Introduction
Gene therapy is a field of biomedicine that involves replacing or repairing defective genes in the diseased cell genome to restore normal cell function without causing any toxicity to non-target tissues. Since the first attempt at human gene therapy in 1980 [1], scientists and clinicians have been constantly researching and conducting trials [2].
Gene therapy can be somatic gene therapy or germ line gene therapy. Somatic gene therapy involves alteration of the genes in selected cells that are not gametes or undifferentiated cells. The effects of these changes are restricted to treated individuals. On the other hand, germ line gene therapy introduces permanent, inheritable changes to the genome of the individual, by targeting gametes. The state of gene therapy research is confined for ethical and technical reasons almost in its entirety to somatic cell gene therapy [3]. Typically, therapeutic genes are identified, isolated and cloned and then introduced into a vector. A vector is a vehicle that is used to deliver the gene of interest to the target tissue [Table/Fig-1]. A vector should deliver precise amount of material into each target cell. Vectors that have been experimented and used include viral and non-viral vectors. Viruses provide the most efficient form of gene transfer. Various viral vectors in experiment today are retroviruses, adenoviruses, adeno-associated viruses and herpes viruses. Non-viral vectors include electroporation, microinjection, use of ballistic particles, calcium vectors, lipid vectors and protein complexes [3].
Introduction of gene into the vector and transfer of the vector into the target cell.
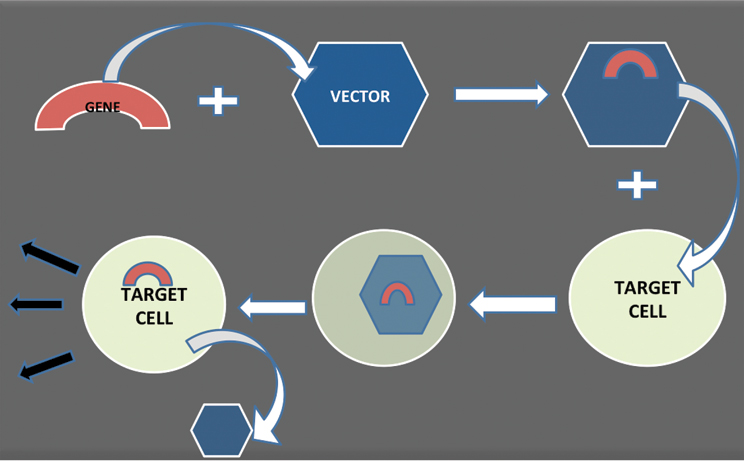
Although considered largely experimental, research is moving to clinical applications, with several commercial gene therapies now available for certain genetic conditions. Many dental conditions and dental applications are also being researched presently all over the world [4, 5]. Following is a brief review of gene therapy in dentistry.
Gene Therapy for Bone Repair
Bone loss may be a consequence of several oral conditions such as periodontal disease, trauma, neoplastic pathology, reconstructive surgery or may be deficient as a result of congenital defects. Currently, efforts at addressing such bone defects revolve primarily around using substitute materials that are either synthetic or harvested. The ideal goal would be to have directed regeneration of bone to meet the necessary needs. This would require manipulation of the critical aspects of bone physiology i.e., osteoinduction, differentiation of osteoblasts and the production of osteoid matrix, osteoconduction and mechanical stimulation [4].
This is where members of the Transformation Growth Factor (TGF) super-family such as Platelet Derived Growth Factors (PDGFs), Insulin-like Growth Factors (IGFs), Transforming Growth Factor-β (TGF-βs) and Bone Morphogenic Proteins (BMPs) have been employed for tissue engineering. When introduced to a site in sufficient concentration, they induce repair by creating molecular cues for native Mesenchymal Stem Cells (MSCs) to migrate, proliferate, differentiate and begin the cascade of osseous extracellular matrix production [6].
While most of these molecules act with overlapping roles, it is the group of BMPs (specifically BMP-2, BMP-4 and BMP-7) that have shown the maximum promise in their direct ability to induce bone formation, both when delivered in in vitro cultures [7] as well to local sites in vivo [8]. However, a problem encountered is that BMPs are degraded and have a short half-life and require sustained high dose delivery. Gene therapy has shown the ability to overcome these issues. The two basic gene therapy strategies employing BMPs are: (1) the delivery of BMP-related genes directly into the area of interest in vivo and (2) the seeding of ex vivo genetically-modified cells into a scaffold which is then implanted [3]. Both approaches permit the sustained delivery of elevated levels of BMPs to target tissues [6].
