Introduction
Autism is an umbrella term for a wide spectrum of disorders known as ASDs or Pervasive Developmental Disorders (PDDs) which are manifested as impairments in central nervous system that result in difficulty in speech, abnormal response to stimuli, poor eye contact, and repetitive behaviour in kids [Table/Fig-1] [1-5]. Recent surveys have suggested that about 60 per 10,000 kids suffer from PDD [6]. A significantly high prevalence of ASD has been observed amongst males as compared to females. A male-to-female gender ratio of 4:1 has been reported for ASD [7]. The typical male to female ratio points to a genetic origin of autism. Studies show that male and female brains are different anatomically and functionally. These differences begin to develop prenatally and mature by adulthood. Sexual differentiation is mainly determined by chromosomal, genetic and hormonal factors. Many researchers believe that autism is caused by imbalance of steroid hormones in early stages of embryonic development. Neurosteroids such as testosterone, oestrogen, androgen, progesterone and oestradiol play an important role in development of cerebellum [8-14]. Scientific data obtained in a study proved that autistic offspring had extensively higher concentrations of many steroid hormones in saliva as compared to the normal children [12].
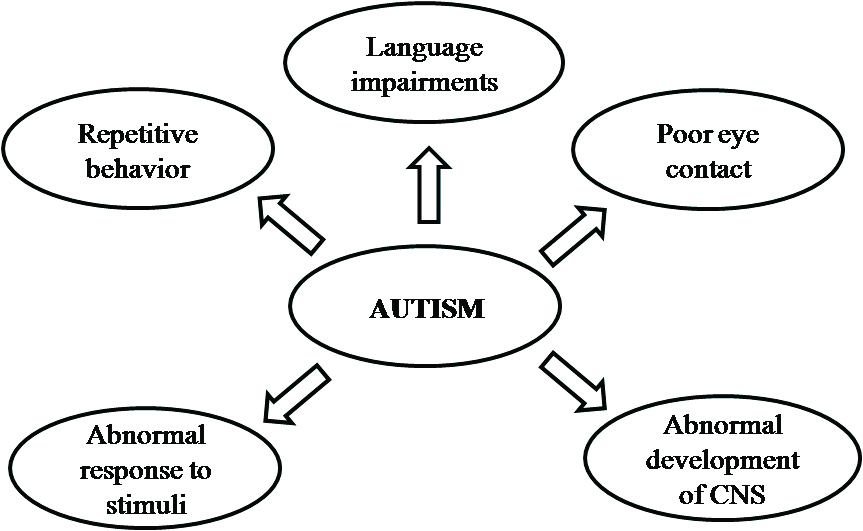
In human beings, a female possess superior ability in language related skills whereas males suffer from a higher incidence of social and communicative difficulty. These observations have given birth to extreme male brain theory which proposes that higher testosterone exposures during prenatal and neonatal developmental stage have a profound effect on sexual dimorphism in human behaviour and ASDs [15]. In this study, an effort has been put forth to highlight the implications of hormone, genetic variation and differential brain growth as well as the effect of their intra-associated events on gender bias in manifestation of ASD.
Causes and Risk Factors Associated with Asd
It has been suggested that ASD is caused by a complex interplay of genetic, environmental and biological factors [Table/Fig-2,Table/Fig-3] [16-36].
Autism related risk factors and their effects.
Risk factors | Effects | Reference |
---|
Environmental |
Advanced Paternal age | The advanced maternal (≥35 years) or paternal (≥40 years) has been associated with higher incidence of the autism. However, higher risk is associated with advanced maternal age. | [16] |
Air pollution | Maternal exposure to air pollution particularly, high concentration of fine particulate matter less than 2.5μ during pregnancy, particularly the third trimester, was associated with greater odds of a child having ASD. | [17] |
Pesticide/ Insecticide exposure | Gestational exposure to pesticides such as organophosphates, insecticides such as pyrethroides and carbamates have been associated with greater incidence of ASD. | [18] |
Heavy Metal and toxins | The accumulation of heavy metals viz. aluminum, lead, and mercury in ASD individuals. High intrauterine concentrations of heavy metals lead to developmental delays and deficits in motor performance and social behaviour. | [19,20] |
Nutritional deficiency | Zinc (Zn2+) deficiency results in irrecoverable impairment of learning and memory. Women taking folic acid supplementation during first trimester of pregnancy have been known to reduce the incidence of autism. Higher incidence of vitamin D deficiency has been reported amongst the infants with ASD. Vitamin A deficiency has been found to be prevalent amongst the ASD children. | [21-24] |
Hormonal |
Testosterone | Brain arousal is enhanced in young male animals. Results in hypersensitisation of amygdala. | [8] |
Progesterone | Low level adversely affects the development of the blood brain barrier. Affects the development of the amygdale (involved in social circuitry). | [25,26] |
Oestrogen | Decrease in CYP19A1 could also to lead to less secretion of estradiol which results in high levels of testosterone. Lack of oestrogen causes hyperactivity of infundibular nucleus. | [11] |
Genetic |
SHANK3 | 22q13.3 deletion in this gene is characterised by severe expressive language and speech delay, hypotonia, global developmental delay, and autistic behaviour. Mutations had an effect on dendritic spine morphology and synaptic transmission. | [27,28] |
PTEN | Show widespread brain overgrowth and impaired social behaviour. Increases in cortical white matter and a distinctive cognitive profile in patients. Characterised by delayed language development, poor working memory and processing speed. | [29,30] |
GABRB3 (GABAA receptor β3 subunit gene) | With the Gabrb3 m-/p+ mutation, which is predicted to increase inhibitory drive from Purkinje cells, CbN neurons in male mice upregulate their mGluR1/5 responses to synaptic stimulation, whereas females do not. Defect causes synaptic dysfunction. Alterations in phosphorylation may contribute to the pathophysiology of ASDs. | [31-33] |
RELN | Haploin sufficiency cause cognitive impairment in rodents and also related to the higher brain functions. Mutations are associated with autosomal recessive lissencephaly with cerebellar hypoplasia. Epigenetic regulation results in behavioural alterations by the induction of LTP in them at PFC. | [34,35] |
GRIN2B | Disruption leads to receptor trafficking defects, NMDAR hypofunction, and alteration of endogeneous modulator affinities. | [36] |
Complex aetiology of autism spectrum disorders. The structure and functional differences in male and female brain in relation to ASD.
