for a given population, maximum acceptable weight for manual lifting is the load that gives rise to disc compressions equivalent to 3425 N as TCF and 500 N as TSF. In the present study, taking this value of 3425 N for TCF and 500 N for TSF as the 100% of spinal loading that is permitted, the excess value of TCF and TSF on L5/S1 were computed and further the percentage of the excess TCF and TSF values out of the value 3425 N and 500 N were calculated [29,30].
Statistical Analysis
The data were analysed using the Statistical Package for Social Sciences (SPSS) for Windows version 21.0 (M/s SPSS Inc., Chicago, IL, USA). All descriptive statistics were presented as mean values and standard error of mean (SEM). Three ways repeated measure analysis of variance (ANOVA) for all the parameters followed by Bonferroni post-hoc test was applied for the pair wise comparison of main effect within group. A value of p≤0.05 was considered as statistically significant.
Results
Physical characteristics of the participants are given in [Table/Fig-2]. The real-time kinetics and predicted kinetics data are presented in [Table/Fig-3,4,5,6 and 7] indicating significant changes in responses. Responses of a higher magnitude of load weight, heights and frequencies of lifts were compared against that of lower load weight, heights and frequencies of lifts.
Physical characteristics (mean±SD) of volunteers (n=8).
Physical Characteristics | Mean±SD (n=8) |
---|
Age (years) | 24.5±2.20 |
Height (cm) | 173.8±3.96 |
Weight (kg) | 73.9±8.31 |
Hand Grip Strength (right) (kg) | 44.0±4.41 |
Hand Grip Strength (left) (kg) | 38.9±3.56 |
Effect of load lifting with varying heights and frequencies on (a) VGRF (b) Moment A-P (c) Work and (d) Power.
Values are presented in Mean±SEM
Significance levels: *p≤0.001; $p=0.03 and p=0.04; #p=0.002; @p=0.03
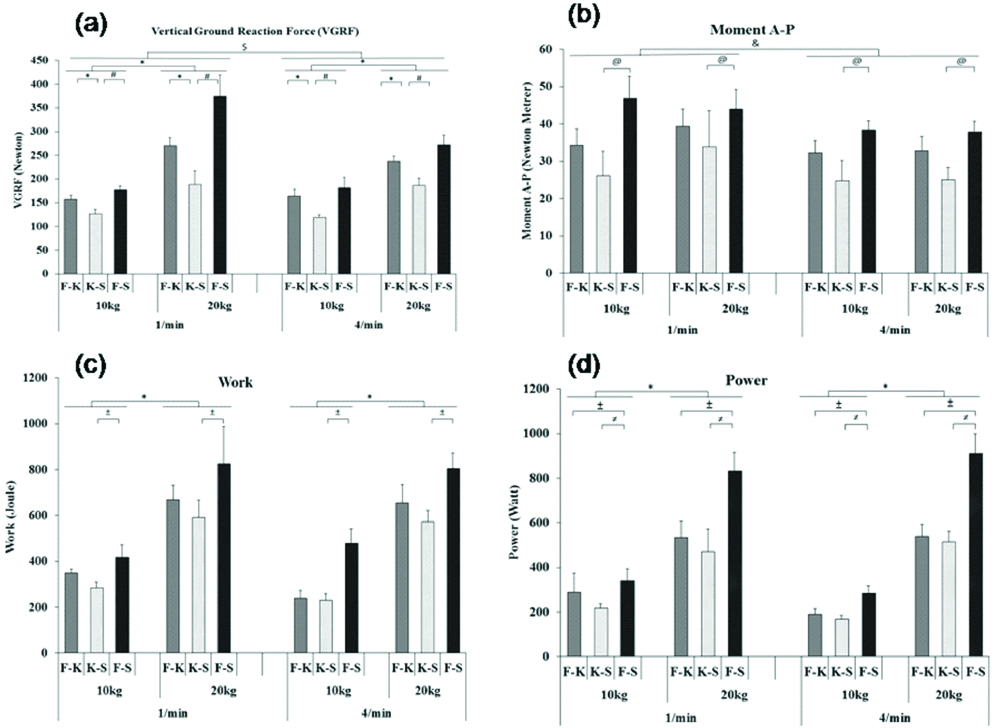
Effect of load lifting with varying height and frequencies on (a) TCF (b) CF-L (c) TSF and (d) SF-L.
