Globally, Acute Gastroenteritis (AGE) is a major public health problem and causes morbidity and mortality among young children. Group A Rotavirus (RVA) is an important etiological agent of severe gastroenteritis in children [1]. In India, recent estimates have shown 872,000 hospitalisations and 78,500 annual deaths occur in children below five years due to rotavirus infection [2]. Rotaviruses belong to the Reoviridae family. The 11-segmented genome encodes six structural (VP1-VP4, VP6, VP7) and five/six non-structural proteins (NSP1-NSP5/6) [3,4]. The intermediate capsid protein VP6 is the primary target for diagnostic assays. It is highly immunogenic and classifies RVA into subgroups SG I, SGII, SGI+II, and SG non-I, non-II based on their reactivity to monoclonal antibodies (MAb) [5]. A nucleotide based classification by the Rotavirus Classification Working Group (RCWG) has identified 16 genotypes (I1-I16) for VP6 gene (SG 1 and II correspond to genotype I2 and I1, respectively [6,7].
The VP7 and VP4 outer proteins, serve as viral attachment and neutralising antigens define G and P genotypes, respectively [8]. Currently, 27 G, 37 P and 42 different G-P type combinations have been reported in humans and animals [3]. G1, G2, G3, G4 and G9 and P4, P6 and P8 are the common circulating strains [9]. Globally, G1P8, G2P4, G3P8, G4P8 and G9P8 are the most prevalent genotype combinations of rotavirus strains cause human infections [10].
The segmented nature of the RVA genome causes re-assortments among co-circulating strains of human and animal origin resulting in the emergence of novel strains [3,11]. Co-infection with different human strains may also give to reassortants. Point mutation, recombination and rearrangement are mechanisms driving high genetic diversity of rotavirus.
VP7 and VP4 proteins are the prime targets for vaccines which elicit neutralising antibody responses that generate serotype-specific and cross-reactive protection [12]. Currently, three vaccines are available; ‘Rotarix’ a monovalent vaccine derived from attenuated human RVA G1P8 strain (GlaxoSmithKline Biologicals, Rixensart, Belgium), ‘RotaTeq’ a pentavalent human bovine reassortment vaccine contain G1, G2, G3, G4 and P8 strains (Merck Vaccines, Whitehouse Station, NJ, USA) and the ‘indigenous Indian Rotavac’, an oral live attenuated vaccine derived from G9P11 human bovine reassortment strain 116E (Bharat Biotech International, India) [3,4,9]. Studies indicate that vaccine efficacy is potentially higher in developed countries than developing countries [13-15]. Immune pressure in face of vaccination may drive selection of new genotypes or mutants may evade the vaccine induced immune response due to substitution in the antigenic region of VP4 and VP7 gene [16].
In India, rotavirus vaccine was included in the Expanded Programme of Immunisation (EPI) in 2016. In this post vaccination era, it is important to conduct surveillance of circulating RVA strains, to identify reasssortants and emergence of latest genotypes. The present study aimed to characterise circulating RVA genotypes in the Central Travancore region in Kerala, India, based on the VP4, VP6 and VP7 genes. In addition, we compared VP4 and VP7 antigenic epitopes of G1P8 strains with vaccine strains to determine the genetic variability.
Materials and Methods
Study Population
During January - December 2013, stool samples (n=75) were collected from children (<10 years, with symptoms of AGE), admitted to the Department of Paediatrics, Pushpagiri Medical College Hospital, Tiruvalla, Kerala. The study was approved by the Institutional review board (Ref: PIMS & RC/EI/388A/10), of 12 march 2011. Children with age (≥3 loose stool per day) with or without associated symptoms such as one or more episodes of vomiting, dehydration, fever and abdominal pain were enrolled in this study. All patient details including, onset of disease, episodes of diarrhoea and vomiting, severity of diarrhoeal disease were extracted from medical records of study subjects. All samples were tested by rotavirus VP6 antigen detection ELISA (PremierTM Rotaclone, Meridian Bioscience, OH, USA) as per the kit instruction. Positives were confirmed by RT- PCR and sequenced for molecular characterisation.
RNA extraction and Reverse-transcription polymerase chain reaction (RT-PCR) of VP6, VP7 and VP4 genes: A 10% faecal suspension was prepared in 0.01M phosphate buffered saline (PBS, pH 7.2) and centrifuged at 10,000 rpm for 30 minutes. Supernatant were aliquoted and stored at-70°C for molecular analysis. Viral RNA was extracted using QIAamp Viral RNA mini kit (QIAGEN, Hilden, Germany) according to the manufacturer’s instructions [17].
All antigen positives were tested by RT-PCR using published primers targeting VP6 gene (379 bp) [17]. The PCR conditions involved initial activation at 95°C for 15 minutes, followed by 40 cycles of amplification (94°C for 30 seconds, 53°C for 1 minute, and 72°C for 1 minute) with a final extension of 72°C for 10 minute. For G and P typing, amplification of VP7 complete gene (1062 bp) and VP4 partial gene (729 bp) were carried out with specific primers [18]. The amplification conditions were as follows: an initial denaturation at 95°C for 15 minute, followed by 40 cycles at 94°C for 1 minute, 50°C for 1 minute, and 70°C for 2 minute with a final extension step at 72°C for 10 minute.