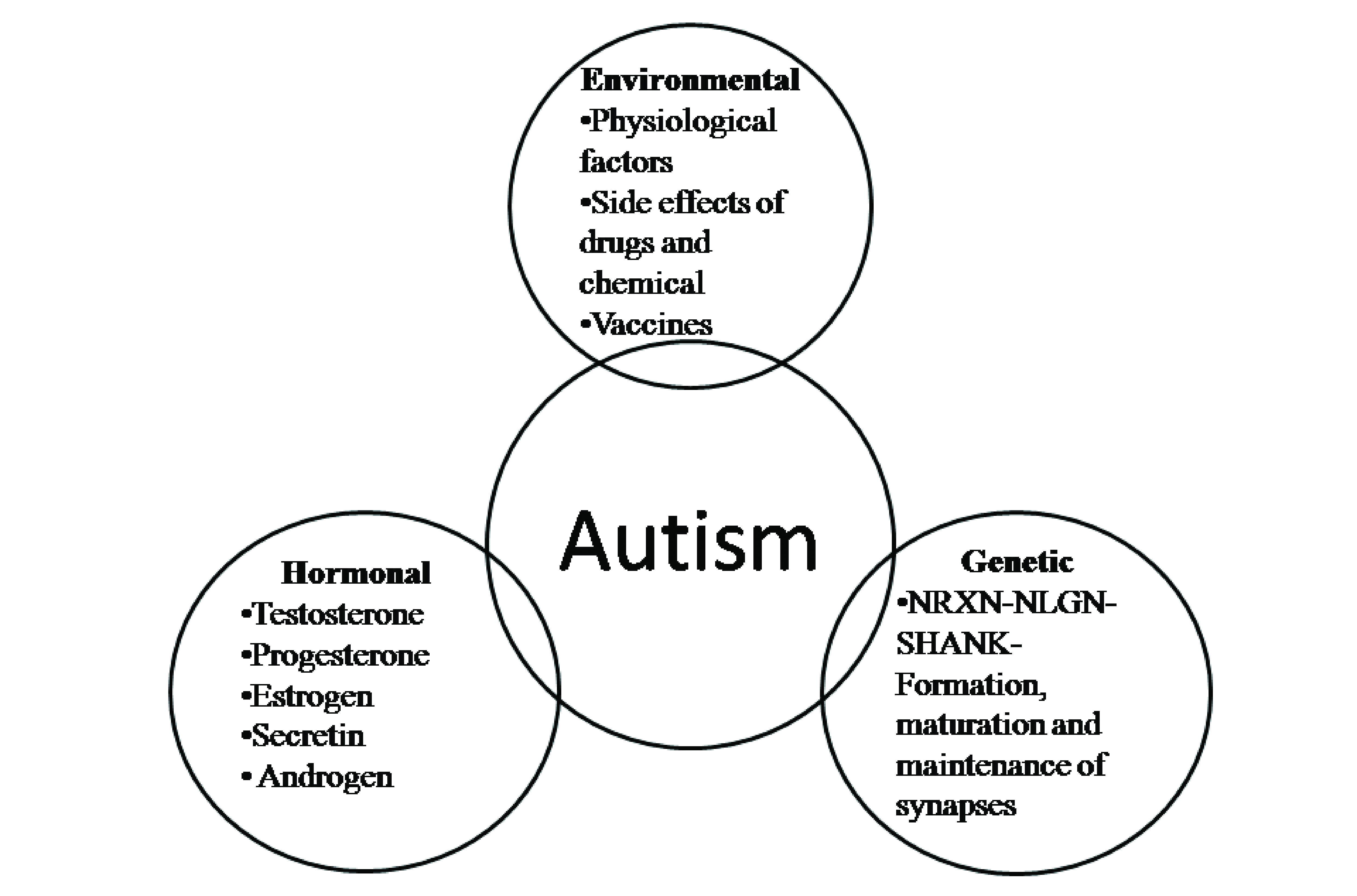
Environmental Factors
It was thought that environmental factors trigger the expression of ASD for the subjects that possess the genetic predisposition. The role of environmental factors contributing to ASD is still inconclusive [37-42]. The environmental factors frequently studied in association with ASD were physiological (parental age, birth trauma), chemical factors and air pollutants. Studies that show association between exposure to heavy metals such as lead, mercury, cadmium and arsenic are incidence of ASD [43]. There are various environmental factors which may have a profound effect on neuronal development during pregnancy.
Genetics
Prominently, mutations in SHANK gene family (SHANK1, SHANK2 and SHANK3) are associated with the development of ASD. These genes encode for the scaffolding proteins located at the Postsynaptic Density (PSD) of glutamatergic synapses and are required for proper formation and functioning of the neural synapses [44]. Mutations in the genes encoding NRXN1, NLGN3, and NLGN4 in the patients of ASD have been reported recently [45]. The Neuexin – Neuroligin –Shank (NRXN-NLGN-SHANK) pathway plays a pivotal role in the formation, maturation and maintenance of synapses. However, the relationship between these mutations and ASD has not been established completely as these mutations have also been found in non symptomatic carriers in the same family. Moreover, same mutations can be associated with quite different phenotypes in different people [46].
Genes such as the SHANK family, Reelin (RELN), Human serotonin transporter (SLC6A4), Gamma-aminobutyric Acid Receptor (GABR), Neuroligin (NLGN), Human Oxytocin Receptor (OXTR), MET, SLC25A12, Glutamate Receptor Gene (GRIK1 or GluR6), Glyoxalase 1, PTEN, GRIN2B have been implicated in ASD. SHANK1 locus is on chromosome 19 and known to induce the enlargement of spine heads [44,47]. SHANK genes cause alterations in synaptic morphology, signaling and behaviour characteristics [48]. A hemizygous SHANK1 deletion results in male carriers but not female carriers to have ASD [44]. Loss of a functional copy of the SHANK3 gene can lead to the neurobehavioural manifestations of 22q13 deletion syndrome and or might give rise to ASD [49]. These genes affect the main parts of brain, social behaviour and many other things. Though several genes have been proven to be associated the aetiology is complex and it is hypothesised that there is a complex interplay between the environmental and genetic factors in causation of ASD.
Biological Factors
As discussed in the previous section, a strong male predominance is observed in ASD that has been baffling the scientific community for long. It has been suggested that the hormonal and immune processes may play a role in the male predominance in autism [50]. In Attention Deficit Hyperactivity Disorder (ADHD), an association with androgen receptor haplotypes has been discovered [51]. Sex steroid hormones such as testosterone, oestrogen and dihydrotestosterone are the hub of steroid hormones in charge for the organisation and maintenance of the sexual differentiation of the brain structures during premature development stages. Several studies carried out in different organisms demonstrate significant structural and behavioural dissimilarities in female and male brains. It has been documented that considerable differences exist in the distribution of the grey-white matter, callosal commissure, anterior commissure, cortex, hypothalamus, bed nucleus of the stria terminalis, limbic and chemical transmitter systems [52].
Sexually Dimorphic Regions of Brain and their Correlation with ASD
The males and females brains develop differently during adolescence and adulthood. It has been observed that male brains are optimised for intra-hemispheric and female brains for inter-hemispheric communication. The assessments of structure and function suggest that female brains are designed to facilitate communication between analytical and intuitive processing modes whereas male brains are structured to facilitate connectivity between perception and coordinated action [53]. Several functional Magnetic Resonance Imaging (fMRI) studies support the dominant concept that persons with ASD have poorer connectivity (or hypo-connectivity) between distant brain regions (such as the frontal and parietal lobes) and increased connectivity (or hyper-connectivity) between local brain regions (such as within the frontal lobe) [54-56]. This might be a reason for a greater incidence of ASD in male.
