Introduction
Frontal EEG activity reflects emotion regulation [1] and exercise is thought to be an emotion-eliciting behaviour mediated by differences in the amplitudes of EEG signals between the left and right cerebral hemispheres. Specific changes in EEG asymmetry are correlated with post-exercise mood changes in humans. Frontal EEG asymmetry appears to best reflect changes in mood. Aerobic fitness appears to influence the positive relationship between frontal asymmetry and self-reports of improved affect and “energy” following exercise [2]. In separate studies, frontal asymmetry is also shown to predict self-reports of lower “energetic arousal’ versus “tense arousal” and more “vigour” immediately following exercise [3-5]. These findings indicate that post-exercise changes in frontal asymmetry might provide an EEG signature for affective responsiveness to exercise. In this regard, EEG asymmetry of the alpha bandwidth is reported to be greater in depressed than in healthy individuals and to be positively correlated with negative affect and behavioural inhibition [6]. More specifically, right frontal alpha EEG asymmetry is reported to be positively correlated with activation of avoidance mechanisms [7] and is shown to be negatively correlated with depressive symptoms [8]. Frontal alpha EEG asymmetry measured in conjunction with Blood Oxygen Level-Dependent (BOLD) activity during a real-time Functional Magnetic Resonance Imaging (fMRI) neuro feedback task shows that EEG asymmetry is correlated with BOLD signal asymmetry [9].
Most research has focused on frontal alpha asymmetry because of its inverse relationship with cortical activity; however, frontal delta, theta [10,11] and beta asymmetries may also need to be studied because of their possible contributions to cortical processing of emotion. Frontal interhemispheric coherence also reflects emotional states due to its role in frontal interconnectivity, most often measured by coherences [11].
Alpha EEG waves are shown to be negatively correlated with activation of the prefrontal and frontal cerebral cortices underlying the electrodes situated on the scalp [12-14]. The left frontal lobe is purported to be a hub for positive emotions and approach behaviours [15]. Adults exhibiting left-side asymmetries may also engage in more sensation-seeking behaviours and infants with left-sided asymmetries do more grasping and hugging than those with right-sided asymmetries [16]. The right frontal lobe, in contrast, is shown to be a hub for negative emotions and withdrawal behaviours [15]. Right-sided frontal asymmetry is thought to be a heritable trait that has been associated with depression [17]. Adults with right-sided asymmetries also seem to exhibit more avoidance behaviours and infants with right-sided asymmetries appear to do more hiding and crying than those with left-sided asymmetries [16]. Frontal asymmetry is also correlated with affective appraisal, with left (positive) asymmetry individuals appraising stimuli as more positive than controls, and vice versa for right (negative) asymmetry individuals [18].
Explanations for the current epidemic of overweight and obesity, in the U.S. and elsewhere, are multifactorial [19]. Poor diet and sedentary lifestyle, without much physical movement or exercise, are key determinants of overweight and obese phenotypes [20]. Regular exercise is shown to be associated with emotional resilience to acute stress in healthy adults [21]. Physical exercise is known to reduce anxiety, but the physiological mechanisms remain unclear. Aerobic exercise, such as swimming, is shown to increase neurogenesis in normal healthy animals, as well as in animals subjected to kainate-induced seizures [22,23]. Damage to the hippocampus is a key mechanism for both neurogenesis and the avoidance learning associated with the development of anxiety syndromes such as post-traumatic stress disorder [24]. These findings suggest the hypothesis that negative emotional responses to physical exercise may underlie a person’s inability to engage in physical exercise and that frontal EEG asymmetry may be predictive of changes in emotion/mood associated with exercise. The present study is, therefore, designed to test the hypothesis that exercise-induced changes in frontal asymmetry reflect changes in mood. Correlation between frontal asymmetry and mood after aerobic exercise could account for differential emotional responses to, and motivation for, engaging in exercise.
Materials and Methods
This study was designed as a prospective, within-subject investigation of the effects of aerobic exercise on mood and frontal EEG asymmetry. The study was approved by the Howard University Human Participant’s Institutional Review Board and was performed in the Nutrition and Exercise Science Laboratory at the Howard University Cancer Center in Washington, DC, during the period 1st June 2016 to 31st May 2017. All subjects provided informed consent.
Participants: Twenty young adult African-Americans (n =10 women, n = 10 men) volunteered as participants in pilot study to determine the feasibility of designing a larger study for the purpose of testing the hypothesis that a subpopulation of African-American women maintain a sedentary lifestyle because of an adverse emotional response to exercise. This pilot study was comprised of two groups of 10 men and 10 women; however, only 8 men and 8 women are included in the data analysis because 4 subjects failed to complete the study. The 4 dropouts resulted from a combination of exercise intolerance, failure to complete the VO2peak test and/or excessive numbers of qEEG artifacts. Sample size was justified by recommendations for pilot studies in clinical research [25]. Sample size estimation was based on preliminary findings during a one-week period of calibration and pretesting of all instruments and protocols. We predicted that an average rest vs. exercise difference of 20% would be detectable with a confidence interval of 90% for n=10 subjects.
