In this technologically savvy era, modern dentistry mainly aims to restore the patient to their normal life. It not only includes restoring health of the patients but also includes their speech and aesthetic demands. The science of missing teeth replacement is as ancient as 300 BC where Egyptians employed variety of methods to replace missing teeth. With the advent of dental implants, came a major breakthrough in dentistry for prosthetic replacement of teeth [1]. Implant dentistry is a beautiful art and science which can achieve normal function as well as amazing aesthetics irrespective of limitations such as atrophy, disease or injury of the stomatognathic system. As a result of continued research in diagnostic tools, implant designs, materials and techniques; implant dentistry has now been able to achieve predictable success as high as 95% for mandibular implants and 90% for maxillary implants [2].
Even with the advancements in material science and designs, implant failure is still a nightmare to both the practitioner as well the patient. Hence, it becomes very crucial to consider and apply strategies for prevention of implant failure [3]. The introduction and widespread use of dental implants for the oral rehabilitation of fully and partially edentulous patients has greatly broadened the scope of modern dental practice, creating additional treatment options in situations where functional rehabilitation was not satisfactory by conventional treatment options [2].
The success or failure of dental implants depends on various complex interwoven factors such as bone quantity, bone quality, surgical technique, implant designs and surfaces and host related factors etc. Zarb GA and Schmitt A highlighted the predominance of bone structure in choosing the most favourable treatment outcome [4]. Density and quantity of the available bone as well as location of the treatment site are crucial factors in treatment planning, selecting implant design, surgical approach and healing time [3]. Out of many classifications of bone density, the classification given by Misch CE is the one which is widely in use. Misch CE classified bone density in four types: D1, D2, D3 and D4 [5]. D1 type of bone is comprised of homogenous compact bone. D2 type of bone is a combination of dense to porous compact bone on the outside and coarse trabecular bone on the inside. D3 type of bone is composed of thinner porous compact bone and fine trabecular bone. D4 type of bone has very light density and little or no cortical crestal bone [5]. Higher failure rates have been reported for the regions with poor quality bone e.g., posterior maxilla or D4 type of bone [2,3,6-9]. To reduce the incidence of implant failure, alterations in implant design and implant surface characteristics have been suggested, particularly in areas with poor bone quality. This will provide better anchorage and more surface area to decrease the stresses in the softer bone type [2]. As different implant-thread designs are available, it is necessary to evaluate the effect of these macroscopic thread patterns on the success of dental implants. There are four basic thread patterns reported in the literature: square, V-shaped, buttress and reverse buttress [2]. But many of the newer implant systems are coming up with a variable thread design which is a combination of square and V-shaped thread design (Alpha bio implants, Nobel Active, Adin). Currently, there are very few studies available regarding the effects of the newer variable thread designs in different bone densities [10].
Hence, the aim of this study was to evaluate the stress distribution with two different implant thread designs within two different bone densities, using three dimensional finite element analyses. While the objectives of the study were to evaluate von Mises stress for buttress implant thread design and variable thread design in D2 and D4 type of bone. D2 and D4 bone densities were selected as these are the commonest of all to be seen in human jaws. Also, in a finite element analytical study, material properties are important which are almost similar for D1 and D2 type of bone and for D3 and D4 type of bone. The study also compared the stresses generated with the buttress and variable thread designs in D2 and D4 type of bone to conclude which implant thread design is better depending upon the bone density.
Materials and Methods
The study was conducted at HKES’s S.N Dental College, Gulbarga, Karnataka, India in 2012 to evaluate the pattern of stress distribution in bone and around the implants using finite element method. Ethical clearance was obtained for the study from the ethical clearance committee of the institute. Three dimensional finite element analyses is an advanced and universally accepted technique for the measurement and quantification of stresses on the dental implant and the surrounding bone [3,10-14].
Construction of the geometric model: Pro-engineer 3-0 software was used for the modelling. A three-dimensional finite element model of a mandibular section of bone and an implant with Porcelain Fused to Metal (PFM) crown covering the implant abutment was used in this study. Different sub-models such as bone, dental implant, implant abutment and PFM crown were designed separately; then these were assembled to form the final model. As this was a finite element analytical study, one assembled model of each of the four types was fabricated which was the sample size.