Broca’s area (Brodmann’s areas 44 and 45) is located in the ventral and posterior portions of the frontal lobe; it processes information coming from Wernicke’s area (Brodmann’s area 22) and converts it into a pattern for language production. In an autopsy analysis (10 men and 11 women free of neurological abnormalities), it was observed that the average volume of Broca’s area in women was approximately 20% larger than in men. This difference may be associated with fact that the women possess better language skills than men [57]. Interestingly, Brun L et al., reported mis-folding within Broca’s area as a distinctive feature of autistic disorder [58]. It is therefore expected that male subjects would suffer more sharply from ASD symptoms such as difficulty in communication.
The Bed Nucleus of Stria Terminalis (BNST) is a nucleus of the forebrain that receives projections from limbic system nuclei and sends projections to several hypothalamic and brainstem targeted areas. It may control behaviour responses to stress [59]. In a study involving 26 age-matched men and women, it was observed that the volume of the posteromedial region of the BNST is 2.5 times larger in men than that of in women. It was suggested that BNST may play a role in aggressive and sexual behaviours [60]. DeLorey TM et al., the expression of autism candidate gene Gamma Amino Butyric Acid A Receptor Beta 3 (GABRB3) subunit gene in mice heterozygous for the Gabrb3 disruption. They discovered reduction in Gabrb3 gene expression in mouse model and more so in male mouse [61]. Gender related differences in cortical regions including sulcal and gyral thickness have been confirmed in several studies [62-64]. Atypical sulcal anatomy has been reported for young autistic children [65].
The limbic system is the seat of many emotions such as fear, anger and emotions related to sexual behaviour, memory and learning. The most prominent components of the limbic system are the thalamus, hypothalamus, hippocampus and the amygdale. Moreover, it is known to be a prominent gender dimorphic region. The large nuclei present in diencephalon region of thalamus help in sensory perception as well as in limbic and motor functions regulations. By using Positron Emission Tomography (PET), it was showed that women have larger thalamic nuclei. This study was done on 120 healthy subjects including 65 women and 55 men [66]. The volumetric measurements of right and left thalamic nuclei were performed by MRI in a case control study group consisting (age range: 9-43 years). The results observed no differences between these two groups for unadjusted thalamic volumes. However, the expected linear relationship between total brain volume and thalamic volume was not observed in the individuals with autism [67].
The hypothalamus is larger in men than in women [63]. The Interstitial Nuclei of the Anterior Hypothalamus (INAH)/Sexually Dimorphic Nucleus of the Preoptic Area (SDN-POA) volume is more than twice as large in young adult men as it is in women, and contains about twice as many cells in men [68]. Kurth F et al., observed decrease in grey matter in a section of the hypothalamus in subjects with autism. This region of hypothalamus has been associated with the synthesis of behaviour modulating hormones such as oxytocin and arginine vasopressin [69]. This supports the hypothesis of abnormal hormonal system in autism and has a potential for further investigation into therapeutic approaches.
Studies have shown that the hippocampus in women is larger than in men, which has been testified by two MRI studies. The amygdale, in contrast to the hippocampus, is larger in men. An MRI study of age group of 15 boys and 15 girls reported that the amygdale was larger in boys than in girls aged 7 to 11 years [70]. The amygdale is very important for masculinised social behaviour and which is affected by testosterone. If a female is exposed to a high dose of testosterone during the neonatal period, it resulted in masculinised social behaviour [71]. A larger right hippocampal volume than typically developing controls, even after controlling for total cerebral volume was observed in autistic children [72].
Women also have a larger caudate nucleus as compared to men; this structure is located within the basal ganglia [73]. The caudate nucleus in children with autism has been observed to be enlarged. Increased size of the caudate nucleus in the basal ganglia has been associated with compulsive behaviours, difficulty with changes in routine, and stereotypical motor movements [74].
The Suprachiasmatic Nucleus (SCN) lies just above the optic chiasm; it receives direct retinal input and generates circadian rhythms. Vasopressin and Vasoactive Intestinal Polypeptide (VIP) nuclei are present in the different sub-divisions of the SCN. The VIP subnucleus of the SCN is significantly larger in young adult men than in women [75]. Interestingly, this sex difference may be reversed as the female sub-nucleus becomes larger after 40 years of age [76]. Abnormal sleep patterns have often been reported in patients with ASD [77-79] or Asperger syndrome [80-82].
Hormones that Affect Gender Bias in ASD
Many regions of brain demonstrate presence of sex hormone receptors such as androgens, oestrogen and progesterone. These hormones are known to effect the development of neuronal network, structural as well as cognitive development. Most of the psychological disorders exhibit marked difference in prevalence between the genders. For example, depression is more common in women while schizophrenia is prevalent in men. Recent research links these differences to hormones and their effect on sexually dimorphic structures in brain [83]. These are the clear indication for the involvement of sex hormones in creating a significant difference in prevalence of ASD in men and women. The immunocytochemical localisation of Oestrogen Receptors (ER) α, β and Androgen Receptors (ARs) has shown that there are indeed numerous targets for sex hormones in the adult human brain [11]. The findings that nuclear androgen and ER α and β staining in the SDN-POA was more intense in men than in women which supports the presence of sex differences in this nucleus [84,85]. In actuality, androgen and oestrogen pathways are metabolically linked together. It is possible that altered androgen and oestrogen levels could both lead, via different pathways, to alterations of cognitive, linguistic, social and emotional circuits. Testosterone affects development of callosal connections, resulting in differences in hemispheric specialisation between males and females [86].
Testosterone
Testosterone is a male sex hormone however, it is present in low concentration in women. In addition to gonads which are the primary producers of testosterone, it is also produced in brain [87]. De novo testosterone synthesis can occur starting from cholesterol or it could be produced from other steroids such as deoxycortisone or progesterone that are circulated along with blood. Testosterone after entering the cell cytoplasm binds to the nuclear ARs and gets transported to nucleus where it binds to hormone response element on resulting in either stimulation of silencing of gene expression [88]. In young male animals, brain arousal is enhanced when testosterone binds to (ARs) of brainstem neurons. This results in hypersensitisation of amygdale in young male subjects. Arousal-related inputs regulate amygdaloid mechanisms for fear and anxiety, with resultant social avoidance. In sharp contrast to boys, girls do not have high levels of testosterone-facilitated arousal-causing inputs to the amygdala and also enjoy the protection afforded by oestrogenic hormones, oxytocin, and the oxytocin receptors. This theory suggests that original technologies applied to the molecular endocrinology of testosterone’s actions through AR will offer new avenues of analysis into ASD [8]. Supported by the hypermasculine aspect, studied from the number of observation, a pathological intrauterine testosterone level is suggested to be responsible for the observed altered cerebral asymmetry and consecutive hemispheric dysfunction [9].