Nucleotide Sequencing and Phylogenetic Analysis
Amplified products were sequenced (Scigenom Pvt., ltd., Kochi) using PCR primers and analysed by Basic Local Alignment Search Tool (BLAST). Multiple nucleotide and amino acid sequence alignment were done with the corresponding RVA reference sequence available in the GenBank by using Clustal Wand BioEdit software. Phylogenetic tree was constructed by Maximum likelihood method using the MEGA 6.0 software (Molecular Evolutionary Genetics Analysis), with 1000 bootstrap replicates.
Accession Numbers
The nucleotide sequences of VP7 gene derived in the study were submitted to GenBank under the accession numbers KU945818- KU945822, KX009876-KX009891. The VP4 sequences were submitted under the accession numbers KX011939-KX011961 and the VP6 gene sequences were deposited under accession numbers KX083646-KX083666, KX181645 and KX181646.
Statistical Analysis
Statistical analysis was performed using SPSS version 16.0. Differences in categorical variables were tested using the student t-test and chi-square (χ2) test. A p-value of <0.05 was considered statistically significant.
Results
Baseline Characteristics of Study Population
Out of 75 {female 29.34% (n=22), male 70.67% (n=53)}, 30.6% (n=23) of the study subjects with AGE were positive for RVA VP6 antigen by ELISA. Of the positives, 61% (n=14) were male and 39% (n=9) were female (p>0.05). The mean age (±SD) of study subjects was 2.39±2.09. Higher rotavirus positivity (65%) was observed in 0-2 years. Rotavirus AGE was characterised by watery diarrhoea, sudden onset of vomiting, high grade fever and some or severe dehydration. The mean duration of RVA diarrhoea was (3.7±1.2) days, which was statistically significant (p=0.003), compared to RVA negative cases (2.8±1.2). Incidence of vomiting was also higher in positive cases (95.7%, p=0.001); however, the duration and episodes of vomiting were not statistically significant. Dehydration was seen in 78.3% (p=0.001) of RVA positives. Severity of diarrhoeal disease was categorized as mild (0-5), moderate (6-10), severe (11-15) and very severe (16-20) using Vesikari scoring system [19]. 83% scored as severe, 13% moderate and 4.3% mild with a mean of 12.5±2.9.
Genotyping and Phylogenetic analysis based on VP6, VP7 and VP4 genes
A total of 23 RVA strains were sequenced and analysed for VP4, VP7 and VP6 genes, G1 (n=17, 80.95%) was the predominant G type detected, followed by G9 (n=4, 19.04%) and few non-typeable strains (n=2, 8.6%). P types were predominantly P8 (n=21, 91.3%) followed by P6 (n=1, 4.3%) and P4 (n=1, 4.3%). G1P8 (n=16, 69.56%) was the most common strain circulating in the region. G9P8 (13.04%), G1P6 (4.3%), G9P4 (4.3%) strains were also detected.
Analysis of VP6 gene
VP6 nucleotide sequence analysis of the RVA strains was done and compared with reference strains. Phylogenetic analysis classified strains into two distinct genotypes. Majority of the strains {91.30% (n=21)} belonged to genotype I1, two strains were identified as genotype I2 [Table/Fig-1]. Genotypes I1 study strains showed 95%-98% identity with reference strains RMC100, US89701, and strains from Pune, Manipur and Bangladesh. Two study strains were clustered with reference strains from Pune, Delhi, US1205 with high bootstrap support, determined as Genotypes I2.
Phylogenetic dendogram based on the VP6 gene of RVA strains circulating in Kerala with reference strains. The tree was constructed using MEGA.6 program with 1000 bootstrap replicate. Strains from Kerala were indicated by symbol (◆).
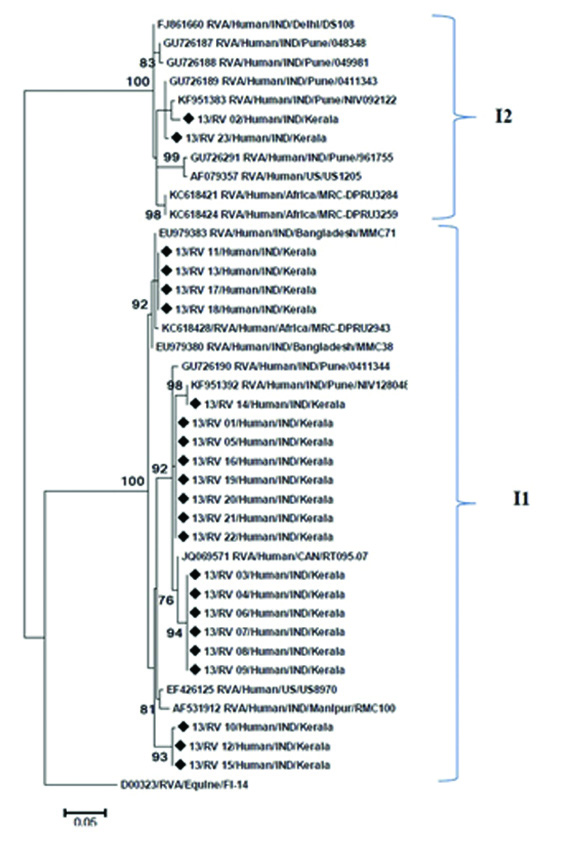
Analysis of VP7 gene
VP7 nucleotide sequences were compared with reference and vaccine strains from GenBank. Phylogenetic analysis showed that strains were distributed into two distinct clusters G1 and G9. Majority of the G1(n=86.95%) strains exhibit maximum nucleotide and amino acid identity (99%) with Indian reference strains (Kolkata, Vellore, Pune and Manipur) and strains from Thailand, Bangladesh and Australia [Table/Fig-2]. Within the G1 cluster, few G1 (n=3) strains separately clustered with Rotarix(A41CB052A), RotaTeq(W179-9) vaccine strains and other reference strains from US and Japan with a high bootstrap support [Table/Fig-2]. These three strains showed 97% nucleotide identity with Rotarix and 93% identity with RotaTeq vaccine strains. G9 strains in this study were closely associated with reference strains from India, Bhutan, Bangladesh and Thailand, showed 96-98% nucleotide and amino acid identity. Their nucleotide homology with G9 116 E vaccine strain was 88% [Table/Fig-2].