1. Modelling of bone: A bone block of 23x17x14 mm, representing the section of the mandible in the molar region was modelled with cortical and cancellous bone. Misch classified bone in four different types according to their density (D1-D4). In this study, we used two different bone densities D2 and D4. In the first model, cortical bone thickness was 2 mm surrounding the cancellous bone while in second model it was 1 mm surrounding the cancellous bone according to bone type D2 and D4 respectively [3].
2. Modelling of Implant and Abutment: A tapered threaded dental implant with dimensions of 4.2x12 mm was used. The present study used two macroscopic implant thread designs i.e., buttress and newer variable thread design. Buttress thread design is the one in which lower surface of the thread is perpendicular to the implant body while upper surface is at an angle. Variable thread design is combination of square and V-shaped thread design. The first type of implant model had a buttress thread design along the whole implant length in D2 and D4 bone types; while the other model had a variable thread design which comprised of square threads in the coronal half and V-shaped threads in the apical region in D2 and D4 bone. In both the designs, the thread arrangement was spiral. In all the models, implant thread pitch was 0.8 mm. A titanium abutment of 4.5 mm length and 4.2 mm diameter was designed over the implant.
3. Modelling of PFM Crown: Porcelain fused to metal crown resembling the mandibular first molar was modelled over the abutment. The crown dimensions were derived from the average dimensions of the mandibular first molar [15]. To form the final model, four sub-models were assembled. Two sub-models had buttress thread designs, one each in D2 and D4 type of bone and 2 sub-models had variable thread design, one each in D2 and D4 type of bone [Table/Fig-1]. The final four models were:
Assembled implant model in D2 bone; b) Assembled implant model in D4 bone.
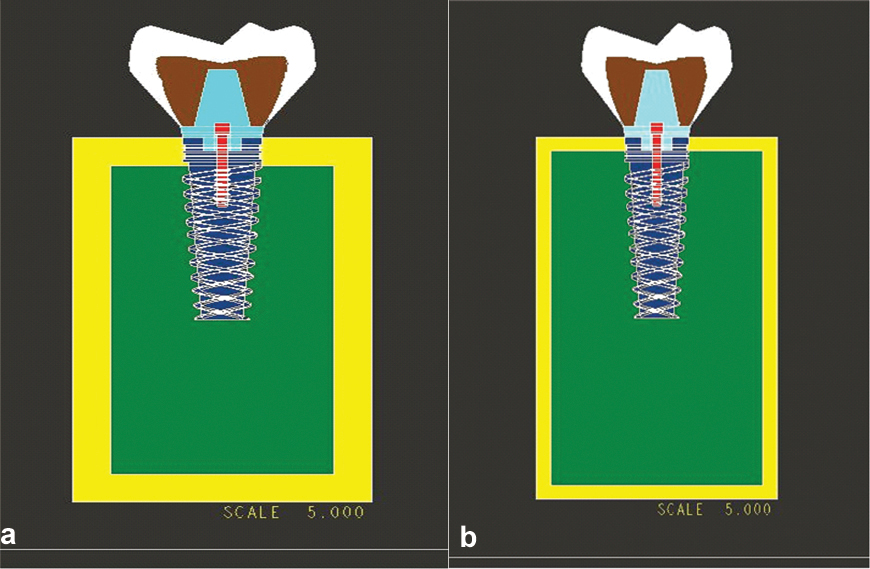
Model I a: Implant with buttress thread design in D2 bone
Model I b: Implant with buttress thread design in D4 bone
Model II a: Implant with variable thread design in D2 bone
Model II b: Implant with variable thread design in D4 bone
Preparation of Finite Element Mesh and Boundary conditions: After construction of the geometric models, each model was converted into a meshed model; by software (hyper mesh-11 software). Nodes and elements in the models are depicted in [Table/Fig-2]. All the vital tissues (cortical, cancellous bone) and implant with superstructure were presumed to be linearly elastic, homogenous and isotropic. The material properties for each component of the model such as Young’s modulus and Poisson’s ratio were determined according to literature survey and were applied to the final models [Table/Fig-3] [3]. For the boundary condition of the model, a supporting system was set up. The model was fixed at the base.
Nodes and elements in the models.