Inclusion Criteria and Exclusion Criteria
Inclusion criteria were self-report of absence of diagnosed disease, sedentary lifestyle, and laboratory measurement of blood pressure within the normal range at rest. Exclusion criteria were use of tobacco products, alcohol or medications and physical exercise or caffeine intake within the past eight hours.
Procedures: Participants visited the laboratory three times, data collection was completed in 6 weeks, with an interval of 3 weeks between each data collection session. Laboratory visits two and three were randomly assigned, employing conditions for measuring mood response with and without submaximal exercise. Laboratory visits two and three were carried out during the luteal phase of the female subjects’ menstrual cycles, to limit a potentially confounding effect on mood [26]. Although studies which employ self-reported categorization of post-exercise emotional states, such as the Profile of Mood States (POMS) questionnaire [27] are semi-quantitative [28], we selected the POMS open source tool because it provides a convenient, relevant comparative database for determining the feasibility of performing future studies with inclusion of more rigorous quantification of emotional states.
Laboratory visit #1: For the first visit, participants performed a progressive exercise test to determine peak oxygen uptake (VO2peak) and physical anthropometric measures were made. The progressive exercise test was performed on an electromagnetically braked cycle ergometer (Corival, Lode, Groningen, Netherlands) and consisted of cycling at 20 W followed by a continuous progressive increase in power of 20 W.min-1 to the limit of volitional fatigue. Expired oxygen and carbon dioxide were analysed breath-by-breath using an online Max II metabolic system (Physio-Dyne Instrument Corp., Quogue, NY). Before each test, the metabolic system gas analysers and ventilatory gas volume transducer were calibrated with known gas concentrations and gas volumes, respectively. Peak oxygen uptake (VO2peak) was calculated as the highest 60-s mean value attained before test termination. Body composition of fat mass was measured using a whole body fan-beam dual energy X-ray absorptiometry densitometer (Hologic Discovery, Marlborough, MA).
Laboratory visit #2: The subject entered the laboratory and was instructed to perform a 5-minute warm-up on the cycle ergometer. Exercise at a specific percentage of peak oxygen consumption was used, in accordance with guidelines for exercise testing [29]; the highest submaximal exercise level maintainable by all the study subjects for 30 minutes was selected for this study, found to be 60% VO2peak during a one-week period of calibration and pretesting of all instruments and protocols. After completing the submaximal exercise workload, the subject was placed in a seated position, and a stretchable Lycra electrode cap with Ag/AgCl electrodes (Electro-Cap, Inc., Eaton, OH) was collected over four sites using the International 10-20 system (Fp1/Fp2, F7/F8). All leads were referenced to linked earlobes, and all electrode impedance were below 5 kΩ. EEG data were acquired using a Brainmaster Atlantis Mini Q-II acquisition system (Brainmaster Technologies, Inc., Bedford, OH). After instrumentation for EEG recording, each participant completed electronically administered measures assessing the intensity of feelings of tension, depression, vigour, fatigue, anger, and confusion (Profile of Mood States-65 items) [30]. Participants were instructed to respond to the Profile of Mood States-65-items “based on how you feel RIGHT NOW.” The Profile of Mood States-65-items questionnaires were completed immediately after the EEG measurements. EEG asymmetry scores were computed for the prefrontal and frontal electrode sites, subtracting the natural log of the alpha, delta, theta, and beta power of the electrodes overlying the left prefrontal or frontal (Fp1 or F7) from those overlying the right prefrontal or frontal (Fp2 or F8) cortices. Prefrontal and inferior frontal coherences were also computed. EEG coherences within each band (delta, theta, alpha and beta) were also computed. Coherences were defined as across-spectral power normalized to the total spectral power in that band, as a function of time. Values range from 0.0 to 1.0, similar to the commonly-used Pearson correlation coefficient. EEG coherence reflects the amount of similarity in frequency content between the Fp1 and Fp2 and between the F7 and F8 electrode sites. If the Fp1-Fp2 and the F7-F8 sites have exactly the same signal in a given band, the coherence is computed to be 1.0, and if they are entirely different, the coherence is computed to be 0.0 (Brainmaster Technologies, Inc., Bedford, OH).
Laboratory visit #3: The participants entered the laboratory and completed a 30-minute bout of seated, quiet rest, a widely used and well-established control condition in studies of acute exercise and mood [31]. The protocol was the same as that for the exercise session, except that the subject sat in a quiet resting position for 30 minutes.
Statistical Analysis
The significance of changes in post-control versus post-exercise prefrontal, inferior frontal asymmetries or coherences and mood scores was determined for the male and female subjects separately, using one-way analysis-of-variance with post-hoc one-tailed t-testing, at p≤ 0.05. Correlations between each mood parameter and prefrontal, inferior frontal asymmetries in each of the four EEG bandwidths (delta, theta, alpha, beta) were evaluated by computing Pearson’s product-moment coefficient, for the male and female subjects jointly and for the post-control and post-exercise conditions separately, with significance guaranteed at p≤ 0.05, 14 df. Data reported are means±standard errors.
Results
[Table/Fig-1] summarizes the anthropomorphic and physiological characteristics of the 16 study subjects who completed the study.
Characteristics of study subjects.