A second non genomic mechanism pathway for testosterone action has also been revealed in recent years. It binds to the membrane receptors leading to activation of second messengers. Moreover, testosterone can be converted to either oestradiol by aromatase or into dihydrotestosterone by reductase.
Progesterone
Progesterone plays a role in establishing neuronal connectivity [89] and cortical circuitry [90]. Obstetrical complications and the brain changes, both are associated with autism due to low level of progesterone [13]. It has been found that if it is supplied to the foetus maternally, it does not only support pregnancy but also promotes brain development. A survey of mothers of autistic children (n=86) compared to mothers of naturally-developing children (n=88) regarding obstetrical histories, including five obstetrical risk factors indicative of low progesterone. This study recommends regular monitoring of progesterone levels in risk pregnancies. A theory suggested ensuring adequate levels of progesterone may decrease the likelihood of autism. The strongest of the low progesterone factors identified was the number of women giving birth to a child with autism who experienced contraceptive failure and this similar result found by Juul-Dam N et al., [13]. Low level of progesterone adversely affects the development of the blood brain barrier and has been implicated in autism [25]. Progesterone has a protective function by maintaining the proteins involved in Blood Brain Barrier (BBB) development and function [91]. The recent work suggests that much of the network pathology is due to axonal pathology, possibly myelination deficits [92-96] and myelination is highly dependent on progesterone [97,98]. Progesterone also affects development of the amygdala, a region also involved in social circuitry [26], here progesterone receptors are highly localised in developing male brains and affect development of the medial pre-optic area of the hypothalamus [99], a region rich in oxytocin-containing neurons involved in social behaviours [100]. At a cellular level, progesterone promotes neurogenesis [101], synaptogenesis and dendritic formation [102-107], again changes which have been proposed to underlie autism [108]. Although, the foetal brain has progesterone receptors, the foetus itself does not produce progesterone and the foetal brain is thus dependent on the maternal placenta supply for organising effects to take place. A male and female foetus will receive the same amount of progesterone from the mother; however the male foetal brain expresses progesterone receptors at critical periods, whereas the female brain has very few receptors [109]. Moreover, placentas are gender-specific and the placenta of a male foetus is more programmable and more liable to alter production of progesterone [110]. Thus, the male brain or the male-placenta may be more influenced by progesterone levels than the female brain, explaining the male preponderance in autism.
Oestrogen
Oestrogens are female sex hormones that include oestrone, oestradiol and oestriol produced in the ovaries, placenta, adrenal cortex and testes in males. Oestradiol induces the masculinisation characteristics in many cases [14]. Male and female hormones differentially control the expression in autistic candidates genes, Retinoic acid-related Orphan Receptor-Alpha (RORA) in a neuronal cell line, SH-SY5Y. RORA transcriptionally regulates aromatase, it is an enzyme that converts testosterone to oestrogen. Aromatase protein is significantly reduced in the frontal cortex of autistic patients with respect to sex- and age-matched controls, and is closely correlated with RORA protein levels in the brain. These consequences suggest that RORA may be under positive or negative control of male and female hormones, respectively, through one of its transcriptional targets, aromatase, moreover suggest a mechanism for introducing sex bias in autism. The level of RORA expression can be regulated by both male and female hormones through their particular receptors and that one of its transcriptional targets is CYP19A1 (aromatase gene). In which it has been proposed that the reduction of RORA observed in autism is exacerbated by a negative feedback mechanism involving decreased aromatase level, which further causes accumulation of testosterone and reduction of oestradiol. Deficiency in RORA in autistic brain is likely to be further enhanced by increased levels of testosterone due to repression of aromatase, a transcriptional target site of RORA. Moreover, it plays a critical role in purkinje cell differentiation and cerebellar development as well as in neuroprotection against inflammation and oxidative stress. These elucidate at least some of the pathology that has been observed in autism. However, the clarification proposes that not all samples from autistic individuals are lacking in RORA, as established by previous studies with lymphoblastoid cell lines and brain tissues. On the other side, decrease in RORA has been observed in brains of male as well as female subjects with ASD indicating that the effects are not specific to any one gender. Fascinatingly, RORA and ER allocate a consensus binding site on DNA (AGGTCA) and as a result target common genes. The shared gene targets may explain the lower incidence of ASD amongst the female subjects with higher levels of oestrogen. Increasing the levels of RORA expression, or by inducing shared target genes of RORA through ER, as a result compensate the part of RORA deficiency can protect females against autism [111]. Molecular alterations within the ER signaling pathway may have a say to the sex difference in ASD, but the extent of such abnormalities in the brain is not known. ERα is involved in actions of reproductive organs and reproductive behaviour, whereas ERβ is known to arbitrate some of the effects of oestrogens on behaviours that are not particularly associated with reproduction, such as locomotors activity, fear responses, anxiety, and learning. The oestrogen-ER complex can bind directly to DNA via an oestrogen responsive element or become attached to a transcription factor. It recruits a variety of coregulators that result in the activation or repression of target genes by modifying chromatin structure. CYP19A1 expression is significantly lower in the brain of ASD subjects. Earlier studies have reported the expression of CYP19A1, the key enzyme required for oestrogen production in the cortex. In addition, CYP19A1 is enriched at synapses and localises to presynaptic structures in cortical neurons, signifying that brain-synthesised oestrogen and it has an important role in neuronal function. The decrease in CYP19A1 could lead to less production of oestradiol which results in increased levels of testosterone as observed in ASD subjects. Lack of oestrogens causes hyperactivity of infundibular nucleus [11].
Vasopressin
Vasopressin is androgen-dependent and of particular importance to male behaviour. Excess vasopressin or disruptions in the vasopressin system could contribute to the male vulnerability to ASD. On the other hand, protective processes mediated via oxytocin or the oxytocin receptor might help to elucidate the relatively rare occurrence of ASD in females. Disruptions in either oxytocin or vasopressin or their receptors could result from genetic variation or epigenetic modifications of gene expression, especially during early development [112].
Further studies should inspect the mRNA and protein levels of sex hormone receptors in the brain samples from ASD and control subjects, and such information would be helpful for better perceptive of the relationship between oestrogen-related and testosterone-related signaling pathways in ASD.
Secretin
Secretin is a gastrointestinal hormone which was first represented as an effective treatment for ASD in 1998. Secretin hormone is a 27 amino-acid polypeptide produced in the intestine. It plays a role in gastrointestinal function. There is a postulated role of secretin in decreasing immune responses in the gut lumen. Secretin receptors have also been demonstrated in the brains of rats and pigs [113]. When injected intracerebrally, it has been observed that secretin decreased the locomotor activity of rats. However, there is still an uncertainty about the role of secretin in the human brain [114].
Secretin alters the activity of structures involved in behavioural breaking in of stress adaptation and visceral reflex reactions. The physiological effects of secretin on behavioural, endocrine, autonomic and sensory neuronal activation patterns, collectively, contribute to central c-fos activation. These studies can consent for further examination of secretin as a brain/gut stress regulatory hormone [115].