Values are presented in Mean±SEM
Significance levels: *p≤0.001; #p=0.003 and p=0.004
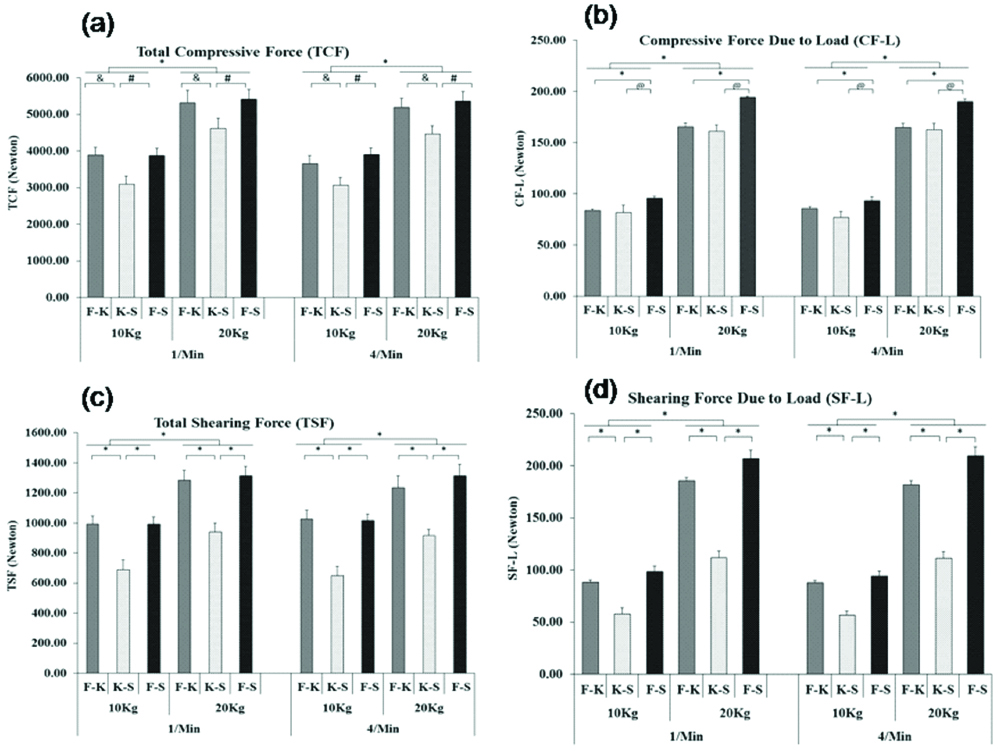
Effect of load lifting with varying heights and frequencies on (a) % age of higher TCF than acceptable limit (b) % age of higher TSF than acceptable limit.
Significance levels: *p≤0.001; #p=0.003; and : p=0.004
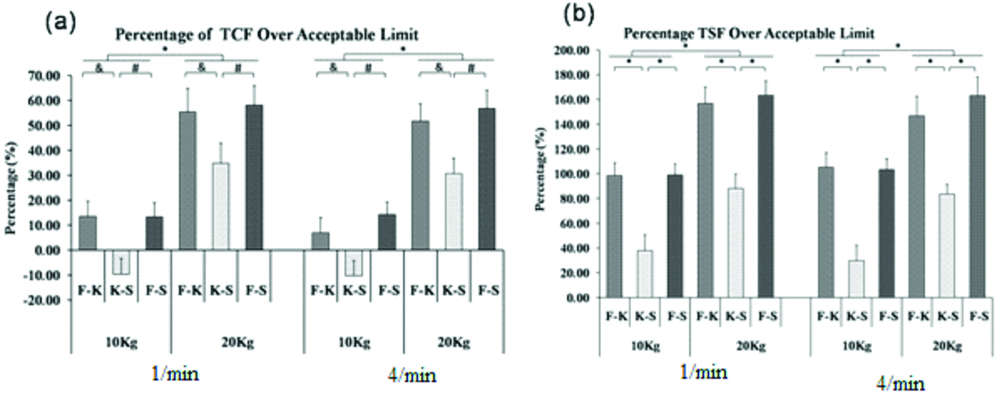
Statistically significant parameters for MWL with different weight, frequency and height of lift conditions.
