Potency of Injectable Hydroxyapatite Chitosan Scaffold for Bone Regeneration
Endang Winiati Bachtiar1, Dewi Fatma Suniarti2, Nadia Desti Fadhilah3, Rahmi Ulfiana4, Basril Abbas5
1 Faculty of Dentistry, Department of Oral Biology and Oral Science Research Center, Universitas Indonesia, Indonesia.
2 Faculty of Dentistry, Department of Oral Biology and Oral Science Research Center, Universitas Indonesia, Indonesia.
3 Faculty of Dentistry, Department of Oral Biology and Oral Science Research Center, Universitas Indonesia, Indonesia.
4 Faculty of Dentistry, Department of Oral Biology and Oral Science Research Center, Universitas Indonesia, Indonesia.
5Tissue Bank, Patir-Batan, Indonesia.
NAME, ADDRESS, E-MAIL ID OF THE CORRESPONDING AUTHOR: Dr. Endang Winiati Bachtiar, Faculty of Dentistry, Department of Oral Biology and Oral Science Research Center, Universitas Indonesia, Salemba Raya 4, Jakarta-10430, Indonesia.
E-mail: endang04@ui.ac.id/endangwiniati08@yahoo.com
Introduction
Several methods are used to enhance bone regeneration and new bone formation. There is a significant clinical need to develop alternatives to autografts and allografts for bone grafting procedures. Biopolymer chitosan combined with hydroxyapatite have been investigated as bone grafts substitute in the form of injectable, porous and biodegradable structure.
Aim
To evaluate the effect of Injectable Hydroxyapatite Chitosan (IHAC) Scaffold for rabbit femoral condyle bone regeneration by assessing its histological view.
Materials and Methods
The experiment was conducted on New Zealand White Rabbits. IHAC scaffold was transplanted into the femoral defect of a treated rabbit, while the control rabbit’s defect was left empty. Bone regeneration was analysed histologically using Modified Salkeld Histological Scoring, four weeks postoperatively.
Results
Cortical bone fusion occurred better in the treated rabbit compared to control rabbit. Active osteoblasts were found in the periphery of the mature bone. Cortical bone had undergone complete maturation. Control rabbit still showed the presence of haemorrhagic area and fibrocartilage that indicated early phase of bone regeneration.
Conclusion
IHAC scaffold is effective for treatment of bone defect by guiding the host response to regenerate the bone tissue.
Introduction
Annually, more than 2.2 million bone grafting procedures are performed worldwide in order to make sure the adequate bone healing in many skeletal defects [1]. Currently, an autograft is the golden standard for treatment of bone defect [2]. However, there are several downsides associated with an autograft. In the clinical application, a portion of bone is taken from a separate donor site from the patient’s body. Hence, the surgical procedure is conducted twice. This treatment is also related to new fracture, donor site pain, new nerve damage, infection and bleeding [3,4]. For these reasons, allograft has been investigated and approved but is also associated with a high potential risk of infection, disease transmission and immunologic reaction. Therefore, tissue engineering can become a treatment of choice with a promising approach for bone regeneration, particularly for a large size bone defect regeneration [3,4]. There are three main factors involved for the same: stem cell, scaffold and growth factor. Scaffold plays an important role as a structural support. An ideal scaffold should be biocompatible, biodegradable, have high surface ratio that could facilitate the growth of the cells to desired tissue [5] and allow sufficient transportation of gases, nutrition and any other important factor [6]. Recently, a three-dimensional injectable scaffold made from Hydroxyapatite (HA) and chitosan named IHAC has been studied extensively by National Atomic Energy Agency of Indonesia in collaboration with Oral Science Research Center, Faculty of Dentistry, University of Indonesia [6]. Hydroxyapatite is a major inorganic component in human hard tissue including bone and teeth [6]. Nowadays, researchers have extensively fabricated hydroxyapatite using biomimetic strategies and is used in many types of implant regeneration and bone regeneration [7,8]. Fabricated hydroxyapatite shows an improved clinical outcome due to its similarity in the chemical composition to natural bone including high osteoconductivity and biocompatibility [9]. Natural bone is a composite consisting of 60-70% hydroxyapatite as an inorganic component and other organic component. This organic component plays a vital role in maintenance of bone structure [10]. Absence of this organic component causes migration of hydroxyapatite particle from implanted site to the healthy tissue [10]. Due to this condition, combining hydroxyapatite with biopolymer was investigated to find the best material that can maintain good properties of hydroxyapatite. Chitosan is a chitin derivate that is obtained by chitin deacetylation [11]. It is the most abundant polysaccharide after cellulose that can be degraded by glucosaminidase and chitosanases. In addition, chitosan has been proven to have an increased biocompatibility property, minimal immune reaction and antimicrobial activity [11]. Another study reported that Deproteinized Bovine Bone Mineral (DBBM) is also capable of regenerating new bone formation [12]. Additionally, Hydroxypropyl Methylcellulose (HPMC) is required in order to create injectable scaffold [13,14]. In the clinical practice, usage of injectable scaffold provides several advantages over the traditional (liquid-powder form) as it minimizes risk of infection, cost of treatment, scar formation and patient discomfort [3]. Also, it can reach the defect site having limited access. Previous study on IHAC conducted by Bachtiar EW et al., showed minimal toxicity and improved cell proliferation, in vitro [11]. Therefore, an evaluation on the potency of injectable hydroxyapatite chitosan scaffold in vivo, is important as the development of this material is important to reduce the dependency to imported goods and promote more affordable medical treatment for local people in Indonesia. We have hypothesized that IHAC will accelerate bone regeneration in the area of defect.
Materials and Methods
This experimental research was conducted from January 2016 to August 2016 in Oral Biology Laboratory, Faculty of Dentistry, and Histology Laboratory and Animal House, Faculty of Medicine, University of Indonesia, Indonesia. Prior to the research, ethical clearance was obtained from Animal Ethic Commission of Bogor Agricultural Institute, Indonesia (No. 05-2015IPB). In this experiment, we used two New Zealand adult rabbits (weight 3 kg). The rabbits were randomly assigned as control rabbit and treated rabbit. Rabbits were quarantined for two weeks preoperatively and received proper food and water throughout the project.
Surgical Procedure
General anesthesia containing a mixture of ketamine (1.5 mL) and xylazine (0.5 mL) was injected by an intramuscular injection. The femoral condyle area was shaved and rinsed with Povidone-Iodine [4]. Longitudinal incision was made in femoral condyle area followed by the elevation of soft tissue. A defect of 1 cm depth and 5 mm in diameter was made in the exposed bone, 2 cm length from the tip of femoral condyle using a bone bur. Defect of the control rabbit was left empty, while defect of treated rabbit received IHAC scaffold [Table/Fig-1].
Surgical procedure. a) Defect was made using bone bur; b) Defect of of 1cm depth and 5mm in diameter was made in rabbit’s femoral condyle; c) IHAC Scaffold placed in defect area.
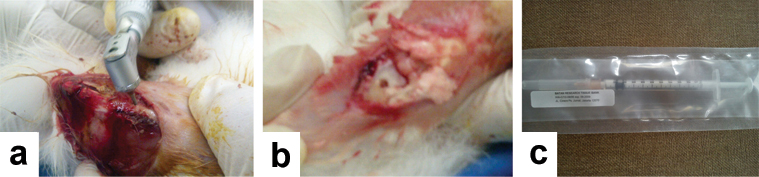
Histologic Processing
After four weeks, the rabbits were euthanized and defect site was removed. The time period of four weeks was taken because formation of hard callus (which marked the mature stage of bone) occured after four weeks.
Then, the adjacent tissue was removed from the sectioned bone. The bone was immersed in 10% formalin for 24 hours, followed by alcohol with concentration 70%, 80%, 95%, and 100% each for 24 hours. After dehydration and decalcification process, the bone was embedded in paraffin a block. Serial 5 μμm thick paraffin block was cut through the center of the femoral condyle defect, subsequently staining was performed with Haematoxylin and Eosin (H&E) for microscopic evaluation.