Studies provide the first direct immunocytochemical demonstration of secretinergic immunoreactivity in the forebrain and provides evidence that the hypothalamus, similar to the gut, which is capable of synthesising secretin. Secretin has a twin expression in the gut and brain secretin cells, as well as its overlapping central distribution with other stress-adaptation neurohormones, particularly oxytocin, indicate that it is a stress-sensitive hormone. Colchicine-induced an upregulation of secretin that signify secretin may be synthesised on command in the response to stress, a possible mechanism of actions that may underlie secretin’s role in autism [116].
Williams K et al., carried out a systematic review of published reports on effectiveness of intravenous secretin therapy for autistic children. However, this survey was unable to establish any effectiveness of secretin in improving ASD related symptoms. Thus, it should not be recommended or administered as a treatment for ASD [117].
Studies on the exact aetiology and cure of ASDs have eluded the medical science for many decades. The studies point to a complex aetiology involving genetic, hormonal and environmental factors that affect during the neuronal development. Extensive experimental evidence based on state of art analytical tools would be required to prove the association conclusively. This would also give an insight to the cure or prevention of this complex developmental disorder.
Conclusion
In the present study, we have made a strong case for involvement of different hormones especially sex hormones to be involved in ASDs. These hormones have multiple receptors in different areas of brain and hence hormones and their receptors can prove to be targets for discovery of drugs that could treat autism.
[1]. Ozand PT, A Al-Odaib, Merza H, S Al Harbi, Autism: a review J Pediatr Neurol 2003 1(2):55-67. [Google Scholar]
[2]. F Bonnet-Brilhault, Autism: an early neurodevelopmental disorder Arch Pediatr 2017 24(4):384-90. [Google Scholar]
[3]. Hagerman RJ, Rivera SM, Hagerman PJ, The fragile X family of disorders: a model for autism and targeted treatments Curr Pediatr Rev 2008 4(1):40-52. [Google Scholar]
[4]. Kaufmann WE, Cortell R, Kau AS, Bukelis I, Tierney E, Gray RM, Autism spectrum disorder in fragile X syndrome: communication, social interaction, and specific behaviours Am J Med Genet A 2004 129(3):225-34. [Google Scholar]
[5]. Rogers SJ, Wehner EA, Hagerman R, The behavioural phenotype in fragile X: symptoms of autism in very young children with fragile X syndrome, idiopathic autism, and other developmental disorders J Dev Behav Pediatr 2001 22(6):409-17. [Google Scholar]
[6]. Fombonne E, Epidemiology of autistic disorder and other pervasive developmental disorders J Clin Pyschiatry 2005 66(Supp 10):03-08. [Google Scholar]
[7]. Fombonne E, Epidemiology of pervasive developmental disorders Pediatr Res 2009 65(6):591-98. [Google Scholar]
[8]. Pfaff DW, Rapin I, Goldman S, Male predominance in autism: neuroendocrine influences on arousal and social anxiety Autism Res 2011 4(3):163-76. [Google Scholar]
[9]. Hermle L, Oepen G, Hemispheric laterality and early childhood autism. A case study of the aetiologic and nosologic problem of the autistic syndrome in childhood Nervenarzt 1987 58(10):644-47. [Google Scholar]
[10]. , Knickmeyer, S Baron-Cohen, Fetal testosterone and sex differences Early Hum Dev 2006 82(2):755-60. [Google Scholar]
[11]. Swaab DF, Chung WC, Kruijver FP, Hofman MA, Hestiantoro A, Sex differences in the hypothalamus in the different stages of human life Neurobiol Aging 2003 24(Supp 1):S1-16. [Google Scholar]
[12]. Majewska MD, Hill M, E Rok-Bujko, P Bieńkowski, Namysowska P, Marked elevation of adrenal steroids, especially androgens, in saliva of prepubertal autistic children Eur Child Adolesc Psychiatry 2014 23(6):485-98. [Google Scholar]
[13]. N Juul-Dam, Townsend J, Courchesne E, Prenatal, perinatal, and neonatal factors in autism, pervasive developmental disorder-not otherwise specified, and the general population Pediatrics 2001 107(4):e63-63. [Google Scholar]
[14]. Dean SL, McCarthy MM, Steroids, sex and the cerebellar cortex: implications for human disease Cerebellum 2008 7(1):38-47. [Google Scholar]
[15]. S Baron-Cohen, Knickmeyer RC, Belmonte MK, Sex differences in the brain: implications for explaining autism Science 2005 310(5749):819-23. [Google Scholar]
[16]. Durkin MS, Maenner MJ, Newschaffer CJ, Lee LC, Cunniff CM, Daniels JL, Advanced parental age and the risk of autism spectrum disorder Am J Epidemiol 2008 168(11)(1):1268-76. [Google Scholar]
[17]. Raz R, Roberts AL, Lyall K, Hart JE, Just AC, Laden F, Autism spectrum disorder and particulate matter air pollution before, during, and after pregnancy: a nested case-control analysis within the Nurses’ Health Study II cohort Environ Health Perspect 2015 123(3):264-70. [Google Scholar]
[18]. Shelton JF, Geraghty EM, Tancredi DJ, Delwiche LD, Schmidt RJ, Ritz B, Neurodevelopmental disorders and prenatal residential proximity to agricultural pesticides: the CHARGE study Environ Health Perspect 2014 122(10):1103-09. [Google Scholar]
[19]. Tabatadze T, Zhorzholiani L, Kherkheulidze M, Kandelaki E, Ivanashvili T, Hair heavy metal and essential trace element concentration in children with autism spectrum disorder Georgian Med News 2015 (248):77-82. [Google Scholar]
[20]. Persico AM, Merelli S, Environmental factors in the onset of autism spectrum disorder Curr Dev Disord Rep 2014 1(1):08-19. [Google Scholar]
[21]. Grabrucker AM, Environmental factors in autism Front Psychiatry 2013 3:01-13. [Google Scholar]
[22]. P Surén, Roth C, Bresnahan M, Haugen M, Hornig M, Hirtz D, Association [22]between maternal use of folic acid supplements and risk of autism spectrum disorders in children JAMA 2013 309(6):570-77. [Google Scholar]
[23]. Bener A, Khattab AO, MM Al-Dabbagh, Is high prevalence of vitamin D deficiency evidence for autism disorder? In a highly endogamous population J Pediatr Neurosci 2014 9(3):227-33. [Google Scholar]