Parameters | Weight | Frequency | Height |
---|
Degrees of Freedom | F-value | p-value | Degrees of Freedom | F-value | p-value | Degrees of Freedom | F-value | p-value |
---|
VGRF | 1,7 | 122.797 | ≤0.001 | 1,7 | 7.345 | 0.03 | 1.166,8.165 | 26.615 | 0.001 |
Moment A-P | - | - | - | 1,7 | 6.525 | 0.04 | 1.098,7.685 | 7.455 | 0.030 |
Work | 1,7 | 94.366 | ≤0.001 | - | - | - | 2,14 | 9.674 | 0.002 |
Power | 1,7 | 136.920 | ≤0.001 | - | - | - | 2,14 | 14.208 | ≤0.001 |
TCF | 1,7 | 104.141 | ≤0.001 | - | - | - | 2,14 | 23.637 | ≤0.001 |
TCF-L | 1,7 | 724.557 | ≤0.001 | - | - | - | 2,14 | 20.211 | ≤0.001 |
TSF | 1,7 | 82.266 | ≤0.001 | - | - | - | 2,14 | 62.098 | ≤0.001 |
TSF-L | 1,7 | 753.571 | ≤0.001 | - | - | - | 2,14 | 76.870 | ≤0.001 |
% TCF Overload | 1,7 | 104.141 | ≤0.001 | - | - | - | 2,14 | 23.637 | ≤0.001 |
%TSF Overload | 1,7 | 72.914 | ≤0.001 | - | - | - | 2,14 | 52.315 | ≤0.001 |
p-values given by Bonferroni post hoc test, SPSS for Windows, version 21.0
Level of significance for lifting at different height.
Parameters | p-value |
---|
F-K vs F-S | F-K vs K-S | K-S vs F-S |
---|
VGRF | -- | ≤0.001 | 0.002 |
Moment A-P | -- | -- | 0.004 |
Work | -- | -- | 0.02 |
Power | 0.020 | -- | 0.009 |
TCF | -- | 0.004 | 0.003 |
TCF-L | ≤0.001 | -- | 0.002 |
TSF | -- | ≤0.001 | ≤0.001 |
TSF-L | -- | ≤0.001 | ≤0.001 |
% TCF Overload | -- | 0.004 | 0.003 |
%TSF Overload | -- | ≤0.001 | ≤0.001 |
p-values given by Bonferroni post hoc test, SPSS for Windows, version 21.0
Real-time Kinetics
Vertical Ground Reaction Force (VGRF), Anterior-Posterior Moment (M A-P), Work done and Power generated due to lifting weights, frequencies of lift and lifting heights are reported graphically in [Table/Fig-3]. Values of realtime kinetic variables reported were significantly lower during lifting at K-S followed by F-K and F-S height. Statistical significance are reported in [Table/Fig-6,7].
Prediction Kinetics
The [Table/Fig-4] represents Total Compressive Force (TCF), Total Shearing Force (TSF), total Compressive Force due to Load (CF-L) and total Shearing Force due to Load (SF-L) exerted on L5/S1 segment of spine. The trend in values for parameters TCF, TSF, CF-L and SF-L are in the order K-S<F-K<F-S. [Table/Fig-6,7] represent significant statistics observed.