Phylogenetic dendogram based on nucleotide sequence of VP7 genes of RVA strains circulating in Kerala with reference and vaccine strains. The tree was constructed based on Maximum likelihood method using MEGA 6.program. Strains from Kerala were indicated by symbol (◆).
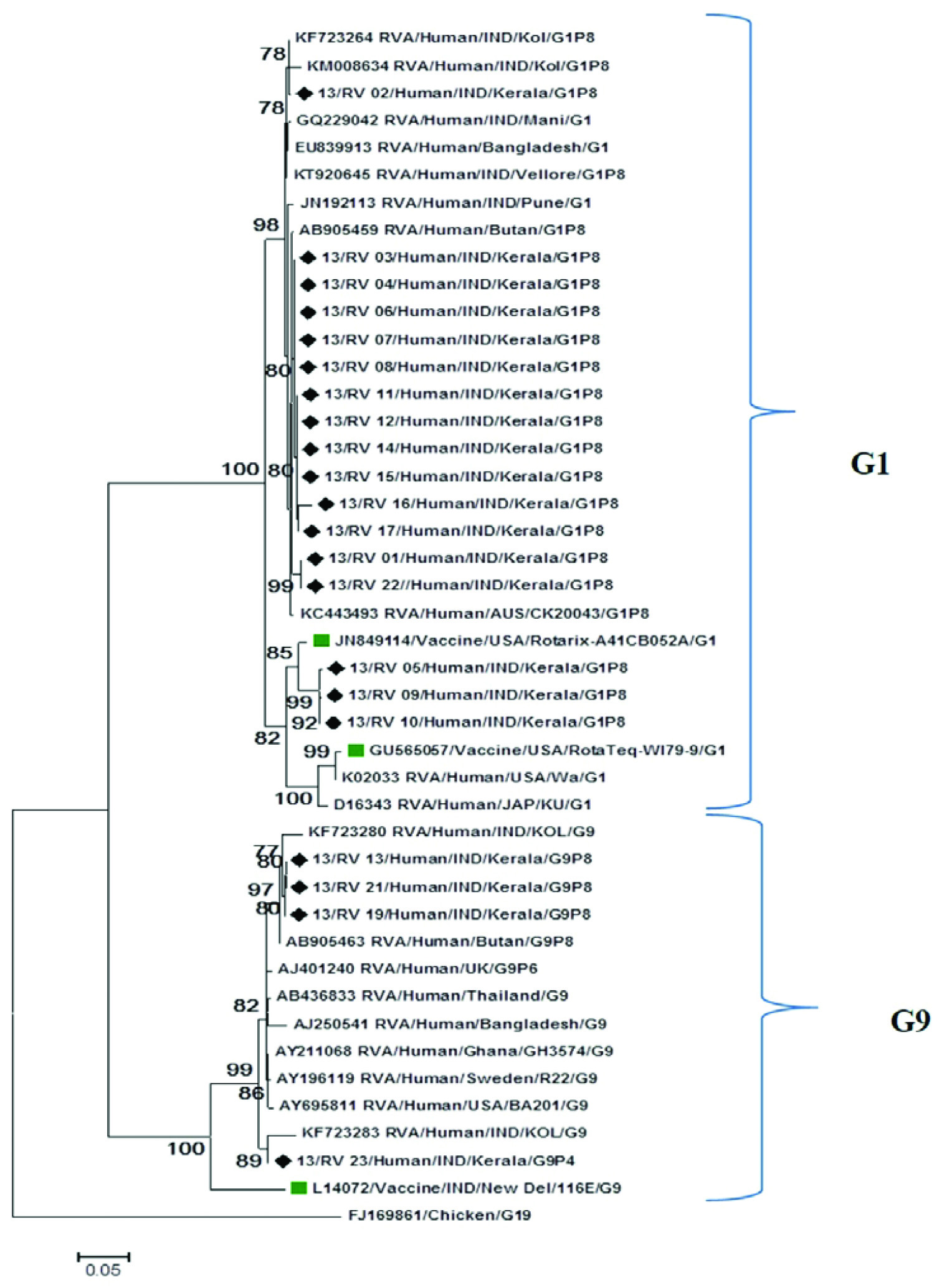
Analysis of VP4 gene
Phylogenetic analysis of VP4 gene revealed that the study strains belonged to genotypes P8, P4 and P6. All P8 (n=21) strains were clustered with reference strains from different countries, but closely associated with those from India (Kolkata, Pune, Manipur) and Thailand showing 98-99% nucleotide and amino acid similarity [Table/Fig-3]. P8 strains in this study shared 91-94% similarity in nucleotide and amino acid level when compared with Rotarix (A41CB052A) and RotaTeq (WI79-4) vaccine strains. P6 (n=1) and P4 (n=1) strains clustered separately in two subsets with Indian and Bhutan reference strains with high bootstrap support [Table/Fig-3].