[24]. Liu X, Liu J, Xiong X, Yang T, Hou N, Liang X, Correlation between nutrition and symptoms: nutritional survey of children with autism spectrum disorder in Chongqing, China Nutrients 2016 8(5):294 [Google Scholar]
[25]. Theoharides TC, Zhang B, Neuro-inflammation, blood-brain barrier, seizures and autism J. Neuroinflammation 2011 8:168 [Google Scholar]
[26]. Breton AB, Austin KJ, Leedy MG, Alexander BM, Effects of progesterone and RU486 on the development and expression of adult male sexual behaviour and gene expression in the amygdala and preoptic area of the hypothalamus Reprod Fertil Dev 2012 24(7):916-22. [Google Scholar]
[27]. Uchino S, Waga C, SHANK3 as an autism spectrum disorder-associated gene Brain Dev 2013 35(2):106-10. [Google Scholar]
[28]. Durand CM, Betancur C, Boeckers TM, Bockmann J, Chaste P, Fauchereau F, Mutations in the gene encoding the synaptic scaffoldingprotein SHANK3 are associated with autism spectrum disorders Nat Genet 2007 39(1):25-27. [Google Scholar]
[29]. AE Clipperton Allen, Chen Y, Page DT, Autism relevant behaviours are minimally impacted by conditional deletion of Pten in oxytocinergic neurons Autism Res 2016 9(12):1248-62. [Google Scholar]
[30]. Tilot AK, Frazier TW, Eng C, Balancing proliferation and connectivity in PTEN-associated autism spectrum disorder Neurotherapeutics 2015 12(3):609-19. [Google Scholar]
[31]. Mercer AA, Palarz KJ, Tabatadze N, Woolley CS, Raman IM, Sex differences in cerebellar synaptic transmission and sex-specific responses to autism-linked Gabrb3 mutations in mice E Life 2016 5:e07596 [Google Scholar]
[32]. Delahanty RJ, Kang JQ, Brune CW, Kistner EO, Courchesne E, Cox NJ, Maternal transmission of a rare GABRB3 signal peptide variant is associated with autism Mol Psychiatry 2011 16(1):86-96. [Google Scholar]
[33]. Vien TN, Modgil A, Abramian AM, Jurd R, Walker J, Brandon NJ, Compromising the phosphodependent regulation of the GABAAR β3 subunit reproduces the core phenotypes of autism spectrum disorders Proc Natl Acad Sci USA 2015 112(48):14805-10. [Google Scholar]
[34]. Hong SE, Huang YY, Cooley DT, S Al Shahwan, Grant PE, Hourihane JO, Autosomal recessive lissencephaly with cerebellar hypoplasia is associated with human RELN mutations Nat Genet 2000 26(1):93-96. [Google Scholar]
[35]. Ishii K, Kubo KI, Nakajima K, Reelin and neuropsychiatric disorders Front Cell Neurosci 2016 10:229 [Google Scholar]
[36]. Hu C, Chen W, Myers SJ, Yuan H, Traynelis SF, Human GRIN2B variants in neurodevelopmental disorders J Pharmacol Sci 2016 132(2):115-21. [Google Scholar]
[37]. Currenti SA, Understanding and determining the aetiology of autism Cell Mol Neurobiol 2010 30(2):161-71. [Google Scholar]
[38]. Rossignol DA, Genuis SJ, Frye RE, Environmental toxicants and autism spectrum disorders: a systematic review Transl Psychiatry 2014 4:e360 [Google Scholar]
[39]. Rossignol DA, Frye RE, A review of research trends in physiological abnormalities in autism spectrum disorders: immune dysregulation, inflammation, oxidative stress, mitochondrial dysfunction and environmental toxicant exposures Mol Psychiatry 2012 17(4):389-401. [Google Scholar]
[40]. Guinchat V, Thorsen P, Laurent C, Cans C, Bodeau N, Cohen D, Pre, peri and [40]neonatal risk factors for autism Acta Obstet Gynecol Scand 2012 91(3):287-300. [Google Scholar]
[41]. Landrigan PJ, What causes autism? Exploring the environmental contribution Curr Opin Pediatr 2010 22(2):219-25. [Google Scholar]
[42]. Curtis LT, Patel K, Nutritional and environmental approaches to preventing and treating autism and Attention Deficit Hyperactivity Disorder (ADHD): a review J Altern Complement Med 2008 14(1):79-85. [Google Scholar]
[43]. Ng M, JG de Montigny, Ofner M, Do MT, Environmental factors associated with autism spectrum disorder: a scoping review for the years 2003-2013 Health Promot Chronic Dis Prev Can 2017 37(1):01-23. [Google Scholar]
[44]. Sato D, Lionel AC, Leblond CS, Prasad A, Pinto D, Walker S, SHANK1 deletions in males with autism spectrum disorder Am J Hum Genet 2012 90(5):879-87. [Google Scholar]
[45]. Onay H, Kacamak D, Kavasoglu AN, Akgun B, Yalcinli M, Kose S, Mutation analysis of the NRXN1 gene in autism spectrum disorders Balkan J Med Genet 2016 19(2):17-22. [Google Scholar]
[46]. TC Südhof, Neuroligins and neurexins link synaptic function to cognitive disease Nature 2008 455(7215):903-11. [Google Scholar]
[47]. Leblond CS, Nava C, Polge A, Gauthier J, Huguet G, Lumbroso S, Meta-analysis of SHANK mutations in autism spectrum disorders: a gradient of severity in cognitive impairments PLoS Genet 2014 10(9):e1004580 [Google Scholar]
[48]. Singla K, Baruah A, Chapadgaonkar SS, Das N, A review on approaches to [48]diagnose the autism spectrum disorder: the need of the hour Int J Adv Res 2017 5(10):695-709. [Google Scholar]
[49]. Bozdagi PA, Sakurai PA, Papapetrou PA, Wang PA, Dickstein C, Takahashi J, Haplo insufficiency of the autism-associated Shank3 gene leads to deficits in synaptic function, social interaction, and social communication Mol Autism 2010 1(1):15 [Google Scholar]
[50]. Becker KG, Male gender bias in autism and pediatric autoimmunity Autism Res 2012 5(2):77-83. [Google Scholar]
[51]. Comings DE, Chen C, Wu S, Muhleman D, Association of the androgen receptor gene (AR) with ADHD and conduct disorder Neuroreport 1999 10(7):1589-92. [Google Scholar]
[52]. S Karaismailoğlu, Erdem A, The effects of prenatal sex steroid hormones on sexual differentiation of the brain J Turk Ger Gynecol Assoc 2013 14(3):163-67. [Google Scholar]
[53]. Ingalhalikar M, Smith A, Parker D, Satterthwaite TD, Elliott MA, Ruparel K, Sex differences in the structural connectome of the human brain Proc Natl Acad Sci USA 2014 111(2):823-28. [Google Scholar]
[54]. Andreasen NC, Cohen G, Harris G, Cizadlo T, Parkkinen J, Rezai K, Image processing for the study of brain structure and function: problems and programs J Neuropsychiatry Clin Neurosci 1992 4(2):125-33. [Google Scholar]