The percentage of the excess TCF and TSF values above the maximum permitted spine loading [28,29] are presented in [Table/Fig-5]. Statistically significant values are given in [Table/Fig-6,7].
Discussion
Health hazards due to MWL are established facts pertaining to almost every sector of industries [1-5]. Past studies have established Low Back Pain (LBP) as the most important occupational health risk caused by ‘lifting weight’ [6-10]. Industrial workers are most susceptible to LBP caused due to disc disruption or degeneration and this factor accounts for about 39% of chronic back injuries [31]. In a psycho-physiological study by Jorgensen MJ et al., which aimed to formulate effective method for identifying MAWL to reduce lower back disorder, 15 male college students were recruited as volunteers and their heart rate, trunk positions, velocities and accelerations along with estimated spinal loading in terms of moments and spinal forces in three dimensions with the help of EMG-assisted biomechanical model were measured. Heart rate predicted moment, compression and shear force were found to increase with an increase in lifting weight [12].
Another study consisting of nine each of male and female college students determined MAWLs for single tasks (pull, lift, carry, lower and push) along with their combinations at different frequencies (three per minute, six per minute) and heights (floor, knuckle and shoulder). Comparison of the MAWL for each of the combination tasks with the single tasks suggested that the use of single tasks to estimate the MAWLs for combination tasks were unacceptable [13]. Past studies have established the mechanism by which disc disruption and degeneration occurs and eventually results in back pain [32]. The most affected region of the spine is the vertebral endplate where the spinal load is sufficiently higher. These endplates are attached to the spinal discs and are important in disc nutrition from blood vessels of the vertebral bones. When a fracture occurs in discs, the body’s healing mechanism seal the crack with scar tissue, inhibiting the flow of nutrition from blood to discs. This inadequate nutrition supply will gradually decrease the discs, leading to fissures or tears of disc fibres with inflammatory response and ultimately result in the sensation of LBP [33]. There are several views as regards to how the disc fracture occurs. One of the most possible causes of disc fracture is the fatigue failure or the overuse injury, wherein a small fracture appears in the endplate due to MWL. With repeated MLW tasks it transforms into the full-fledged fracture. Thus, a sub-maximal repetitive loading can lead to an injury experienced that is similar to an injury due to onetime overload of the tissue beyond its strength [34,35]. The best possible method to minimize degeneration of endplates might be reducing the probability of occurrence of initial endplate fracture. Therefore adequate Manual Material Handling (MMH) task design approach is needed to reduce low back pain resulting from MMH. The study by Hoozemans MJ et al., established that lifting height and weight were important determinants of the low back load during MMH [36]. Also, a review article by Paul PFMK et al., discussed that lifting objects of less than 3 kg were manually handled for more than two times a minute but loads >25 kg regardless of the frequency were considered to be a risk factor for LBP [37]. Therefore, for the present study medium (10 kg) and heavy (20 kg) loads were selected to identify the MAWL for Indian population. A study by Garg A et al., compared biomechanical stresses between static and dynamic biomechanical models during performing lifting tasks. Results indicated that reflected compressive force at the lower back and peak moments at various body joints in dynamic biomechanical simulation were approximately two/three times greater than those based on a static biomechanical simulation [17]. The study by Hoozemans MJ et al., investigated the effect of lifting height and mass on peak low back load in terms of net moments, compression forces and anterior-posterior shear forces [36]. They reported beneficial effects of optimising vertical location of the load as against decreasing the mass of lifting. Results of present study corroborate with those of Hoozemans MJ et al. Present study shows that anterior-posterior moment was significantly higher during F-S height lift, which involved complete upper torso bending as compared to K-S height lift involving upright posture [36]. Similarly, TSF and SF-L were also significantly higher during lifting from low level (floor height) to higher (knuckle or shoulder) height as against lifting from K-S height. In the present subjects, the force exerted on L5/S1 of the spine was significantly higher during 20 kg than 10 kg weightlifting, irrespective of the frequency of lift.