Variable | Men (n = 8) | Women (n = 8) |
---|
Age (yr) | 21.5±0.2 | 20.8±0.3 |
Height (cm) | 175.1±1.1 | 162.5±2.5 |
Weight (kg) | 77.9±2.3 | 69.7±7.0 |
Body fat mass (%) | 13.9±1.1 | 29.4±3.2 |
Heart rate (b.min-1) | 71.5±3.5 | 88.6±4.9 |
Systolic pressure (mm Hg) | 128.2±2.5 | 119.2±2.1 |
Diastolic pressure (mm Hg) | 89.6±1.4 | 79.5±2.7 |
VO2peak (ml.kg-1.min-1) | 30.3±2.2 | 27.0±1.7 |
[Table/Fig-2] shows the mood disturbance scores measured 30 minutes after control resting and after exercise. There were significant decrements in the post-exercise depression score for the females and in tension and total mood disturbance scores for both males and females. Gender differences were observed wherein the depression rating, the Fp1-Fp2 and F7-F8 alpha coherences [Table/Fig-3,4] were significantly decreased after exercise for the female subjects, but not for the male subjects.
Post-exercise and post-control mood ratings.
| Male (N = 8) | Female (N = 8) |
---|
Post-Exercise | Post-Control | Post-Exercise | Post-Control |
---|
Anger | 3.8±1.3 | 6.0±1.6 | 4.0±1.5 | 9.2±3.6 |
Confusion | 5.7±0.4 | 6.5±0.9 | 6.5±1.4 | 7.7±1.3 |
Depression | 4.2±1.5 | 6.0±0.8 | 3.1±1.2 | 6.2±1.2* |
Fatigue | 4.7±1.2 | 5.3±0.8 | 6.5±1.7 | 7.5±1.6 |
Tension | 7.0±1.5 | 10.1±1.3* | 6.8±2.0 | 10.7±1.7* |
Vigor | 16±1.5 | 14.6±1.9 | 14.0±1.7 | 9.6±2.0 |
TMD | 9.6±5.0 | 19.3±2.8* | 13.0±6.5 | 31.8±7.5* |
TMD = Total Mood Disturbance
Data in means±standard errors; *Differences significant at p≤ 0.05, F≥3.78
Note: Immediately after 30 minutes of exercise condition and control condition participants completed electronically administered Profile of Mood States Questionnaire
Post-exercise and post-control Fp1 and Fp2 asymmetries and coherences.
| Male (N = 8) | Female (N = 8) |
---|
Post-Exercise | Post-Control | Post-Exercise | Post-Control |
---|
Fp1, Fp2 (D) asym | .79±.04 | 1.0±.12* | .86±.07 | .79±.07 |
Fp1, Fp2 (A) asym | .77±.03 | .88±.06 (p=.07) | .75±.05 | .80±.02 |
Fp1, Fp2 (T) asym | .82±.04 | .96±.06 (p=.07) | .84±.06 | .88±.04 |
Fp1, Fp2 (B) asym | .78±.05 | .91±.13 | .79±.04 | .80±.02 |
Fp1, Fp2 (D) cohe | 56.4±4.2 | 53.9±2.5 | 54.4±.09 | 52.5±4.5 |
Fp1, Fp2 (A) cohe | 30.8±4.1 | 39.4±8.0 | 23.0±4.1 | 33.4±4.2* |
Fp1, Fp2 (T) cohe | 41.9±3.1 | 44.8±4.1 | 34.2±5.1 | 41.6±4.5 |
Fp1, Fp2 (B) cohe | 19.0±3.4 | 26.8±5.7 | 10.5±1.8 | 17.0±3.1 |
D = Delta; A = Alpha; T = Theta; B = Beta
Data in means±standard error; *Difference significant at p≤ 0.05, F≥ 3.78
Asym = Asymmetry
Cohe = Coherence
Post-exercise and post-control F7 and F8 asymmetries and coherences
| Male (N = 8) | Female (N = 8) |
---|
Post-Exercise | Post-Control | Post-Exercise | Post-Control |
---|
F7, F8 (D) asym | .88±.03 | .91±.05 | .92±.04 | .90±.04 |
F7, F8 (A) asym | .94±.02 | .92±.02 | .87±.03 | .91±.03 |
F7, F8 (T) asym | .93±.01 | .96±.03 | .98±.04 | .99±.02 |
F7, F8 (B) asym | .78±.05 | .91±.13 | .79±.04 | .80±.02 |
F7, F8 (D) cohe | 69.9±3.3 | 70.4±3.3 | 65.1±4.1 | 70.0±4.4 |
F7, F8 (A) cohe | 43.5±5.2 | 51.7±8.2 | 36.0±5.7 | 47.6±3.8* |
F7, F8 (T) cohe | 55.7±2.8 | 56.5±3.9 | 47.4±6.3 | 55.0±4.0 |
F7, F8 (B) cohe | 24.2±2.2 | 33.0±6.9 | 17.7±3.6 | 24.9±4.2 |
D = Delta; A = Alpha; T = Theta; B = Beta
Data in means±standard errors; *Difference significant at p≤ 0.07, F≥ 3.28
Asym = Asymmetry
Cohe = Coherence
[Table/Fig-3,4] present the EEG asymmetry and coherence measurements made immediately before completing the mood disturbance questionnaire. The post-exercise decrease in prefrontal delta asymmetry was significant (p≤0.05); the prefrontal alpha and theta asymmetries decrements were marginally significant in the male subjects (p=0.07); asymmetry changes in the females were not significant.
