Nanotechnology involves the manipulation and application of engineered particles with at least one dimension, which is less than 100 nm [1]. The nanomaterial physicochemical properties caused by their small size, shape, aggregation, chemical composition, surface structure and solubility [2]. The small size of SNPs has increased their uptake and interaction with biological tissues [3]. SNPs are widely used in a variety of biomedical products as an antimicrobial additive [4]. SNPs are used for treatment of diseases that need for maintenance of circulating drug concentrations or targeting of specific cells or organs [5]. Nanoparticles can cause diseases such as asthma, emphysema, bronchitis, lung cancer, arteriosclerosis, arrhythmia, heart disease, Crohn’s disease, liver and kidney damage [6]. Recent in-vitro studies have shown that SNPs can induce toxicity in cells derived from a variety of organs [4]. The studies showed that SNPs had the cytotoxicity in rat neuroendocrine cells (PC-12) [7], rat alveolar macrophages [8], mouse fibroblast (NIH3T3) [9], human skin carcinoma (A431) [10], and human acute monocytic leukemia cell line (THP-1) [11], Human Epidermal Keratinocytes (HEKs) [12] mouse spermatogonial stem cells (C18-4) [13], human hepatoma cell line (HL-7702) [14].
Nanoparticles are degraded into ions. Those free ions can produce highly reactive hydroxyl radicals, which damage biological macromolecules such as DNA, proteins, and lipids [15], producing oxidative stress to the body, thereby inducing or enhancing inflammation [16] whereas, nanoparticles can enter into the body through ingestion, inhalation, or absorption through skin contact [17]. In addition, the use of SNPs in cosmetics and textiles has increased human skin penetration of SNPs [4].
It is necessary to examine the possible dermal toxicity of SNPs. In prior studies, we have observed subacute dermal toxicity of SNPs (10 μg/mL) on renal function parameters and hepatic necrosis biomarkers in serum [18] and SNPs (100 μg/mL) on serum chemical biomarkers of liver and TGF-beta1 level [19].
SNPs are the most widely used in consumer products. Thus, because of increasing potential for exposure of human to SNPs, there is an increasing concern about possible side effects of this nanoparticles. Despite the number of studies that have been done, little attention was paid to the dermal toxicity of these particles on human health in this study, two different SNPs doses were prepared, and repeated-dose toxicity in two different periods was evaluated in liver and kidney tissue of BALB/c mice after dermal exposure.
Materials and Methods
This experimental study was conducted in the Department of Biology, Faculty of Sciences, University of Shahrekord, Shahrekord, Iran, in August 2013 and September 2013. The experiment was started after obtaining the approval from Institutional Animal Ethical Committee, and the experiments were conducted according to the US National Institute of health guidelines.
Inclusion criteria was healthy mice, and exclusion criteria was survival of mice after anaesthesia.
Animal Selection
Male Balb/c mice of about six weeks with health status were purchased from animal house of Shahrekord Azad University, and were allowed to adapt to the animal room environment for one week before the onset of the study. The animals were maintained at a temperature of 22±3°C, relative humidity of 50%-70%, and 12 hour light/dark cycle. The mice were given ad libitum a standard diet and tap water.
SNPs solution (8000 ppm) was purchased from Nano-shop Co., Tehran, Iran. The size of the nanoparticle and purity were 40 nm and 98%, respectively.
Experimental Design
The animals were randomly divided into five groups of 10 (negative control, positive control, pseudo-control (sham) and two experimental (10 and 100 μg/mL SNPs) groups. Skin near the vertebral column of each animal was shaved (an area of 0.90 cm × 0.90 cm) for treatment. After general anaesthesia, the bandage surface was treated in the experimental groups, the positive control group, and in pseudo-control group respectively with a volume of 50 μL of the SNPs solution (10 and 100 μg/mL), AgNO3 solution (100 μg/mL), and distilled water was added to the sterile bandage of mice, then the bandages were fixed in the shaved surface, and kept separately for three and seven days, but the negative control group was without treatment and bandage. In this study, SNPs and AgNO3 solution were diluted with distilled water. At the end of exposure periods residual test bandage was removed using water.