Model | Nodes | Elements |
---|
Model I a(Implant with buttress thread design in D2 bone) | 42452 | 215601 |
Model I b(Implant with buttress thread design in D4 bone) | 105274 | 71248 |
Model II a(Implant with variable thread design in D2 bone) | 52240 | 257783 |
Model II b(Implant with variable thread design in D4 bone) | 104602 | 70725 |
Material properties assigned to the model.
Material | Young’s Modulus (GPa) | Poisson’s Ratio (v) |
---|
Titanium dental implant and abutment | 110 | 0.35 |
Dense trabecular bone (for D1, D2, D3, D4 bone) | 1.37 | 0.3 |
Low-density trabecular bone (for D4 bone) | 1.10 | 0.3 |
Cortical bone | 13.7 | 0.3 |
Co-Cr alloy | 218 | 0.33 |
Feldspathic porcelain | 82.8 | 0.35 |
Application of different loads and Stress analysis: Static forces were applied. Vertical as well as oblique forces were also considered as the latter represents more realistic occlusal forces [3]. Vertical and oblique forces of 450 N each were applied at three locations i.e., central fossa (150 N), buccal cusp tip (150 N), distal marginal ridge (150 N) [Table/Fig-4]. Von Mises stress analysis was done. The stress analysis was performed on a personal computer of Dell Precision 420 Dual Pentium III 1 GHz (Dell, Austin, Tex) using software COSMOS/M (version 2.5; Structural Research and Analysis Corp, Santa Monica, Calif). Boundary conditions, loading, and mathematical model were prepared with the help of Pro/Engineer 2000i (Parametric Technology Corp). The outputs were transferred to a COSMOS/M program to show the stress values and distributions.
Results
The results of the von Mises stress analysis by Ansys software provided results in the form of von Mises stress fields with colour-coded bands. Each colour band represented a particular range of stress value, which is stated in Mega Pascal (MPa). Von Mises stress analysis, was done for the aforementioned four models under both vertical as well as oblique loading (450 N each) [Table/Fig-5]. Stress distribution patterns for the implant with variable thread design under vertical as well as oblique loading in D2 and D4 type of bone showed maximum stresses at the neck of the implant in cortical bone. In D2 type of bone, maximum stresses were 109.89 MPa and 257.46 MPa which were observed in the coronal cortical part of the bone corresponding to the neck of the implant under vertical and oblique loading respectively [Table/Fig-6].
Meshed model showing application of: a) Vertical loads; b) Oblique loads.
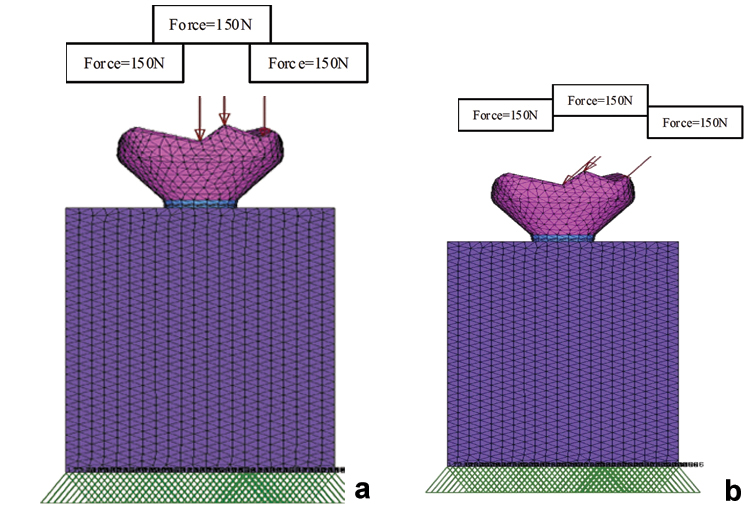
Von mises stress analysis for variable thread design and buttress thread design.
Component | Variable thread design | Buttress thread design |
---|
D2 type of bone | D4 type of bone | D2 type of bone | D4 type of bone |
---|
Vertical loading | Obliqueloading | Verticalloading | Obliqueloading | Verticalloading | Obliqueloading | Verticalloading | Obliqueloading |
---|
Stress within the bone(Maximum stressvalue in MPa) | 109.89 | 257.46 | 243.93 | 371.81 | 113.51 | 176.14 | 290.99 | 481.27 |
Variable thread design: Stress patterns generated within the bone at bone implant interface in D2 type of bone under: a) Vertical loading; b) Oblique loading.