The National Institute of Occupational Safety and Health (NIOSH) guidelines for manual lifting recommends that no worker should lift a load that gives rise to disc compression above 6361 N and the administrative control limit be considered for giving rise to disc compressions on L5/S1 segment of spine greater than 3425 N. It is established that beyond this limit spinal injury initiates [25]. Observations of the present study indicate that lifting of 10 kg weight to K-S lifting height for both frequencies of lift was safe in terms of NIOSH lifting criterion as the spinal force generated was less than the recommended limit. However, for lifting heights F-K or F-S, where bending is required, TCF exerted on the L5/S1 segment of the spine showed overloading of >10% for 10kg weightlifting and >50% for 20 kg weightlifting, respectively, irrespective of lifting frequency. Results of present study further show that, considering NIOSH recommended a limit of TSF on the spine for MWL that involved bending [30], for 10 kg weight of lift overloading of spine observed was ≥100% or more, while with 20 kg, it showed overloading of ≥150%. Lifting 10 kg weight without bending (K-S) resulted in >35% overload. It has been reported that not only lifting the load, but vertical distance of the load lifting also forms an important determinant of safe lifting [38]. Molen VDHF et al., indicated that implementation of mechanical lifting equipment or technique to adjust manual lifting height would be beneficial to reduce lower back injury when lifting block weight of 11 to 16 kg range [39]. Corroborating these studies, present study results also showed that real-time force data and prediction force data agree with each other and was higher during F-S height lifting than either F-K or K-S height lifting. As regards long distance (F-S) lifting, spinal overload was beyond NIOSH acceptable limit [25], indicating that such lifting tasks were not advisable for the present population. In the study by Freivalds A et al., VGRF and predicted L5/S1 compressive force was found to increase with increasing lifting weight during lifting two different psychophysiological loads as determined by the participants’ subjective feeling of exertion or fatigue with different size boxes with and without handle [26]. Findings of the present study also indicated similar effects of significantly higher VGRF during 20 kg as compared to 10 kg lifting the weight, in the order of F-S >F-K >K-S heights. The present investigation also reported that TCF and CF-L exerted on L5/S1 segment of the spine increased significantly with lifting height, from the lower level (floor) to higher lifting heights (knuckle or shoulder). Further, the present findings indicated that an increase in lifting weight had significant effects on spinal load exertion. Based on the above observations it can be suggested that manual lifting of a load greater than 10 kg should not be carried out by average Indian population even in the occupationally active age group. This may reduce spinal loading and in turn, bring about a reduction in the incidents of LBP. Apart from the reduction in the load to be lifted, avoiding bending during lifting is essential. The novelty of this study lies in simultaneous collection and presentation of real-time and predicted kinetics data for MWL tasks which validate each other by showing a similar trend in responses. Although, the results of the study have added the wealth of new information on MWL tasks by young Indian population.
Limitation
The present study has a limitation as well, in terms of fewer subjects considered. Therefore, based on the results of this pilot study, we cannot recommend or formulate any lifting norms for the present population as more subjects need to be studied on similar lines.
Conclusion
The present study clearly indicates that according to both real-time and prediction kinetics data, lifting of loads up to 10 kg from knuckle to shoulder height at 1 lift per minute is within an acceptable limit. A maximum lifting load of 10 kg may be suggested for lifting tasks which do not involve any bending of the upper torso, irrespective of lifting height. Lifting 10 kg load while bending caused excessive spinal loading which according to NIOSH recommendations increased the risk of LBP. An important and novel outcome of this study is the fact that real-time kinetics responses corroborated with 2D predicted kinetics responses. This indicated that under adverse field situations where a collection of real-time motion data is not possible, one could draw valid inferences using the 2D images of the workers while carrying out the particular task.
Supplementary data are available with this file.
p-values given by Bonferroni post hoc test, SPSS for Windows, version 21.0p-values given by Bonferroni post hoc test, SPSS for Windows, version 21.0