Histopathological Analysis
The mice were killed under ether on three and seven days of the study, small pieces of mouse liver and kidney were fixed in 10% buffered formalin and dehydrated in a graded series of alcohol. Liver and kidney samples were sectioned at 5 μm, and then the samples were stained with Haematoxylin and Eosin (H&E) for histopathological studies. Animals in all experimental groups survived at termination of each period of the study and showed no clinical signs in all animals in all experimental groups.
Results
We found no mortality, abnormal behavior in SNPs and AgNO3-treated rats but minor histopathological changes were seen in the treatment group with SNPs.
Toxic Responses of Liver
In negative and pseudo control mice showed normal liver tissue with no specific injury [Table/Fig-1].
H&E stained liver sections of mice in 3 day of study (X40). a) Negative control and pseudo-control (Sham) with no tissue injury; b) Positive control with hyperemia in the central vein (arrow) inflammatory cells around the central vein and inflammatory foci (star); c) Mice treated with 10 μg/mL SNPs with hyperemia in the central vein (arrow) and inflammatory cells around the central vein; d) Mice treated with 100 μg/mL SNPs with hyperemia in the central vein (arrow), inflammatory cells around the central vein (star), inflammatory foci and the early stages of vacuolar degeneration of hepatocytes.
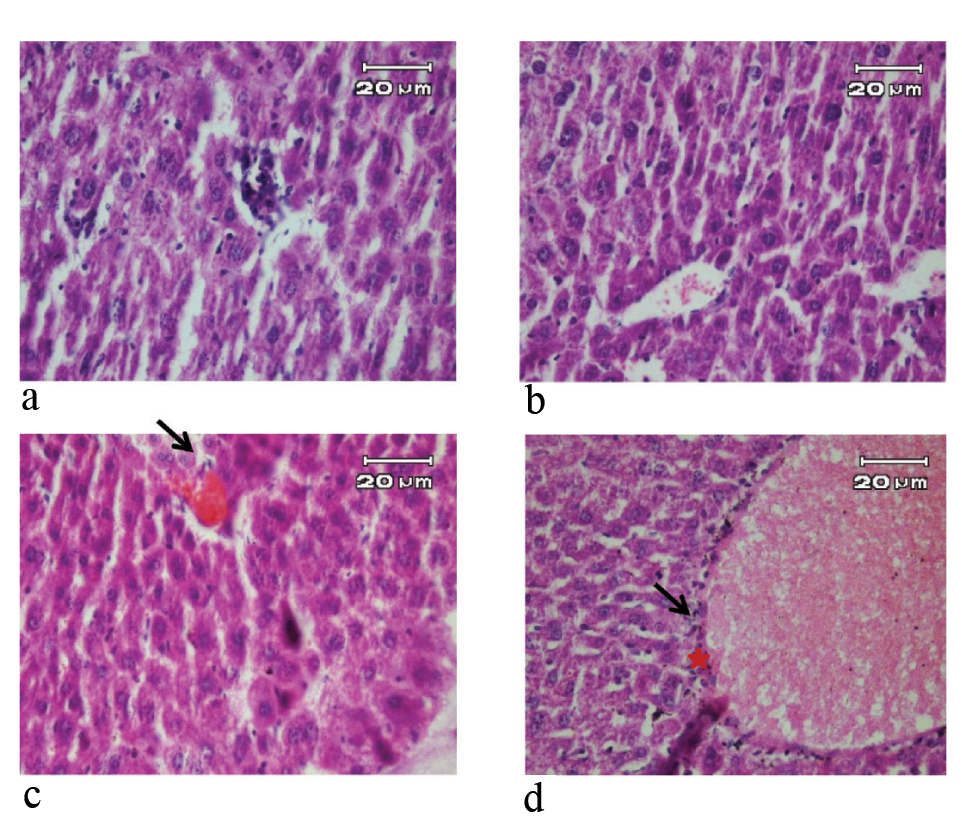
In contrast, the pathological changes of liver such as hyperemia in the central vein, inflammatory cells around the central vein and inflammatory foci were observed in positive control group during experiment periods. In the group treated with 10 μg/mL solution of SNPs, hyperemia in the central vein, dilatation of the central vein, inflammatory cells were observed during experiment periods. In the group treated with 100 μg/mL SNPs, hyperemia in the central vein, inflammatory cells around the central vein, inflammatory foci and the early stages of vacuolar degeneration of hepatocytes were observed on third day. On seventh day, in the group treated, in addition to the observations of the third day, it was observed cloudy swelling in hepatocytes [Table/Fig-2,3].