[55]. Andreasen NC, Cizadlo T, Harris G, Swayze V, DS O’leary, Cohen G, Voxel processing techniques for the antemortem study of neuroanatomy and neuropathology using magnetic resonance imaging J Neuropsychiatry Clin Neurosci 1993 5(2):121-30. [Google Scholar]
[56]. Andreasen NC, Rajarethinam R, Cizadlo T, Arndt VW, Swayze S, Flashman LA, Automatic atlas-based volume estimation of human brain regions from MR images J Comput Assist Tomogr 1996 20(1):98-106. [Google Scholar]
[57]. Harasty J, Double KL, Halliday GM, Kril JJ, McRitchie DA, Language-associated cortical regions are proportionally larger in the female brain Arch Neurol 1997 54(2):171-76. [Google Scholar]
[58]. Brun L, Auzias G, Viellard M, Villeneuve N, Girard N, Poinso F, Localized misfolding within Broca’s area as a distinctive feature of autistic disorder Biol Psychiatry Cogn Neurosci Neuroimaging 2016 1(2):160-68. [Google Scholar]
[59]. McElligott ZA, Winder DG, Modulation of glutamatergic synaptic transmission in the bed nucleus of the striaterminalis Prog Neuropsychopharmacol Biol Psychiatry 2009 33(8):1329-35. [Google Scholar]
[60]. Allen LS, Gorski RA, Sex difference in the bed nucleus of the striaterminalis of the human brain J Comp Neurol 1990 302(4):697-706. [Google Scholar]
[61]. DeLorey TM, Sahbaie P, Hashemi E, Li WW, Salehi A, Clark DJ, Somatosensory and sensorimotor consequences associated with the heterozygous disruption of the autism candidate gene, Gabrb3 Behav Brain Res 2011 216(1):36-45. [Google Scholar]
[62]. Gur RC, Turetsky BI, Matsui M, Yan M, Bilker W, Hughett P, Sex differences in brain gray and white matter in healthy young adults: correlations with cognitive performance J Neurosci 1999 19(10):4065-72. [Google Scholar]
[63]. Goldstein JM, Seidman LJ, Horton NJ, Makris N, Kennedy DN, Caviness VS, Normal sexual dimorphism of the adult human brain assessed by in vivo magnetic resonance imaging Cereb Cortex 2001 11(6):490-97. [Google Scholar]
[64]. Hardan AY, Muddasani S, Vemulapalli M, Keshavan MS, Minshew NJ, An MRI study of increased cortical thickness in autism Am J Psychiatry 2006 163(7):1290-92. [Google Scholar]
[65]. Auzias G, Viellard M, Takerkart S, Villeneuve N, Poinso F, D Da Fonséca, Atypical sulcal anatomy in young children with autism spectrum disorder Neuroimage Clin 2014 4:593-603. [Google Scholar]
[66]. Murphy DG, DeCarli C, Mclntosh AR, Daly E, Mentis MJ, Pietrini P, Sex differences in human brain morphometry and metabolism: an in vivo quantitative magnetic resonance imaging and positron emission tomography study on the effect of aging Arch Gen Psychiatry 1996 53(7):585-94. [Google Scholar]
[67]. Hardan AY, Girgis RR, Adams J, Gilbert AR, Keshavan MS, Minshew NJ, Abnormal brain size effect on the thalamus in autism Psychiatry Res 2006 147(2-3):145-51. [Google Scholar]
[68]. Swaab DF, Fliers E, A sexually dimorphic nucleus in the human brain Science 1985 228(4703):1112-15. [Google Scholar]
[69]. Kurth F, Narr KL, Woods RP, J O’Neill, Alger JR, Caplan R, Diminished gray matter within the hypothalamus in autism disorder: a potential link to hormonal effects Biol Psychiatry 2011 70(3):278-82. [Google Scholar]
[70]. Caviness VS, Kennedy DN, Richelme C, Rademacher JF, Filipek PA, The human brain age 7–11 years: a volumetric analysis based on magnetic resonance images Cereb Cortex 1996 6(5):726-36. [Google Scholar]
[71]. Meaney MJ, McEwen BS, Testosterone implants into the amygdala during the neonatal period masculinize the social play of juvenile female rats Brain Res 1986 398(2):324-28. [Google Scholar]
[72]. Schumann CM, Hamstra J, BL Goodlin-Jones, Lotspeich LJ, Buonocore H, MH , The amygdala is enlarged in children but not adolescents with autism; the hippocampus is enlarged at all ages J Neurosci 2004 24(28):6392-401. [Google Scholar]
[73]. Filipek PA, Richelme C, Kennedy DN, Caviness VS, The young adult human brain: an MRI-based morphometric analysis Cereb Cortex 1994 4(4):344-60. [Google Scholar]
[74]. Sears LL, Vest C, Mohamed S, Bailey J, Ranson BJ, Piven J, An MRI study [74]of the basal ganglia in autism Prog Neuropsychopharmacol Biol Psychiatry 1999 23(4):613-24. [Google Scholar]
[75]. Swaab DF, Zhou JN, Ehlhart T, Hofman MA, Development of vasoactive intestinal polypeptide neurons in the human suprachiasmatic nucleus in relation to birth and sex Brain Res Dev Brain Res 1994 79(2):249-59. [Google Scholar]
[76]. Heath RA, The Praeger handbook of transsexuality: Changing gender to match mindset 2006 Greenwood Publishing Group [Google Scholar]
[77]. Reynolds AM, Malow BA, Sleep and autism spectrum disorders Pediatr Clin North Am 2011 58(3):685-98. [Google Scholar]
[78]. Devnani PA, Hegde AU, Autism and sleep disorders J Pediatr Neurosci 2015 10(4):304-07. [Google Scholar]
[79]. Cohen S, Conduit R, Lockley SW, Rajaratnam SM, Cornish KM, The relationship between sleep and behaviour in Autism Spectrum Disorder (ASD): a review J Neurodev Disord 2014 6(1):44 [Google Scholar]
[80]. Tani P, Lindberg N, T Nieminen-von Wendt, L Von Wendt, Alanko L, Appelberg B, Insomnia is a frequent finding in adults with Asperger syndrome BMC Psychiatry 2003 3(1):12 [Google Scholar]
[81]. Paavonen EJ, Vehkalahti K, Vanhala R, L von Wendt, T Nieminen-von Wendt, Aronen ET, Sleep in children with Asperger syndrome J Autism Dev Disord 2008 38(1):41-51. [Google Scholar]
[82]. NM Øane, Bjorvatn B, Sleep disturbances in adolescents and young adults with autism and Asperger syndrome Autism 2005 9(1):83-94. [Google Scholar]
[83]. Neufang S, Specht K, Hausmann M, O Güntürkün, B Herpertz-Dahlmann, Fink GR, Sex differences and the impact of steroid hormones on the developing human brain Cereb Cortex 2009 19(1):464-73. [Google Scholar]
[84]. A Fernández Guasti, Kruijver FP, Fodor M, Swaab DF, Sex differences in the distribution of androgen receptors in the human hypothalamus J Comp Neurol 2000 425(3):422-35. [Google Scholar]
[85]. Kruijver FPM, Balesar R, Espila AM, Unmehopa UA, Swaab DF, Oestrogen receptor-alpha distribution in the human hypothalamus in relation to sex and endocrine status J Comp Neurol 2002 454(2):115-39. [Google Scholar]