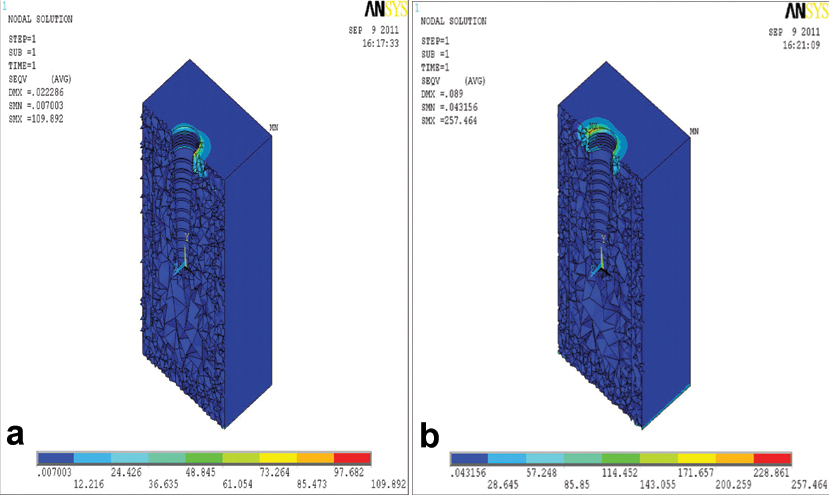
Similarly, von Mises stresses were 243.93 MPa and 371.81 MPa under vertical and oblique loading in D4 type of bone respectively [Table/Fig-7]. So, it was observed that the stresses were more in D4 type of bone than in D2 type of bone under vertical and oblique loading with variable thread design. As in the case of variable thread design, under both loading conditions maximum stress was seen at the neck of the implant in cortical bone, for the buttress thread design the same was observed. In D2 type of bone, stresses were 113.51 MPa and 176.14 MPa which were observed in the coronal part of the bone corresponding to the neck of the implant under vertical and oblique loading respectively [Table/Fig-8]. Similarly, von Mises stresses were 290.99 MPa and 481.27 MPa under vertical and oblique loading respectively in D4 type of bone in buttress thread design [Table/Fig-9].
Variable thread design: Stress patterns generated within the bone at bone implant interface in D4 type of bone under: a) Vertical loading; b) Oblique loading.
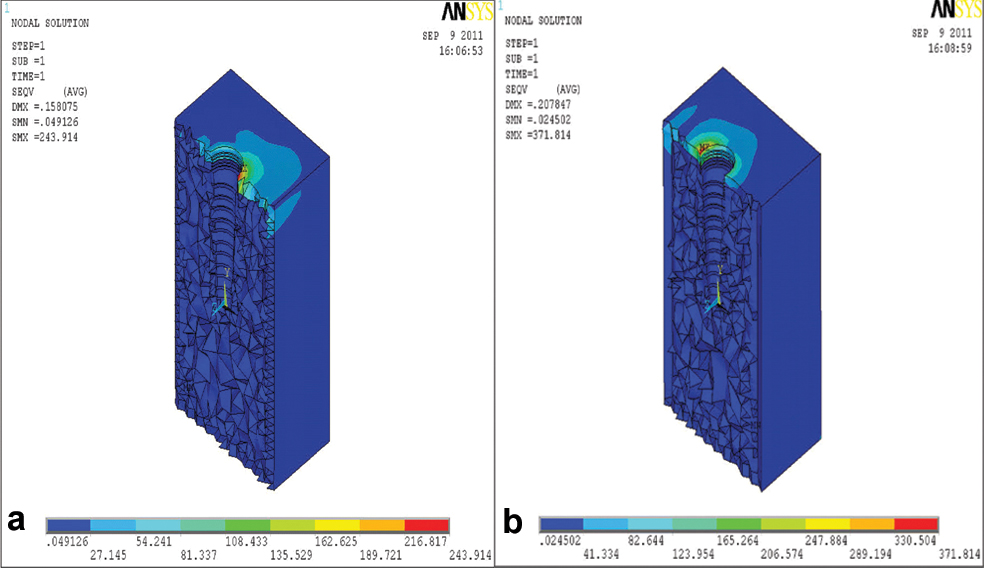
Buttress thread design: Stress patterns generated within the bone at bone implant interface in D2 type of bone under: a) Vertical loading; b) Oblique loading.