Phylogenetic dendogram based on nucleotide sequence of VP4 genes of RVA strains circulating in Kerala with reference and vaccine strains. The tree was constructed based on Maximum likelihood method using MEGA 6. Program. Strains from Kerala were indicated by symbol (◆).
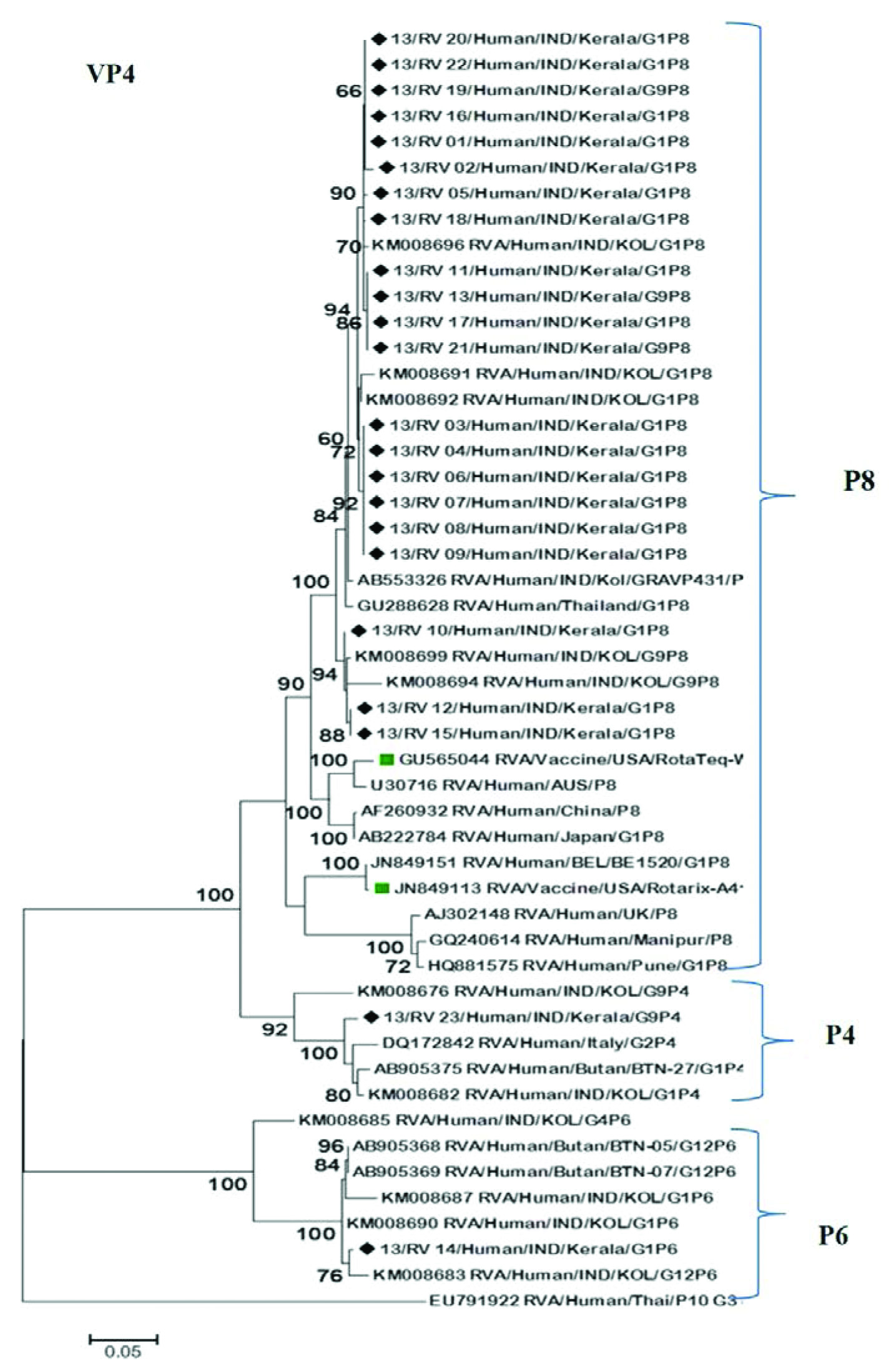
Comparative analysis of the deduced aminoacid sequence of VP6 fragment
Analysis of deduced amino acid sequences of VP6 fragment showed conserved amino acid substitution distinguishing strains into two genotypes I1 and I2. Genotype I1 showed substitution in three strains at position 281(V281 I) and 355 (I 355V) [Table/Fig-4]. Strain specific variations were observed in genotype I2 at position 305(Asn-Ala), 310(Gln-Asn), 315 (Glu-Gln/Leu), 339(Asn-Ser), 342(Leu-Met), 348(Ala-Ser) on comparison with genotype I1 [Table/Fig-4].