[86]. Keller F, Ruta L, The male prevalence in autism spectrum disorders: hypotheses on its neurobiological basis In the Neurochemical Basis of Autism 2010 Boston, MASpringer:13-28. [Google Scholar]
[87]. Mellon SH, Griffin LD, Compagnone NA, Biosynthesis and action of neurosteroids Brain Res Rev 2001 37(1-3):03-12. [Google Scholar]
[88]. Tsai M, BW O’Malley, Molecular mechanisms of action of steroid/thyroid receptor superfamily members Annu Rev Biochem 1994 63(1):451-86. [Google Scholar]
[89]. Sanchez AM, Flamini MI, Polak K, Palla G, Spina S, Mannella P, Actin cytoskeleton remodeling by sex steroids in neurones J Neuroendocrinol 2012 24(1):195-201. [Google Scholar]
[90]. Jahagirdar V, Wagner CK, Ontogeny of progesterone receptor expression in the subplate of fetal and neonatal rat cortex Cereb Cortex 2010 20(5):1046-52. [Google Scholar]
[91]. Ishrat T, Sayeed I, Atif F, Hua F, Stein DG, Progesterone and allopregnanolone attenuate blood–brain barrier dysfunction following permanent focal ischemia by regulating the expression of matrix metalloproteinases Exp Neurol 2010 226(1):183-90. [Google Scholar]
[92]. Brun CC, Nicolson R, N Leporé, Chou YY, Vidal CN, DeVito TJ, Mapping brain abnormalities in boys with autism Hum Brain Mapp 2009 30(12):3887-900. [Google Scholar]
[93]. Zikopoulos B, Barbas H, Changes in prefrontal axons may disrupt the network in autism J Neurosci 2010 30(44):14595-609. [Google Scholar]
[94]. Carmody DP, Lewis M, Regional white matter development in children with autism spectrum disorders Dev Psychobiol 2010 52(8):755-63. [Google Scholar]
[95]. Shukla DK, Keehn B, RA Müller, Tract specific analyses of diffusion tensor imaging show widespread white matter compromise in autism spectrum disorder J Child Psychol Psychiatry 2011 52(3):286-95. [Google Scholar]
[96]. Steinman G, Predicting autism at birth Med Hypotheses 2013 81(1):21-25. [Google Scholar]
[97]. Baulieu EE, Schumacher M, Progesterone as a neuroactiveneurosteroid, with special reference to the effect of progesterone on myelination Steroids 2000 65(10-11):605-12. [Google Scholar]
[98]. LM Garcia Segura, Melcangi RC, Steroids and glial cell function Glia 2006 54(6):485-98. [Google Scholar]
[99]. Wagner CK, Nakayama AY, GJ De Vries, Potential role of maternal progesterone in the sexual differentiation of the brain Endocrinology 1998 139(8):3658-61. [Google Scholar]
[100]. Gil M, Bhatt R, Picotte KB, Hull EM, Sexual experience increases oxytocin receptor gene expression and protein in the medial preoptic area of the male rat Psychoneuroendocrinology 2013 38(9):1688-97. [Google Scholar]
[101]. Barha CK, Ishrat T, Epp JR, Galea LA, Stein DG, Progesterone treatment normalizes the levels of cell proliferation and cell death in the dentate gyrus of the hippocampus after traumatic brain injury Exp Neurol 2011 231(1):72-81. [Google Scholar]
[102]. Woolley CS, McEwen BS, Roles of oestradiol and progesterone in regulation of hippocampal dendritic spine density during the estrous cycle in the rat J Comp Neurol 1993 336(2):293-306. [Google Scholar]
[103]. McEwen BS, Woolley CS, Oestradiol and progesterone regulate neuronal structure and synaptic connectivity in adult as well as developing brain Exp Gerontol 1994 29(3-4):431-36. [Google Scholar]
[104]. Sakamoto H, Ukena K, Tsutsui K, Effects of progesterone synthesized de novo in the developing Purkinje cell on its dendritic growth and synaptogenesis J Neurosci 2001 21(16):6221-32. [Google Scholar]
[105]. Romeo RD, Waters EM, Mcewen BS, Steroid-induced hippocampal synaptic plasticity: sex differences and similarities Neuron Glia Biol 2004 1(3):219-29. [Google Scholar]
[106]. SI Sá, Lukoyanova E, Madeira MD, Effects of oestrogens and progesterone on the synaptic organization of the hypothalamic ventromedial nucleus Neuroscience 2009 162(2):307-16. [Google Scholar]
[107]. Baudry M, Bi X, Aguirre C, Progesterone–oestrogen interactions in synaptic plasticity and neuroprotection Neuroscience 2013 239:280-94. [Google Scholar]
[108]. Wegiel J, Kuchna I, Nowicki K, Imaki H, Wegiel J, Marchi J, The neuropathology of autism: defects of neurogenesis and neuronal migration, and dysplastic changes Acta Neuropathol 2010 119(6):755-70. [Google Scholar]
[109]. Wagner CK, Progesterone receptors and neural development: a gap between bench and bedside? Endocrinology 2008 149(6):2743-49. [Google Scholar]
[110]. Mueller BR, Bale TL, Sex-specific programming of offspring emotionality after stress early in pregnancy J Neurosci 2008 28(36):9055-65. [Google Scholar]
[111]. Sarachana T, Xu M, Wu RC, Hu VW, Sex hormones in autism: androgens and oestrogens differentially and reciprocally regulate RORA, a novel candidate gene for autism PLoS One 2011 6(2):e17116 [Google Scholar]
[112]. Carter CS, Sex differences in oxytocin and vasopressin: implications for autism spectrum disorders? Behav Brain Res 2007 176(1):170-86. [Google Scholar]
[113]. TL O’Donohue, Charlton CG, Miller RL, Boden G, Jacobowitz DM, Identification, characterization, and distribution of secretin immunoreactivity in rat and pig brain Proc Natl Acad Sci 1981 78(8):5221-24. [Google Scholar]
[114]. Charlton CG, Miller RL, Crawley JN, Handelmann GE, TL O’Donohue, Secretin modulation of behavioural and physiological functions in the rat Peptides 1983 4(5):739-42. [Google Scholar]
[115]. Welch MG, Keune JD, TB Welch-Horan, Anwar N, Anwar M, Ruggiero DA, Secretin activates visceral brain regions in the rat including areas abnormal in autism Cell Mol Neurobiol 2003 23(4-5):817-37. [Google Scholar]
[116]. Welch MG, Keune JD, TB Welch-Horan, Anwar N, Anwar M, Ludwig RJ, Secretin: hypothalamic distribution and hypothesized neuroregulatory role in autism Cell Mol Neurobiol 2004 24(2):219-41. [Google Scholar]
[117]. Williams K, Wray JA, Wheeler DM, Intravenous secretin for autism spectrum disorders (ASD) Cochrane Database of Systematic Reviews 2012 (4):CD003495DOI: 10.1002/14651858.CD003495.pub3 [Google Scholar]