Histologic Analyses
The histological slides were examined using a binocular microscope (Olympus, China) at 40X and 100X magnification. The examination was carried out in Histology Department, Faculty of Medicine, University of Indonesia, by experts in Histology. The experts performed a descriptive histological evaluation based on modified Salkeld Histological Scoring so as to obtain the histological healing score for each rabbit [12]. The higher score shows better bone formation [Table/Fig-2].
Histological Scoring | Description |
---|
0 | No healing |
1 | Healing with fibrous union |
2 | Healing with fibro-cartilaginous or cartilage union |
3 | Bone healing with mineralized cartilage union |
4 | Mature bone healing |
Modified Salkeld Histological Scoring of bone healing [12].
Results
Bone regeneration was evaluated histologically after four weeks post scaffold transplantation into the femoral condyle defect [Table/Fig-3,4]. In control rabbit’s histological view, the concave area is the central of defect area. Dark-red zone indicated mineralized cortical bone (hard tissue), while the light-red zone indicates un mineralized bone and soft tissue. Histological evaluation in the defect area showed poor bone formation, indicated by the minimal formation of mineralized bone fragment in some areas [Table/Fig-3a]. Presence of haemorrhagic area, chondrocyte and fibroblast could be seen in the defect area of the control rabbit [Table/Fig-4]. These three components indicated that the bone formation was still in an early stage of bone healing. Based on Modified Salkeld Histological Scoring, the control rabbit score was 2; as the fibrocartilage is the dominant component found in this histological view [15]. Meanwhile, the histological view of treated rabbit showed an increased compact bone formation compared to control rabbit. This is indicated by the union of bone fragment into well formed cortical bone [Table/Fig-3b]. An increased number of osteocytes were found in this newly formed bone. Many active osteoblasts were found embedded along the peripheral of cortical bone [Table/Fig-4b]. Osteoblast was in a flat shape which means that the osteoblast actively differentiated into osteocyte [15]. The changes of the treated rabbit were significant, indicated by the presence of abundant osteoblasts and osteocytes and also a better union of cortical bone. Based on Modified Salkeld Histological Scoring, treated rabbit score was 4, as the osteogenesis already reached the mature stage.
Histological observation at four weeks postoperatively on control rabbit: (a) and treated rabbit; (b) in 40X magnification. Adipose tissue (At), Defect Area (Da), Bone fragment (Fb), Cortical bone (Cb).
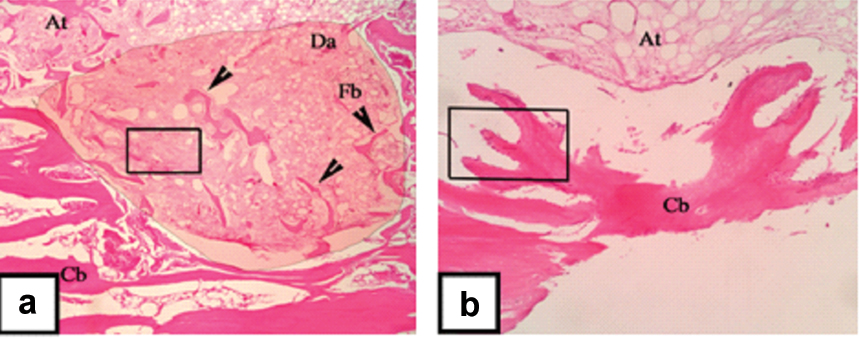
Histological observation at four weeks postoperatively on control rabbit: (a) and treated rabbit; (b) in 100X magnification. Haemorrhagic area (Hm, black arrowhead), Fibroblast (Fb, green arrowhead), Chondrocyte (Ch, blue arrowhead), Osteocyte (Os, red arrowhead), Osteoblast (Ob, black arrowhead).