Showing alignment of the specific amino acid residues of the VP6 protein of RVA strains grouped into genotype I1 and I2 with reference strains.
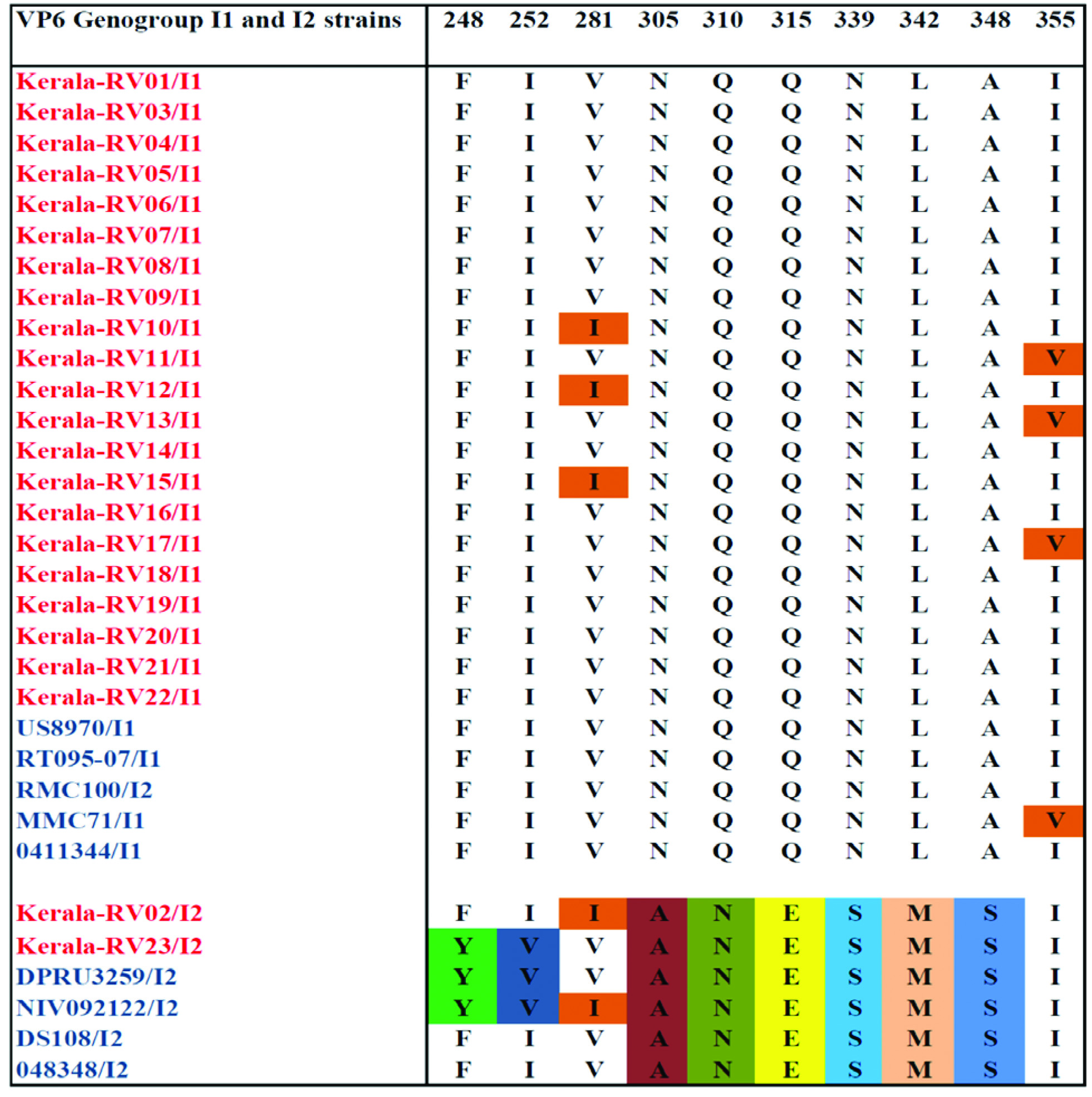
Comparative analysis of deduced amino acid sequence of VP7 and VP4 antigenic epitopes of G1P8 RVA strains with vaccine strains
Amino acid sequence of circulating G1P8 rotavirus study strains were compared with Rotarix and RotaTeq vaccine strains. The VP7 protein consists of two antigenic epitopes: 7-1(7-1a and 7-1b) and 7-2, contain 29 amino acid residues. In the epitopes 7-1a majority of the strains showed substitutions at N94S, E97D, S123N, and K291R positions while, 7-1b was more conserved, showed D213Y and D213E substitutions in two strains. In 7-2 epitope, we have observed S147N and M217T substitution in study strains when compared with Rotarix G1 vaccine strain and substitution in M217T residue with RotaTeq vaccine strain [Table/Fig-5].
Showing comparison of residues that constitute the 7-1a, 7-1b and 7-2 epitopes of study strains and the vaccine strains.