H&E stained liver sections of mice in 7 day of study (X40). a) Negative control and pseudo-control (Sham) with no tissue injury; b) Positive control with hyperemia in the central vein (arrow), inflammatory cells around the central vein and inflammatory foci (star); c) Mice treated with 10 μg/mL SNPs with dilatation of the central vein (arrow) and inflammatory cells around the central vein (star); d) Mice treated with 100 μg/mL SNPs with hyperemia in the central vein, inflammatory cells around the central vein, inflammatory foci and hepatocytes showed cloudy swelling (arrow).
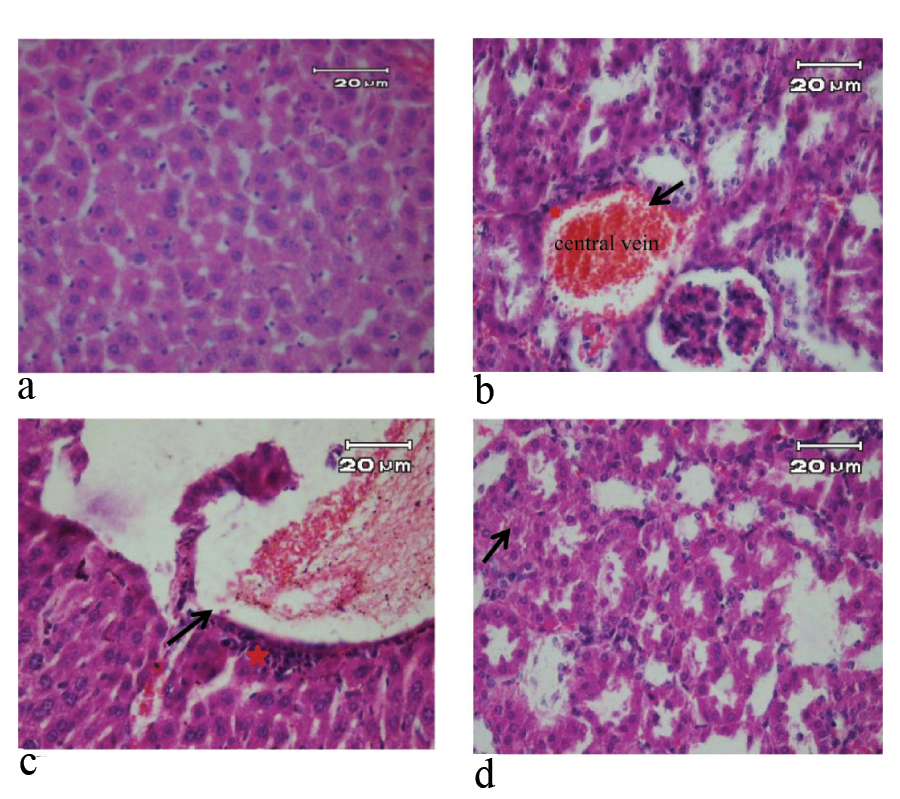
H&E stained kidney sections of mice in 3 day of study (X40). a) Negative control and pseudo-control (Sham) with no tissue injury; b) Positive control with glomerular hyperemia (arrow), inflammatory cells and vacuolar degeneration of renal cells (star); c) Mice treated with 10 μg/mL SNPs with glomerular hyperemia (arrow), vacuolar degeneration of renal cells, and vacuolar degeneration in distal renal tubular (star); d) Mice treated with 100 μg/mL SNPs with glomerular hyperemia (arrow), cloudy swelling in renal cells and vacuolar degeneration in distal renal tubular (star).