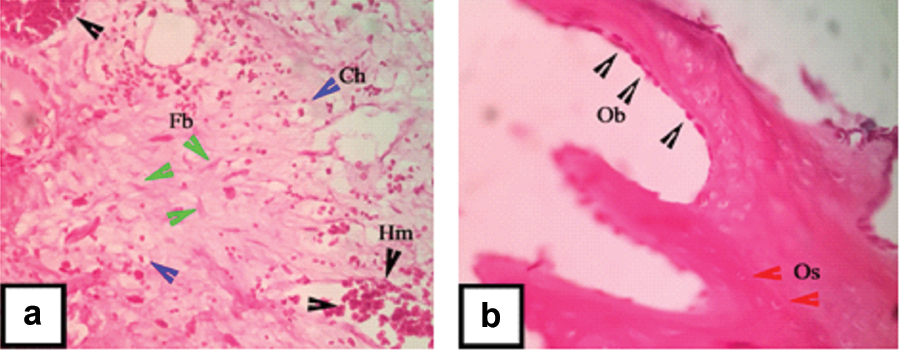
The result of histological evaluation in control and treated rabbit are shown in [Table/Fig-5]. A positive sign indicates the presence of bone-healing feature. A negative sign indicates the absence of them. Control rabbit had four positive and already reached the un-mineralized bone formation, but haemorrhagic area and inflammatory cells still can be found. It means there was an impairment in the bone healing process. On the other hand, treated rabbit already reached the highest stage of bone healing indicated by the presence of osteocyte, osteoblast and mineralized cortical bone.
Comparison of control rabbit and treated rabbit in bone healing and regeneration progress.
| Feature in bone healing | Control group | Treated group |
---|
Better bone formation | Hematoma | + | – |
Inflammatory cells | + | – |
Fibroacrtilage callus | + | – |
Unmineralized bone | + | + |
Mineralized bone | – | + |
Discussion
An ideal scaffold should possess the characteristics of biocompatibilty, should be biodegradable, have a high surface ratio that could facilitate the growth of cells to desired tissue and allow sufficient transport of gases, nutrition and any other important factors. Composite scaffold made from natural polymer and bone-like hydroxyapatite have been investigated intensively and show promising clinical outcome [11]. Hydroxyapatite and chitosan are good materials for bone regeneration due to their biocompatibility, osteo-conductivity and biodegradability [11]. In this paper, we have described the evaluation of IHAC scaffold transplantation in the femoral bone of New Zealand rabbit using Modified Salkeld Histological Scoring. By using this scoring system, the quality of bone regeneration can be evaluated. From the histological analysis, we observed a discrepancy in bone-healing progress between control and treated rabbit. The treated rabbit showed a progressive increase in bone regeneration indicating the union of mineralized cortical bone with the remodeling phase. On the other hand, the defect area in the control rabbit consisted of bone fragments and dominated by callus. These findings are similar in a research on Hydroxyapatite-Chitosan conducted by Chen Y et al., that showed complete healing of the bone defect in the rabbit receiving nano-hydroxyapatite/chitosan composite, while defect in control rabbit was still visible [3]. HA and chitosan are reported to enhance the process of osteoblast proliferation and differentiation [4]. Scanning Electron Microscope (SEM) evaluation of composite scaffold conducted by Kong L et al., showed many osteoblast cells adhered into the pores walls and distributed well [13]. The results showed that the combination of HA and chitosan increased the biocompatibility of scaffold [13]. Chitosan cationic feature plays a vital role in the interaction with some anion element such as Glycosaminoglycan (GAG) and proteoglycan. Glycosaminoglycan is known to be responsible in retaining and accumulating the cytokines and growth factor, so it will enhance the process of bone regeneration [13]. Research conducted by Liu H et al., showed other possible mechanisms of bone regeneration in HA-CS (Hydroxyapatite-Chitosan) transplanted defect. Hydroxyapatite/chitosan scaffold promotes bone regeneration by enhancing the adhesion, proliferation and activation of integrin-Bone Morphogenetic Protein (BMP) signaling pathway [14]. Further study needs to be conducted with larger sample size and analysing other biomarkers quantitatively. In the future, IHAC can be projected to be the solution for many skeletal problems as it is easy to use, affordable and accessible.