A significant decrement in post-exercise prefrontal alpha coherence (p≤0.05) and a marginally significant (p=0.07) decrement in inferior frontal alpha coherence were observed in the females. There were no significant changes in the post-exercise coherences in the males, for any of the bandwidths.
Significant correlations for the post-control and post-exercise conditions (n=16, 14 df).
Post-control conditions |
Fp1, Fp2 Beta asymmetry vs. Confusion = 0.48 |
Fp1, Fp2 Theta coherence vs. Anger = -0.47 |
Post-exercise conditions |
F7, F8 Beta asymmetry vs. Vigor = 0.46 |
F7, F8 Theta asymmetry vs. Vigor = -0.54 |
F7, F8 Delta asymmetry vs. Confusion = -0.45 |
F7, F8 Delta asymmetry vs. Tension = -0.49 |
F7, F8 Delta asymmetry vs. Vigor = -0.49 |
All correlations significant at p≤ 0.05.
[Table/Fig-5] summarizes the significant correlations found for the post-control and post-exercise mood disturbance scores and EEG measurements (n=16, 14 df). Significant negative correlation between the total mood disturbance score and prefrontal beta asymmetry was found, across post-rest and post-exercise (r= -0.38, p≤0.05, 30 df). There were also significant correlations between confusion and prefrontal beta asymmetry during rest (r= 0.48, p≤0.05, 14 df), between vigor and inferior frontal beta asymmetry, between vigor and both inferior frontal theta and delta asymmetry during exercise (r= 0.46, -0.54, p≤ 0.05, 14 df), and between vigor, confusion, tension and inferior frontal delta asymmetry post-exercise (r= -0.49, -0.45, -0.49, p≤0.05, 14 df).
[Table/Fig-6] demonstrates a significant correlation between the post-exercise tension score and F7-F8 delta asymmetry, by linear regression analysis. This correlation is of importance because significant differences between the post-control and post-exercise tension scores were found for both the male and the female groups of this cohort, shown in [Table/Fig-2] .
Linear regression analysis demonstrating correlation between post-exercise profile of mood states tension score and frontal F7-F8 delta asymmetry.
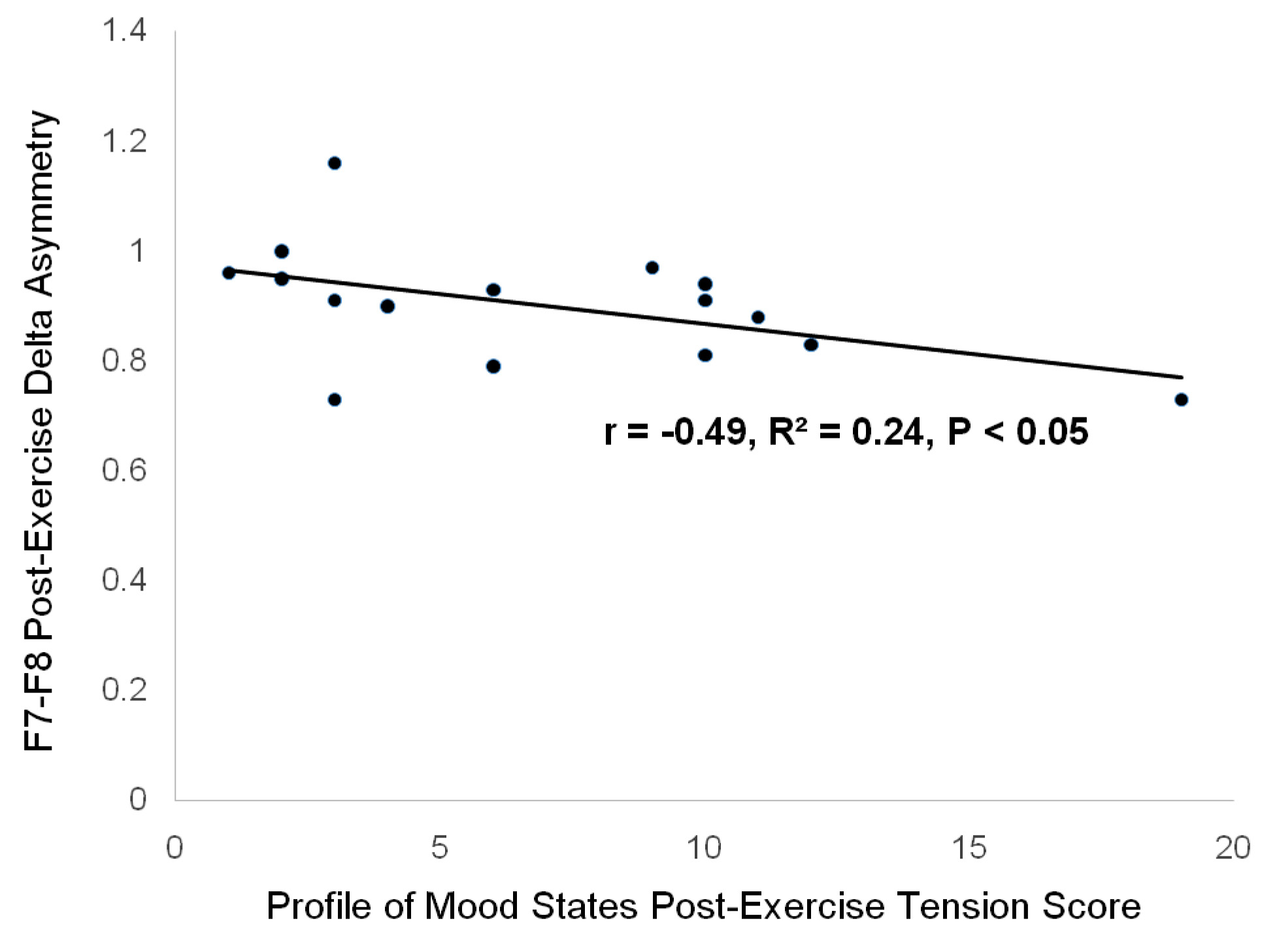
Discussion
In the present study, the alpha wave magnitude was found to be greater on the right (Fp2 and F8) than on the left for all subjects, by virtue of the positive prefrontal and inferior frontal values. Because cortical activation decreases as alpha wave magnitude increases, the positive alpha asymmetry values for the present study subjects are indicative of predominant cortical activation in the left prefrontal (Fp2) and inferior frontal (F8) cerebral hemisphere.
The main qEEG findings of this study are significant exercise-induced decrements in prefrontal delta, alpha and theta asymmetries in healthy male African-American university students, in the absence of any such changes in asymmetries in their female counterparts. Both the post-exercise tension and the total mood disturbance scores were decreased for this mixed-gender cohort. However, the post-exercise depression scores were decreased only for the females, suggesting the possibility of a gender-related difference.
Bouts of resting and aerobic exercising conditions (post-control, post-exercise) were evaluated at the same relative workloads across all subjects. We controlled for inter-individual differences in exercise intensity as a potential factor influencing the emotional responses of the study subjects by requiring all subjects to exercise at an intensity of 60% of their predetermined VO2peak, in accordance with American College of Sports Medicine guidelines for exercise testing [29]. We considered using ventilatory threshold, recommended by Ekkekakis and associates [32], but ventilatory threshold is reported to be highly variable with respect to VO2peak in a cohort of sedentary subjects; therefore, was not expected to be a reliable indicator of exercise intensity in our sedentary subjects.