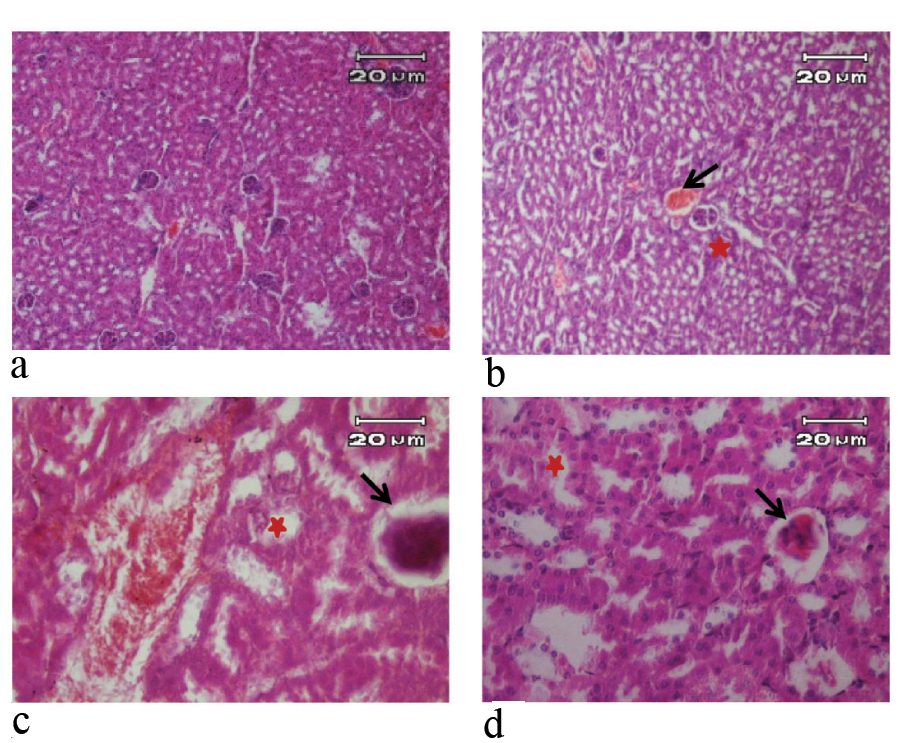
Toxic Responses of Kidney
Kidney tissue of negative and pseudo control mice were normal during experiment periods [Table/Fig-4]. In positive control group (100 μg/mL AgNO3 solution), glomerular hyperemia, vacuolar degeneration of renal cells and inflammatory cells were identified during experiment periods. In the group treated with 10 μg/mL solution of SNPs, glomerular hyperemia and vacuolar degeneration in renal cells and in distal renal tubular were observed on third day, and on seventh day, glomerular hyperemia, hyperemia in the renal medulla and vacuolar degeneration of renal cells were identified. In high-dose mice (100 μg/mL SNPs) was observed glomerular hyperemia, vacuolar degeneration in distal renal tubular and cloudy swelling in renal cells on third day. In this group, glomerular hyperemia, cloudy swelling in proximal and distal tubular and karyolysis in distal tubule were observed on seventh day [Table/Fig-4].
H&E stained kidney sections of mice in 7 day of study (X40). a) Negative control and pseudo-control (Sham) with no tissue injury; b) Positive control with glomerular hyperemia, inflammatory cells (arrow) and vacuolar degeneration of renal cells (star); c) Mice treated with 10 μg/mL SNPs with glomerular hyperemia, hyperemia in the renal medulla, vacuolar degeneration of renal cells (star); d) Mice treated with 100 μg/mL SNPs with glomerular hyperemia (arrow), cloudy swelling in proximal and distal tubular and the distal tubule showed karyolysis (star).