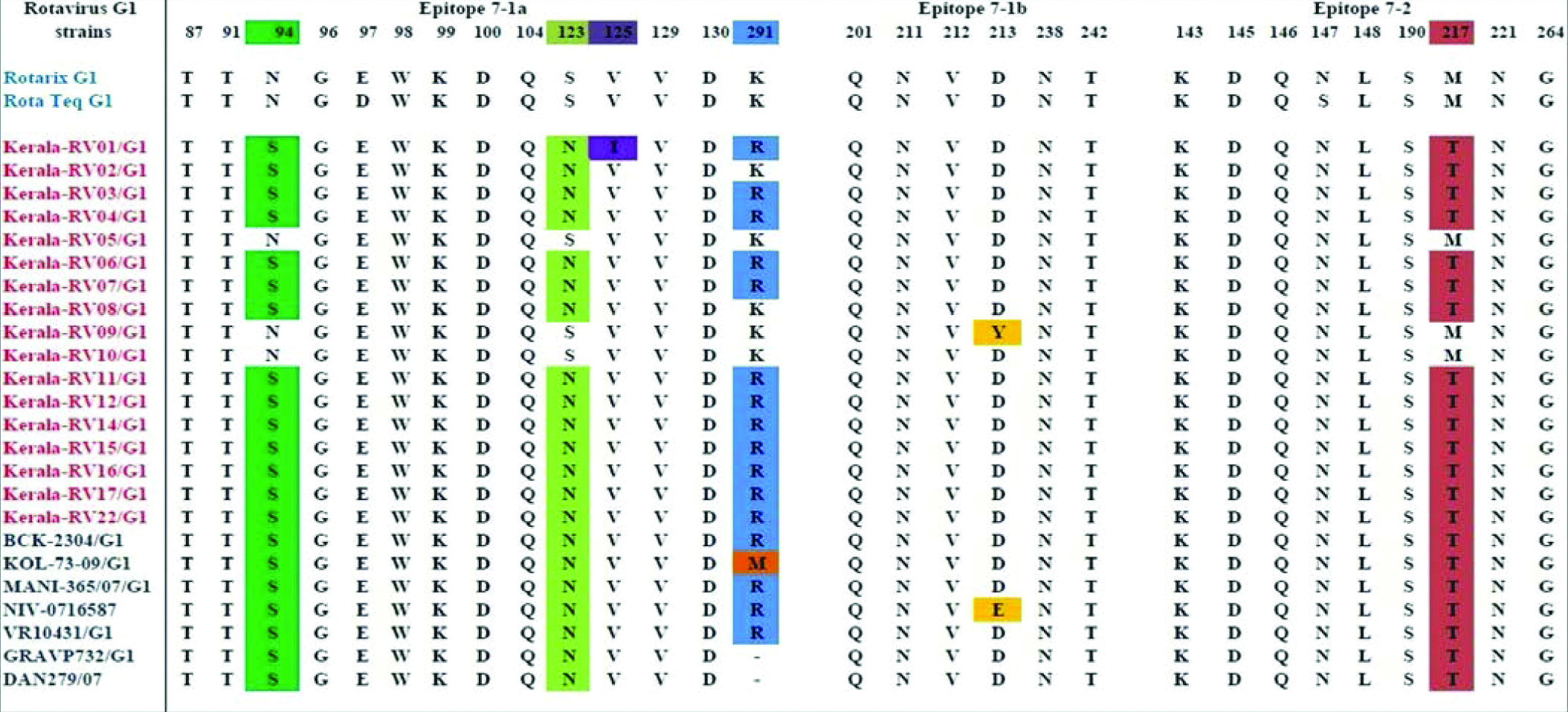
Comparison of deduced amino acid sequence of VP4 (VP8) protein of G1P8 study strains with vaccine strains showed mismatches at 24 amino acid residues [Table/Fig-6]. In the VP8 epitope region, we have identified N113D, S125N, S131R, N135D substitutions in the 8-3 region and N195G in the 8-1 region. Two substitutions N113D, S145G were present in all strains compared to RotaTeq vaccine strains. N113D, S125N, S131R, N135D, S145G, S189N, N195G are the substitutions observed in all strains with Rotarix vaccine. Of that 113,145 and 195 are the known neutralisation escape mutation sites [Table/Fig-6].
Showing antigenic variation in the VP4 (VP8) amino acid residues of study strains in comparison with vaccine and reference strains. Neutralisation escape mutation sites were high lightened.