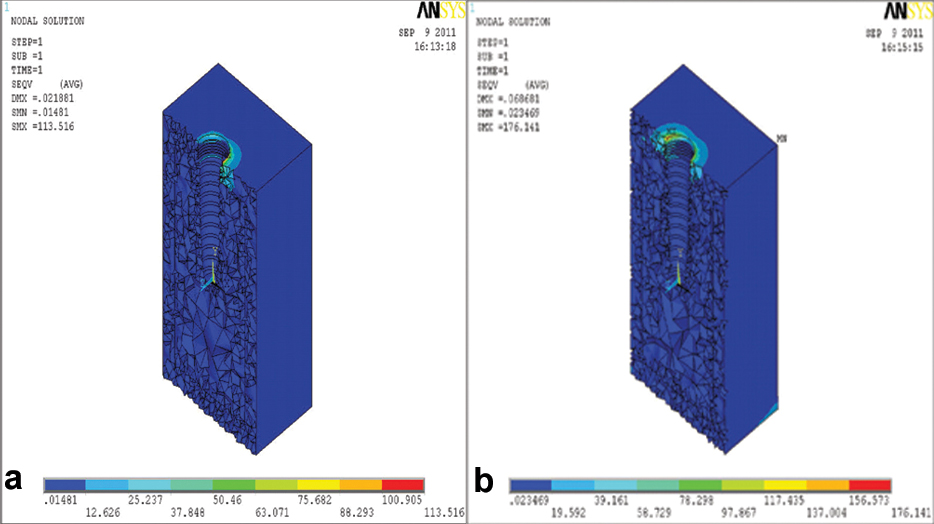
Buttress thread design: Stress patterns generated within the bone at bone implant interface in D4 type of bone under: a) Vertical loading; b) Oblique loading.
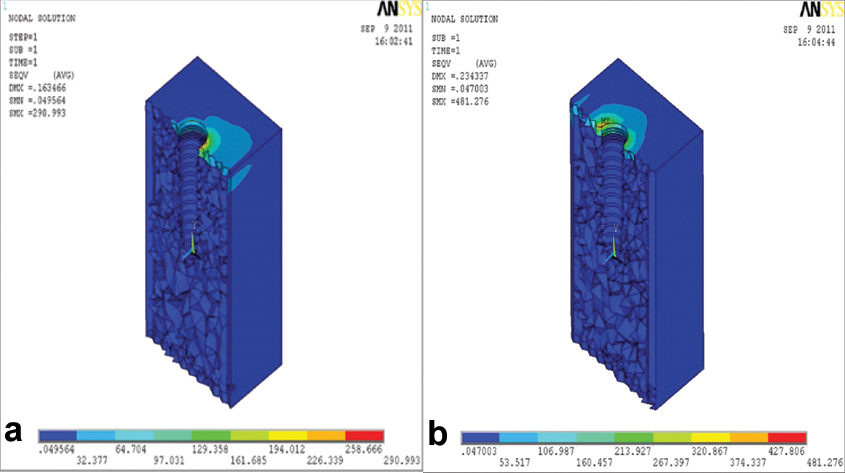
Hence, it was observed that the stresses were more in D4 type of bone than in D2 type of bone under vertical and oblique loading with both the variable and buttress thread designs.
While comparing these implant thread designs, the variable thread design showed less stresses than buttress thread design in D4 type of bone, while conclusive results were not obtained in D2 type of bone.
Discussion
Bone quality and implant thread design are two principal factors for the long term success of the implant. In terms of bone quality, D4 bone has the weakest biomechanical strength and the lowest Bone Implant Contact (BIC) area to distribute the load at the implant bone interface [5]. This may be the reason for higher failure rates in D4 type of bone than other bone densities. Use of threads in implant design is to increase stability, functional surface area of implants and to distribute the stress along the implant/bone interface [10,16,17]. It should be considered that the functional surface area requirements would increase from a minimum for an implant in D1 bone to a maximum for implants in the D4 bone [5]. So, for better result depending upon the bone quality at the location of dental implant placement, clinician should select appropriate implant thread design.