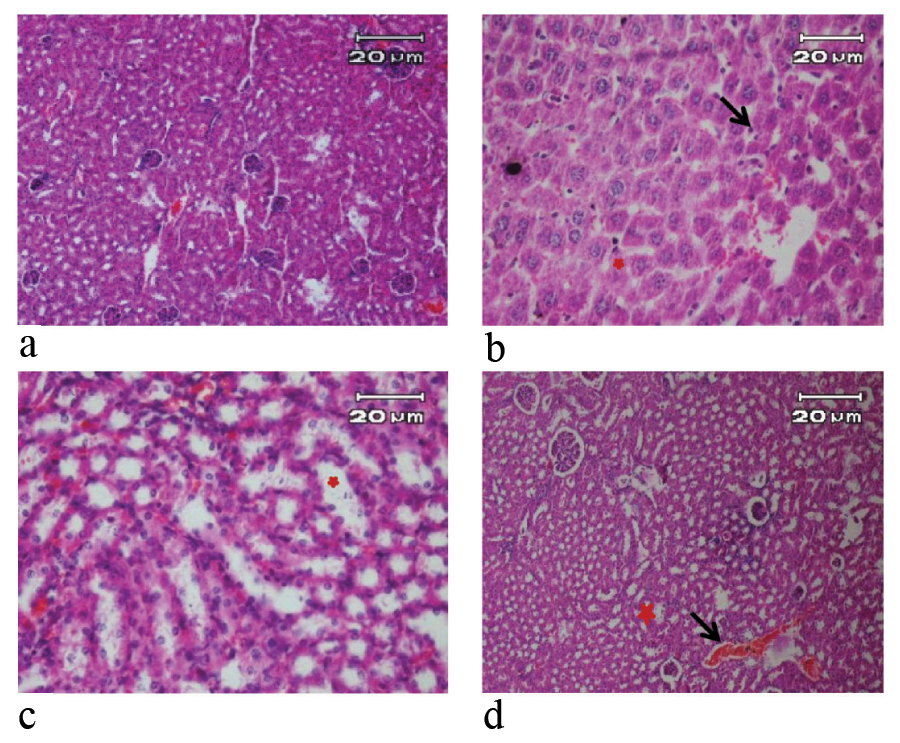
Discussion
The purpose of this study was to assess the histological response of liver and kidney in mice to skin absorption of SNPs. We observed that SNPs can induce liver and kidney damage in mice following skin absorption in a dose- dependent manner.
The study results showed that SNPs translocated from the blood system to some organs, such as liver and kidney, then caused hepatotoxicity or renal toxicity [20]. Nanoparticle toxicity is determined by inflammation resulting from oxidative stress [21]. Liver is one of the main targets of oxidative stress; because of slow clearance and storage of nano material scan produce free radicals, and thus prevalence of numerous phagocytic cells in this organ. Additionally, kidney is one of organs of high blood flow that can also be affected by nano materials [22].
A study have investigated the toxicity of different concentrations of SNPs (50 nm) in Bovine Retinal Endothelial Cells (BRECs) for 24 hour in the presence and absence of Vascular Endothelial Growth Factor (VEGF). They reported that silver nanoparticles can inhibit cell survival via PI3K/Akt dependent pathway in bovine retinal endothelial cells [23]. A study have reported the cytotoxicity and oxidative stress in human hepatoma HepG2 cells by SNPs (5–10 nm) at doses range 0.5 to 10 μg/mL [24]. Park EJ et al., studied the toxicity of different concentrations of SNPs (42 nm) in mice by oral administration for 28 days. They reported that Aspartate Aminotransferase (AST), Alanine Aminotransferase (ALT) and Alkaline Phosphatase (ALP) were significantly increased in only the group treated with the highest dosage (1 mg/kg SNPs), that this changes are the signs of hepatotoxicity. Blood Urea Nitrogen (BUN) and creatinine were not increased, but inflammatory responses were observed in kidney, it seemed that SNPs impaired the filtration capacity of kidney [25]. The researchers found that silver was distributed to all examined organs with the maximum amount detected in the liver and spleen for both the silver nanoparticles (20 nm non-coated, or 15 nm PVP- coated SNPs, 90 mg/kg of bw) and AgNO3 (9 mg/kg of body weight) treatments after 28 days of oral exposure in rats. They supposed that ingested silver is more bioavailable in the ionic form than the particulate form, but found that the general effects of exposure to SNPs appeared to be similar to the effects of exposure to silver nitrate. Interestingly, using single particle inductive coupled plasma mass spectroscopy (SP-ICP_MS), they found SNPs in rats of AgNO3 group and proposed that SNPs are composed of silver ions in vivo and they are probably formed from silver salts [26]. We have seen similar changes in positive control (AgNO3), and SNPs groups.