Lower tension, depression and total mood scores are reported to reflect an improvement in mood post-exercise [33]. In a longitudinal study, increased aerobic fitness and habitual physical activity are shown to be associated with fewer symptoms of depression and increased emotional balance [34]. In that regard, the emergence of depression appears to be associated with transition from a physically-active to a sedentary lifestyle [35]. Evidence is also emerging that exercise is an effective treatment for depression [36]. Yoga is also shown to lower anxiety, depression and anger [37,38]. These findings suggest that the decrements in total mood disturbance, tension and depression which we found are consistent with mood changes generally reported for exercise.
In the present study, all the subjects exhibited positive asymmetries at rest which decreased after aerobic exercise, but nevertheless remained positive. By our method of computation, positive asymmetry values indicate greater EEG power in the right cerebral hemisphere, underlying Fp2 and F8. Marginally significant (p=0.07) decrements in prefrontal theta and prefrontal alpha power were found, with a significant (p<0.05) decrement in prefrontal delta power. Taken together, the trends of decreased power in the delta, theta and alpha bandwidths suggest that there were decreases in amplitudes of these slow brain waves on the right and/or increases in these amplitudes on the left. Amplitudes of fast prefrontal and frontal beta and gamma brain waves are shown to be positively correlated with cortical activation; however, amplitudes of the slow prefrontal/frontal theta and alpha are negatively correlated with activation [13,14,39]. The delta waves have not been systematically studied, but evidence is emerging that prefrontal/frontal delta amplitude is correlated with motor inhibition and interference with mental attention/concentration [40]. In summary, the present and previous studies suggest that individuals possessing EEG asymmetries, both left- and right-sided, may exhibit greater emotional responsiveness than individuals without such asymmetries.
The findings of the present study further suggest the hypothesis that the interconnectivity between left and right prefrontal or inferior frontal cortices may be linked with changes in mood, post-exercise. We observed a significant post-exercise decrease in prefrontal alpha coherence for the female subjects which may reflect such linkage with interconnectivity. This result is one of the more interesting findings of this study. The post-exercise decrement in prefrontal alpha coherence associated with a decrement in prefrontal alpha asymmetry in the female subjects suggests that less communication in the alpha bandwidth was likely associated with more balanced right-left cortical activation. This, in turn, supports the notion that asymmetrical cortical activation of hubs may serve an important cognitive information-integrating function. Thus, such asymmetry may provide an important neurophysiological stimulus for improving interconnectivity between information processing hubs in the human brain. Indeed, evidence is emerging that asymmetry is a normal anatomical feature of the cerebral hemispheres in primates [41] and that parallel changes in interhemispheric interconnectivity with development of multifarious cytoarchitectures might be required to keep up with the information processing demands of large, specialized cerebral hemispheres [42].