In the present study, both the above mentioned factors were considered. A comparative analysis was done using implants with two different thread designs in two different bone qualities. As three dimensional finite element analysis provides an actual representation of stress behaviour on the supporting bone, it was used in this study [3,10,18]. Results of the present study showed that the stresses were more in D4 type of bone than in D2 type of bone under vertical and oblique loading for variable as well as buttress thread design. Results of the present study were in agreement with the study done by Sevimay M et al., who studied the effect of different bone types on stress distribution in an implant supported mandibular crown and concluded that the stresses were more for D3 and D4 type of bone than D1 and D2 bone [3]. Holmes DC and Loftus JT did a study to evaluate the influence of bone quality on the transmission of occlusal forces for endosseous implants. The study concluded that the placement of implants in a dense bone would result in reduced micro-movement of the dental implant which would lead to reduced stress concentration and increased fixture stabilization [19]. The present study also compared buttress thread design and variable thread design in D2 and D4 type of bone. Comparison of variable and buttress thread design in D4 type of bone showed that variable thread design is better than the buttress thread design, considering the stresses transferred to the bone. This may be due to the square and V-shaped thread shape of variable thread design which provided better functional surface area which is important mainly in D4 type of bone. Dunder S et al., investigated the effect of implant thread geometry on the stress distributions in two different threaded dental implant systems (Nobel Active and Nobel Replace) that have the same diameter and length. They showed that the stresses were more with Nobel Replace system than in Nobel Active which has a variable thread design [10]. So, this study also supports results of the present study.
In a similar study, Geng JP et al., compared four different implant thread form configurations. The authors concluded that V-shaped thread and large square thread form configurations appear to be suitable for use but thin thread form should be avoided and small square thread form is not satisfactory [20]. In a two-dimensional finite element analysis, Chun HJ et al., compared implants with V-shaped, square and reverse-buttress grooves. It was observed that, the square thread implants were better in resisting shear forces [21]. In another study which compared threaded implant with the cylindrical implant in four different bone densities (D1, D2, D3, D4), it was found that the threaded dental implants created more stress in comparison to cylindrical implants. Hence, the authors concluded that cylindrical implant designs should be preferred for softer bone, than the threaded implant design [22].
Dental implants typically are fabricated from titanium or its alloys. The modulus of elasticity of titanium is more than 5 to 10 times that of cortical bone. A mechanical principle ‘composite beam analysis’ states that when two materials of different moduli are placed together with no intervening material and one is loaded, a stress contour increase will be observed wherever the two materials first come in contact. This phenomenon is observed in photoelastic materials and three dimensional finite element analysis studies when an implant is placed within a bone stimulant and loaded [2,23,24]. Results of this study were in accordance with this principle which was also confirmed by many other studies showing maximum stresses were concentrated in the cortical bone at the neck of the implant irrespective of type of bone [3,19,20,25-27].
Clinical Implication
It is better to use dental implants with newer variable thread design particularly in softer bone like D4 type of bone for better results than implants with buttress thread design.
Future Prospects
Similar study can be carried out with all implant thread designs in all four types of bone densities for better selection of implants based on bone quality and thread design.
Limitation
Several assumptions were made in this study. The structures in the model were considered as homogenous and isotropic. At the same time they possess linear elasticity. There may be differences in the properties of living tissues. For instance, it is reported that the cortical bone of the mandible is transversely non-homogeneous and isotropic [28]. In this study, the cement layer between abutment and crown was not considered. The occlusal anatomy of the model may influence the stress distribution pattern. In the present study the forces were applied specifically over the central fossa, buccal cusp tip and distal fossa. However, direction as well as the amount of the forces acting on the crown may differ. The occlusal form used for this model may not necessarily be the same for all molar teeth.
Conclusion
For both, variable and buttress thread design, von Mises stresses were more within D4 type of bone than D2 type of bone. These stresses were more concentrated at the neck of the implant within D2 as well as in D4 type of bone. In D4 type of bone, von Mises stresses were more for the buttress thread design than variable thread design. In view of the above conclusions, it may be inferred that variable thread design may be more suitable in softer bone.