In a previous study, the distribution and accumulation of SNPs (50-100 nm) and silver micro particles (2-20 μm) were evaluated in rats following subcutaneous injection. Silver was demonstrated in the blood and some organs, such as liver and kidney that these organs had higher levels of silver than other organs. Translocation of SNPs was higher from silver micro particles [20]. Kazem Koohi M et al., found the pathological changes in the liver of rabbits by dermal exposure of SNPs [27]. Korani M et al., reported that nanosilver (< 100 nm) can cause histopatholgical abnormalities to skin, liver, and spleen of guinea pigs in a dose and time-dependent manner following dermal absorption of nanosilver [28]. They also reported the histopatholgical abnormalities in kidney, heart and bone of guinea pigs in a similar study [29]. These studies have similar results to the present study, but in other model animal. We observed hepatotoxicity and renal toxicity after skin absorption of SNPs (40 nm) in mice. Many studies have examined SNPs toxicity after oral administration [30], and inhalation [31]. Loghman A et al., observed the dose-dependent lesions in broiler chicken liver following 42 days of oral SNPs administration. They reported cell swelling and hyperemia at low-dose (4 ppm), hyperemia, dilated central vein and severe fatty change at more doses (8 and 12 ppm) and an increase in connective tissue and focal necrosis of hepatocytes at the highest dose (12 ppm) [32]. Kim YS et al., evaluated the toxicity of different doses of SNPs in rats after oral administration for 90-days. The results showed the histopathological abnormalities in different organs such as kidneys, spleen, heart, liver and etc., [30]. SNPs release silver ions (Ag+) in the aqueous state [33]. It is reported similar biological response for both SNPs and silver ions by some other investigators [12]. Kawata K et al., showed that both nano sized particle of silver and silver ions help for the toxic effects of SNPs [34]. However, some studies indicate that SNPs caused the toxicity independent of silver ions [33]. SNPs caused cellular toxicity and oxidative stress. In contrast, the silver ions induced the inflammatory response, but they led to a lower overall stress response in compared to SNPs [4]. Our prior studies showed that dermal absorption of SNPs (40 nm) with concentration of 10 μg/mL [18] and 100 μg/mL [19] have inhibition effects on the production of anti-inflammatory cytokine (TGF-β1). This change may lead to SNPs negative effects on liver and other sensitive organs such as kidneys where inflammatory reactions occur [19], also a significant increase in serum levels of renal function parameters such as blood urea nitrogen and hepatic necrosis biomarkers in serum such as ALT and Aspartate Aminotransferase (AST) in mice of exposure to 10 μg/mL SNP was observed [18]. In present study, we reported SNPs toxicity on the liver and kidney of mice at various concentrations (10 and 100 μg/mL) in a dose-dependent manner. According to the demonstrated results, we did not show clear histopathological changes between the various experimental periods, but our finding observed minimal histopathological changes between the various doses. So that, high-dose of SNPs (100 μg/mL) could cause the early stages of vacuolar degeneration of hepatocytes. In Korani N study has also been reported that the doses >100 could cause inflammation, limited pale destruction and hepatocytes degeneration in guinea pig liver after dermal absorption of SNPs [28]. In addition, in our study, in the mouse renal treated with high-dose of SNPs (100 μg/mL) was observed the cloudy swelling in proximal and distal tubular and karyolysis in the distal tubule.
Limitation
The limitations of the study were shaving area and time course of study.
Conclusion
In the present study, the toxicity potentials of SNPs were determined on the target organs including liver and kidney. We observed no mortality in mice of the treatment groups, but minor dose-dependent histopathological changes were shown in the treatment groups with SNPs. Considering to the present findings, SNPs (40 nm) can induce hepatotoxicity and nephrotoxicity following skin absorption in a dose-dependent manner. Therefore, it is necessary to measure tissue levels of SNPs in all treatment mice. It is also recommended for further research with various doses for different periods of time following various routes of administration of SNPs.
Financial support: This work was funded by University of Shahrekord, Shahrekord, Iran.