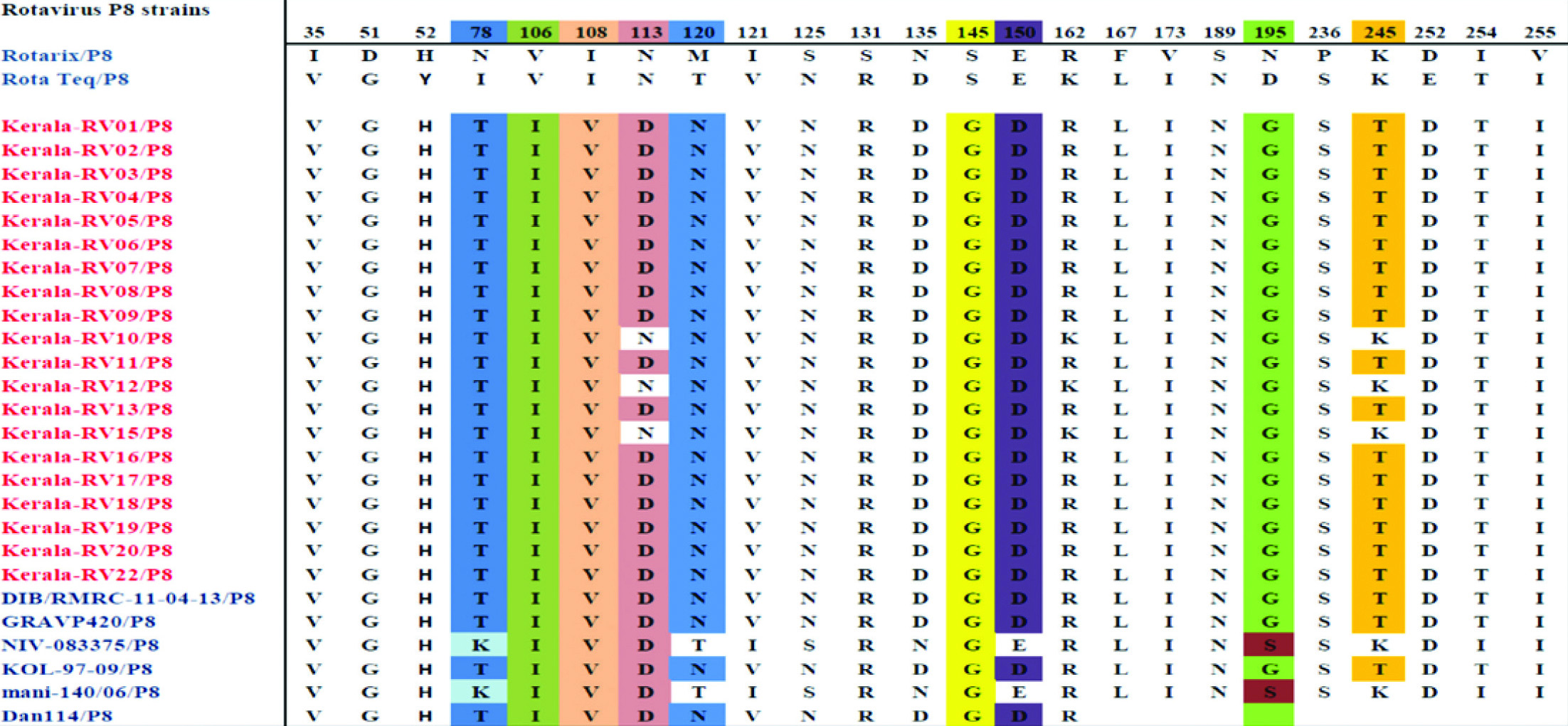
Discussion
Rotavirus infection remains a significant cause of mortality among young children in India. The two commercially available vaccines Rotarix and RotaTeq have proven their efficacious in developed countries. However, studies have reported their effectiveness and impact is lower in developing countries (Asia and Africa) [8]. The genetic diversity of RVA strains, their genetic reassortment and recombination and possible interspecies transmission has rendered, control of infection remains largely unsuccessful [9]. During the study period rotavirus vaccines were not included in the national immunisation scheme. The higher vaccine cost restricted its usage in patients.
Only a small proportion of children (10%) were vaccinated at the centre during the study period. They were not included in the study because present study subjects were all inpatients with symptoms of acute gastroenteritis.
The present study was done to understand genomic diversity of circulating RVA types in Central Kerala after the introduction of vaccine in present hospital in 2012. None of the subjects had a prior RVA vaccination history. In this study prevalence of rotavirus infection was more in children below two years with a peak incidence (34.78%) observed in younger 6-12-month-old children. This is in sync with previous reports from India [3,20]. Risk factors that increase susceptibility to rotavirus infection are age (<2 years), low birth weight, birth season, transmission from siblings, poverty, malnutrition, dehydration, poor food hygiene, lack of sanitation, water contamination and education level [21]. Indian studies have reported high mortality rates among girls due to poor nutritional status, lack of immunization and failure to seek medical attention and health care; however in this study there is no difference observed in the prevalence among sexes [20].
Incidence of vomiting was higher in RVA positive cases and diarrhoea lasted for a median of five days with multiple episodes. We found that 69.6% RVA positive cases had some dehydration and 8.7% had severe dehydration. Duration of the hospital stay was longer and all of them required oral rehydration. According to Vesikari scoring system 83% RVA positive cases had a severe clinical course than gastroenteritis from other causes [19].
In the present study, G1 (80.95%) and G9 (19.04%) were the circulating genotypes. Previous Indian studies have reported dominance of G1 strains at frequency of 31-60% [9,18,22]. In India, during 2013-2014 G1P8 was the most prevalent genotype detected in western India, were as in northern India G1P8 was detected in 2005-2007 [8,23]. Recent study from southern India has reported G2P4 was the predominant genotype identified until 2007, then it was replaced by G1P8 from 2008 onwards. In 2016, G9P4 was the most frequently detected genotype (42%) in south India, were as in eastern India G3P8 (41.30%) was the most prevalent strain followed by G1P8 (17.39 %) [4,8,24,25]. G9 is an emerging global genotype, accounting for 70% of rotavirus infection world-wide [26]. It is also reported an increase prevalence (33.3%) of novel G12 rotavirus strains in India during 2006 to 2007 [27].