We also found a mixture of significant negative and positive correlations between mood and frontal asymmetries. The relevant findings include significant negative correlation between the total mood and prefrontal beta asymmetry, across the post-rest and post-exercise conditions. This suggests that low mood score was associated with higher activation of the right prefrontal cortex, consistent with what is known about right-sided asymmetries. There also was a significant positive correlation between confusion and prefrontal beta asymmetry during the post-control resting condition. This finding suggests that greater confusion, a negative emotion, was associated with greater activation of the prefrontal cortex. On the other hand, the finding that confusion and tension scores were positively correlated with inferior frontal delta asymmetry after exercise indicates that these negative emotions were associated with more-left-sided activation after exercise. This suggests the hypothesis that mood changes are associated with aerobic exercise might be bandwidth- and site-specific and also as a result of interhemispheric redistribution of blood flow which is, no doubt, worthy of further study. The positive correlations for negative aspects of mood (confusion and tension scores) and positive correlations for a positive aspect of mood (vigor score) and the inferior frontal (F7, F8) beta, theta and delta asymmetries found post-exercise suggest a complex relationship between interhemispheric asymmetry and mood at the inferior frontal cortex.
Limitation
The main limitation of this study is that it was based on a limited number of subjects and had low statistical power, which creates conditions for type 2 statistical errors; hence, the results of this study should be interpreted cautiously pending a future study with a larger number of subjects. There were several exercise-induced asymmetries and coherences that were found to be marginally significant, which should be statistically significant in a study based on a larger number of subjects. For example: the significant exercise-induced decrease in alpha coherence in the females was accompanied by a trend toward decrease in alpha coherence in the males (p=0.1).
Conclusion
In our experience, some individuals exhibit negative emotional responses to exercise which would need to be overcome in order to include regular exercise for health maintenance; especially, to avoid overweight and obesity. The main results of this study demonstrate trends toward improvements in mood and changes in prefrontal/inferior frontal brain wave symmetry after exercise which appears to be gender-specific. Future studies should be designed to evaluate EEG asymmetry in conjunction with exercise-induced mood changes in larger, more diverse populations.
TMD = Total Mood DisturbanceData in means±standard errors; *Differences significant at p≤ 0.05, F≥3.78Note: Immediately after 30 minutes of exercise condition and control condition participants completed electronically administered Profile of Mood States QuestionnaireD = Delta; A = Alpha; T = Theta; B = BetaData in means±standard error; *Difference significant at p≤ 0.05, F≥ 3.78Asym = AsymmetryCohe = CoherenceD = Delta; A = Alpha; T = Theta; B = BetaData in means±standard errors; *Difference significant at p≤ 0.07, F≥ 3.28Asym = AsymmetryCohe = CoherenceAll correlations significant at p≤ 0.05.
[1]. Dennis TA, Solomon B, Frontal EEG and emotion regulation: electrocortical activity in response to emotional film clips is associated with reduced mood induction and attention interference effects Biological psychol 2010 85(3):456-64.10.1016/j.biopsycho.2010.09.00820863872 [Google Scholar] [CrossRef] [PubMed]
[2]. Petruzzello SJ, Hall EE, Ekkekakis P, Regional brain activation as a biological marker of affective responsivity to acute exercise: influence of fitness Psychophysiol 2001 38(1):99-106.10.1111/1469-8986.3810099 [Google Scholar] [CrossRef]
[3]. Hall EE, Ekkekakis P, Petruzzello SJ, Predicting affective responses to exercise using resting EEG frontal asymmetry: does intensity matter? Biol Psychol 2010 83(3):201-06.10.1016/j.biopsycho.2010.01.00120064586 [Google Scholar] [CrossRef] [PubMed]
[4]. Woo M, Kim S, Kim J, Petruzzello SJ, Hatfield BD, Examining the exercise-affect dose-response relationship: does duration influencefrontal EEG asymmetry? Int J Psychophysiol 2009 72(2):166-72.10.1016/j.ijpsycho.2008.12.00319110012 [Google Scholar] [CrossRef] [PubMed]