Conclusion
Injectable Hydroxyapatite Chitosan (IHAC) scaffold is capable of supporting the adhesion and differentiation of osteoblasts. This condition supported the bone regeneration in the treated rabbit, indicated by complete union of cortical bone and fast progression of bone regeneration. Therefore, IHAC has a potential in promoting and guiding bone regeneration in bone defect. Further research on the development of IHAC scaffold is required to create more promising hard tissue graft for bone tissue engineering.
[1]. Chen Y, Huang Z, Li X, Li S, Zhou Z, Zhang Y, In vitro biocompatibility and osteoblast differentiation of injectable chitosan/nano-hydroxyapatite/collagen scaffold J of Nanomaterials 2012 :401084 [Google Scholar]
[2]. Li Y, Chen S, Li L, Qin L, Wang X, Lai Y, Bone defect animal models for testing efficacy of bone substitute biomaterials Journal Of Orthopedic Translation 2012 3:95-104. [Google Scholar]
[3]. Chen Y, Li S, Li X, Zhang Y, Huang Z, Feng O, Noninvasive evaluation of injectable chitosan/nano- hydroxyapatite/collagen scaffold via ultrasound J of Nanomaterials 2012 :939821 [Google Scholar]
[4]. Liu J, Mao K, Liu Z, Wang X, Cui F, Guo W, Injectable biocomposites for bone healing in rabbit femoral condyle defects Plos One 2013 8(10):01-11. [Google Scholar]
[5]. Rodríguez-vázquez M, Vega-ruiz B, Ramos-zúñiga R, Saldaña-koppel DA, Quiñones-Olvera LF, Chitosan and its potential use as a scaffold for tissue engineering in regenerative medicine Biomed Research Int 2015 :821279 [Google Scholar]
[6]. Bachtiar E, Amir L, Suhardi P, Abas B, Scaffold degradation during bone tissue reconstruction in Macaca nemestrina mandible Interv Med Appl Sci 2016 8(2):77-78. [Google Scholar]
[7]. Tortora GJ, Wiley J, Roesch B, Principle of Anatomy and Physiology USAWiley:182-95. [Google Scholar]
[8]. Cengiz B, Gokce Y, Yildiz N, Aktas Z, Calimli A, Synthesis and characterization of hydroxyapatite nanoparticles Colloids Surfaces A: Physicochem Eng Asp 2008 322(1-3):29-33. [Google Scholar]
[9]. Tzaphlidou M, Bone architecture, collagen structure and calcium/phosphorus maps J Biol Phys 2008 3(4):39-49. [Google Scholar]
[10]. Feng X, Chemical and biochemical basis of cell-bone matrix interaction in health and disease Curr Chem Biol 2009 3(2):189-96. [Google Scholar]
[11]. Bachtiar EW, Bachtiar BM, Abas B, Harsas NA, Sadaqah NF, Aprilia R, Biocompatibility and osteoconductivity of injectable bone xenograft, hydroxyapatite, and hydroxyapatite- chitosan on osteoblast culture Dent J 2010 4(3):176-80. [Google Scholar]
[12]. Wahyudi M, Kamal AF, Siregar NC, Prasetyo M, Effect of extracorporeal irradiation on segmental bone autograft incorporation in Sprague-Dawley rats Med J Indones 2014 2(3):147-53. [Google Scholar]
[13]. Kong L, Gao Y, Cao W, Gong Y, Zhao N, Zhang X, Preparation and characterization of nano-hydroxyapatite/chitosan composite scaffolds J Biomed Mater Res-Part A 2005 7(5):275-82. [Google Scholar]
[14]. Liu H, Peng H, Wu Y, Zhang C, Cai Y, Xu G, The promotion of bone regeneration by nanofibrous hydroxyapatite/chitosan scaffolds by effects on integrin-BMP/Smad signaling pathway in BMSCs Biomaterials 2013 34(18):4404-17. [Google Scholar]
[15]. Gartner LP, Hiatt JL, Color Textbook of Histology 3th edPhiladelphiaElsevier Health Sciences [Google Scholar]