In our study G1P8 (69.56%) was the most prevalent G/P combination identified and is reported in 50-65% of rotavirus infections children worldwide [27]. A previous study from Kerala reported circulation of G1P8 (49.7%) and G9P8 (26.4%) [22]. Few additional genotypes G9P8 (13.04%), G1P6 and G9P4 have been infrequently identified. It was noted that strains from Kerala exhibited divergence when compared with the original vaccine strains suggesting changes in the circulating strains overtime. Similar finding were reported from India and other countries in the present study [3,4,15,16,28,29]. None of the study subjects were vaccinated against rotavirus. We cannot say vaccine pressure was responsible for a strain shift in this area. Since, there is paucity of data from the region it is difficult to speculate on the origin of circulating genotypes.
This is the first study from Kerala which provide phylogenetic and molecular analysis of VP6 gene. Presence of genotypes I1 (91.30%) and I2 (8.7%) were detected. Indian studies have reported dominance of genogroup I (73.5%) compared to genogroup II (26.5%) [17]. Genotype I1 was the most frequent VP6 genotype detected in this study and showed close similarity with standard reference strains RMC100, US8970 and previously reported strains from India, Bangladesh and Africa. Majority of the G1P8 strains were genotype I1, two strains G1P8 and G9 P4 were identified as genotype I2 clustered with US1205, Indian and African reference strains.
The present results suggest the possibility of emergence of strains which could evade the immune response elicited by the RVA vaccine. In the study we have noted that strains from Kerala exhibited divergence when compared with the original vaccine strains suggesting changes in the circulating strains. G9 and G1 strains in this study showed less homology with vaccine strains, suggesting genetic diversity to escape from vaccine-derived immune response. The antigenic variation observed between the circulating strains and components of vaccine strains may potentially reduce the immune protection of vaccinated children and it may influence the vaccine efficacy.
The phylogenetic analysis of VP7 and VP4 genes indicates greater homology of study strains with reference strains from Kolkata, Pune, Vellore, Delhi, Manipur, Bangladesh, Thailand, and Bhutan. We also found that G1P8 study strains showed intra genotypic difference when comparison with G1 and P8 components of Rotarix and RotaTeq vaccine strains.
Deduced aminoacid sequences alignment of VP6 gene (aa 246-363) distinguishes strains into 2 genotypes I1 and I2. Majority of the strain belong to Genotype I1, out of which three of them showed substitutions at position 281(V281 I) and 355 (I 355V), Two strains belonged to genotype I2 showed conserved residues at position 305-Ala, 310-Asn, 315-Glu, 339-Asn, 342-Met and 348-Ser. Current results agreed with previous reports and suggest that the VP6 gene are distinct among genotypes [30].
The currently circulating G1 strains showed substitutions in their VP7 and VP4 antigenic epitopes when compared with vaccine strains. For the VP7 gene, eight substitutions (94,97,147,123,125,213,217,291) were observed in majority of strains, out of which two (97,147) were known neutralisation escape sites [31]. Previous studies have demonstrated that pre-existing antibodies can block the amino acid residues 94 and 213 of G 1 strains and provide protection against RVA infections. In the present study we found that epitopes 7-1a and 7-2 was more prone to variation than 7-1b. Three of the strains showed 100% sequence similarity with Rotarix vaccine strain.
The VP4 spike protein is cleaved into VP8 and VP5. Structural studies have shown VP8 forms the globular head contain 4 surface exposed neutralising epitopes (8-1 to 8-4), which include 25 amino acids (30). In the VP8 region of VP4 gene 22 substitutions were observed with Rotarix and 12 with RotaTeq vaccine strains. N113D, S125N, S131R, N135D, S145G, S189N, N195G are substitution observed in the VP8 antigenic epitope of G1P8 strains. Aminoacid substitution at position 113,145 and 195 are the reported neutralisation escape mutation sites [32]. Previous study have reported the non-conservative amino acid substitution residues N113D and D195G involves a change in charge from neutral to negative and negative to neutral respectively. It is also reported a change in polarity at the non-conservative position 120 where an M120N substitution observed [33]. Variation in the antigenic epitopes may possibly help these strains to escape from vaccine induced protection.
Limitation
The present study was limited to a single centre hospital based, similar study on a larger sample size will capture true burden of rotavirus infections circulating in the community. Further multi-centre studies are needed to understand the burden of rotavirus infection and vaccine efficacy in region.
Conclusion
Rotavirus vaccine has now been introduced into the universal immunisation programme. However, at the study site rotavirus immunisation has been ongoing since 2012. It is seen that though vaccination reduces disease severity, G9 and G1 strains in this study showed less homology with vaccine strains, suggesting genetic diversity to escape from vaccine-derived immune response. Small number of samples was a limitation of the study. This epidemiological data is important to detect the emergence of potentially epidemic strains, for the formulation of rotavirus vaccines. It is possible that the currently circulating G1 and G9 genotypes can be replaced by newly emerging strains and vaccine induced pressure on genotypes. Therefore, higher coverage of vaccination and continuous monitoring is important even after introduction of vaccine.