[5]. Woo M, Kim S, Kim J, Petruzzello SJ, Hatfield BD, The influence of exercise intensity on frontal electroencephalographic asymmetry and self-reported affect Res Q Exerc Sport 2010 81(3):349-59.10.1080/02701367.2010.1059968320949855 [Google Scholar] [CrossRef] [PubMed]
[6]. Gollan JK, Hoxha D, Chihade D, Pflieger ME, Rosebrock L, Cacioppo J, Frontal alpha EEG asymmetry before and after behavioural activation treatment for depression Biological psychol 2014 99:198-208.10.1016/j.biopsycho.2014.03.00324674708 [Google Scholar] [CrossRef] [PubMed]
[7]. Ng R, Fishman I, Bellugi U, Frontal asymmetry index in Williams syndrome:Evidence for altered emotional brain circuitry? Social Neurosci 2015 10(4):366-75./10.1080/17470919.2015.100566725633341 [Google Scholar] [CrossRef] [PubMed]
[8]. Feng X, Forbes EE, Kovacs M, George CJ, Lopez-Duran NL, Fox NA, Children’s depressive symptoms in relation to EEG frontal asymmetry and maternal depression J Abnorm Child Psychol 2012 40(2):265-76.10.1007/s10802-011-9564-921894523 [Google Scholar] [CrossRef] [PubMed]
[9]. Zotev V, Yuan H, Misaki M, Phillips R, Young KD, Feldner MT, Correlation between amygdala BOLD activity and frontal EEG asymmetry during real-time fMRI neurofeedback training in patients with depression Neuroimage Clin 2016 11:224-38.10.1016/j.nicl.2016.02.00326958462 [Google Scholar] [CrossRef] [PubMed]
[10]. Knyazev GG, Slobodskoj-Plusnin JY, Bocharov AV, Event-related delta and theta synchronization during explicit and implicit emotion processing Neuroscience 2009 164(4):1588-600.10.1016/j.neuroscience.2009.09.05719796666 [Google Scholar] [CrossRef] [PubMed]
[11]. Lee YY, Hsieh S, Classifying different emotional states by means of EEG-based functional connectivity patterns PLoS One 2014 9(4):e9541510.1371/journal.pone.009541524743695 [Google Scholar] [CrossRef] [PubMed]
[12]. Cook IA, O’Hara R, Uijtdehaage SH, Mandelkern M, Leuchter AF, Assessing the accuracy of topographic EEG mapping for determining local brain function Electroencephalogr Clin Neurophysiol 1998 107(6):408-14.10.1016/S0013-4694(98)00092-3 [Google Scholar] [CrossRef]
[13]. Goldman RI, Stern JM, Engel J Jr, Cohen MS, Acquiring simultaneous EEG and functional MRI ClinNeurophysiol 2000 111(11):1974-80.10.1016/S1388-2457(00)00456-9 [Google Scholar] [CrossRef]
[14]. Ritter P, Villringer A, Simultaneous EEG–fMRI, Neurosci Biobehav Rev 2006 30(6):823-38.10.1016/j.neubiorev.2006.06.00816911826 [Google Scholar] [CrossRef] [PubMed]
[15]. Spielberg JM, Stewart JL, Levin RL, Miller GA, Heller W, Prefrontal Cortex, Emotion, and Approach/Withdrawal Motivation Social and Personality Psychol Compass 2008 2(1):135-53.10.1111/j.1751-9004.2007.00064.x20574551 [Google Scholar] [CrossRef] [PubMed]
[16]. Santesso DL, Segalowitz SJ, Ashbaugh AR, Antony MM, McCabe RE, Schmidt LA, Frontal EEG asymmetry and sensation seeking in young adults Biological Psychol 2008 78:164-72.10.1016/j.biopsycho.2008.02.00318367308 [Google Scholar] [CrossRef] [PubMed]
[17]. Manna CB, Tenke CE, Gates NA, Kayser J, Borod JC, Stewart JW, EEG hemispheric asymmetries during cognitive tasks in depressed patients with high versus low trait anxiety Clin EEG Neurosci 2010 41(4):196-202.10.1177/15500594100410040621077571 [Google Scholar] [CrossRef] [PubMed]
[18]. Tomarken AJ, Davidson RJ, Wheeler RE, Doss RC, Individual differences in anterior brain asymmetry and fundamental dimensions of emotion J PersSoc Psychol 1992 62(4):676-87.10.1037//0022-3514.62.4.6761583591 [Google Scholar] [CrossRef] [PubMed]
[19]. McAllister EJ, Dhurandhar NV, Keith SW, Aronne LJ, Barger J, Baskin M, Ten putative contributors to the obesity epidemic Crit RevFood Sci Nutr 2009 49(10):868-913.10.1080/1040839090337259919960394 [Google Scholar] [CrossRef] [PubMed]
[20]. Booth FW, Roberts CK, Laye MJ, Lack of exercise is a major cause of chronic diseases Comprehensive Physiol 2012 2(2):1143-211.10.1002/cphy.c110025 [Google Scholar] [CrossRef]
[21]. Childs E, de Wit H, Regular exercise is associated with emotional resilience to acute stress in healthy adults Frontiers in Physiol 2014 5:16110.3389/fphys.2014.0016124822048 [Google Scholar] [CrossRef] [PubMed]
[22]. Anderson E, Shivakumar G, Effects of exercise and physical activity on anxiety Frontiers in Psychiatry 2013 4:2710.3389/fpsyt.2013.0002723630504 [Google Scholar] [CrossRef] [PubMed]
[23]. Gorantla VR, Sirigiri A, Volkova YA, Millis RM, Effects of swimming exercise on limbic and motor cortex neurogenesis in the kainate-lesion model of temporal lobe epilepsy Cardiovasc Psychiatry Neurol 2016 2016:391576710.1155/2016/391576727313873 [Google Scholar] [CrossRef] [PubMed]
[24]. Cominski TP, Jiao X, Catuzzi JE, Stewart AL, Pang KC, The role of the hippocampus in avoidance learning and anxiety vulnerability Front Behav Neurosci 2014 8:27310.3389/fnbeh.2014.0027325152721 [Google Scholar] [CrossRef] [PubMed]
[25]. Moore CG, Carter RE, Nietert PJ, Stewart PW, Recommendations for planning pilot studies in clinical and translational research Clinical and Translational Sci 2011 4(5):332-37.10.1111/j.1752-8062.2011.00347.x22029804 [Google Scholar] [CrossRef] [PubMed]
[26]. Endicott J, The menstrual cycle and mood disorders J Affect Disord 1993 29(2-3):193-200.10.1016/0165-0327(93)90033-G [Google Scholar] [CrossRef]
[27]. McNeil JK, Droppleman LF, Loor M, Manual for thew profile of mood states: POMS San Diego Educational and Industrial Testing Service 1971 [Google Scholar]
[28]. Ekkekakis P, Petruzzello SJ, Acute aerobic exercise and affect: current status, problems and prospects regarding dose-response Sports Med 1999 28(5):337-74.10.2165/00007256-199928050-0000510593646 [Google Scholar] [CrossRef] [PubMed]
[29]. American College of Sports Medicine ACSM’s guidelines for exercise testing and prescription 2005 7th EditionPhiladelphia, PALippincott, Williams, & Wilkins [Google Scholar]
[30]. McNair DM, Droppleman LF, Lorr M, Edits Manual for the Profile of Mood States; POMS Edits 1992 [Google Scholar]
[31]. McDowell CP, Campbell MJ, Herring MP, Sex-related differences in mood responses to acute aerobic exercise Med Sci Sports Exerc 2016 48(9):1798-802.10.1249/MSS.000000000000096927128666 [Google Scholar] [CrossRef] [PubMed]
[32]. Ekkekakis P, Lind E, Joens-Matre RR, Can self-reported preference for exercise intensity predict physiologically defined self-selected exercise intensity? Res Q Exerc Sport 2006 77(1):81-90.10.1080/02701367.2006.1059933416646355 [Google Scholar] [CrossRef] [PubMed]
[33]. Yoshihara K, Hiramoto T, Sudo N, Kubo C, Profile of mood states and stressrelated biochemical indices in long-term yoga practitioners Biopsychosoc Med 2011 5(1):610.1186/1751-0759-5-621635790 [Google Scholar] [CrossRef] [PubMed]
[34]. Galper DI, Trivedi MH, Barlow CE, Dunn AL, Kampert JB, Inverse association between physical inactivity and mental health in men and women Med Sci Sports Exerc 2006 38(1):173-78.10.1249/01.mss.0000180883.32116.2816394971 [Google Scholar] [CrossRef] [PubMed]
[35]. van Gool CH, Kempen GI, Penninx BW, Deeg DJ, Beekman AT, van Eijk JT, Relationship between changes in depressive symptoms and unhealthy lifestyles in late middle aged and older persons: results from the Longitudinal Aging Study Amsterdam Age Ageing 2003 32(1):81-87.10.1093/ageing/32.1.8112540353 [Google Scholar] [CrossRef] [PubMed]
[36]. Cooney GM, Dwan K, Greig CA, Lawlor DA, Rimer J, Waugh FR, Exercise for depression Cochrane Database Syst Rev 2013 12(9):CD00436610.1002/14651858.CD004366.pub624026850 [Google Scholar] [CrossRef] [PubMed]
[37]. Michalsen A, Grossman P, Acil A, Lanqhorst J, Ludtke R, Esch T, Rapid stress reduction and anxiolysis among distressed women as a consequence of a three-month intensive yoga program Med Sci Monit 2005 11:CR555-61. [Google Scholar]
[38]. Lavey R, Sherman T, Mueser KT, Osborne DD, Currier M, Wolfe R, The effects of yoga on mood in psychiatric inpatients Psychiatr Rehabil J 2005 28:399-402.10.2975/28.2005.399.40215895926 [Google Scholar] [CrossRef] [PubMed]
[39]. Michels L, Bucher K, Lüchinger R, Klaver P, Martin E, Jeanmonod D, Simultaneous EEG-fMRI during a working memory task: modulations in low and high frequency bands PLoS ONE 2010 5(4):e1029810.1371/journal.pone.001029820421978 [Google Scholar] [CrossRef] [PubMed]
[40]. Harmony T, The functional significance of delta oscillations in cognitive processing Front Integr Neurosci 2013 7:8310.3389/fnint.2013.0008324367301 [Google Scholar] [CrossRef] [PubMed]
[41]. Atkinson EG, Rogers J, Cheverud JM, Evolutionary and developmental implications of asymmetric brain folding in a large primate pedigree Evolution; International Journal of Organic Evolution 2016 70(3):707-15.10.1111/evo.1286726813679 [Google Scholar] [CrossRef] [PubMed]
[42]. Wang D, Liu H, Functional connectivity architecture of the human brain: not all the same. The Neuroscientist: A Review Journal Bringing Neurobiology Neurology and Psychiatry 2014 20(5):432-38.10.1177/107385841454329025030990 [Google Scholar] [CrossRef] [PubMed]