Studies have shown the ability of Ad-BMP-7 adenovirus vectors to transfer genes encoding for BMP-7 directly into tissues (direct gene transfer) and have monitored the expression of the transduced gene products from the neighbouring vector-inoculated cells [9, 10]. Similarly, the in vivo applicability of BMP-2 gene delivery to tissues of mandibular defects by means of an adenoviral vector has been tested successfully [8].
The ex vivo methods have been validated in several studies where gene transfer is accomplished in harvested cells (typically stem cells of mesenchymal origin, bone marrow derived, adipose derived or dedifferentiated muscle or fibroblast derived stem cells) in a tissue-culture environment and the transduced cells are then placed back in the host. This has been successfully shown in studies attempting BMP-gene transfer into MSCs [11, 12] and PDGF-gene transfer into periodontal cells [13]. Ex vivo methods are convenient since it does not invoke any immune response, the target cell for transduction can be chosen according to the site and multiple genes {such as vascular endothelial growth factor (VEGF) as well as BMP can be transduced into a single cell} [14]. This is counter balanced by the morbidity of harvesting cells pretreatment and the difficulty in isolating certain cell lines and subsequently expanding them.
Gene Therapy for Salivary Gland Disorders
Tumours and their surgical resection, autoimmune disorders such as Sjogren’s syndrome, postradiation fibrosis are some conditions that can cause loss of salivary gland tissue and therapy that can restore or regenerate damaged salivary glands tissue is much desired. Salivary glandular tissue lends itself well to gene therapy since it is easily accessible via retrograde instillation of medicines through the salivary ducts and is anatomically encapsulated from adjacent structures, thereby reducing concerns associated with viral vector transduction.
An additional application of salivary gene therapy, owing to the abundant exocrine salivary protein expression is the possibility of secreting genetically engineered substances. These may be used to secrete particular substances and overcome single protein deficiencies or to deliver therapy to local areas i.e. oesophageal, pharyngeal, oral areas [15].
Research groups using various animal models have reported salivary gland gene transfer for hormones such as growth hormone and insulin [16, 17]. These studies found the expression of transgene-proteins in bloodstream, i.e., endocrine activity from the salivary glands, which may be useful to treat systemic protein deficiency disorders.
Antimicrobial and anticandidial peptides such as histatin-3 have also been transduced into salivary glands by means of cDNA, offering a novel approach to the management of candidial infections in the upper-GIT using naturally occurring antifungal proteins [18]. This has potential application since Candida spp. are common opportunistic infection and are a notorious cause of mucositis in HIV-compromised individuals.
Postradiation salivary hypofunction has been addressed in primates by the modulation of non secretory cells which have survived radiation into a secretory phenotype by means of an insertion of a gene, aquaporin 1 (AQP1), by means of an adenovirus vector, AdhAQP1 [19].
In Sjögren’s-syndrome, the destruction of glandular tissue maybe isolated to salivary and lacrimal tissue, or may be accompanied by secondary autoimmune diseases such as rheumatoid arthritis. Due to the autoimmune nature of the disease, infiltrating CD4+ cells release proinflammatory mediators (cytokines interleutin-2, interferon-γ, tumour necrosis factor-α) as well as anti-inflammatory mediators (interleutin-4, interleutin-6, interleutin-10). The upregulation of genes coding for anti-inflammatory cytokines, therefore, has been pinpointed to offer relief for dry mouth, keratoconjunctivitis. This has been done in several studies by means of a recombinant adenovirus rAAV2hVIP, encoding the human-VIP gene (Vasoactive Intestinal Peptide) [20-22].
Gene Therapy for Chronic Pain Management
Chronic, intractable, neurologic pain within the oro facial distribution is a serious challenge to manage for the dental professional. Promising gene therapy in animal models may hold the key to solve these conditions. Researchers at the Mount Sinai School of Medicine have engineered a virus carrying a gene for an endogenous opioid, which is injected directly to the spinal fluid adjacent to the dorsal root ganglia. Since the dorsal root ganglia acts as a gateway to higher pain centres and opioids produce a morphine like blockade of this pain gateway, results lasting for up to three months have been achieved [23]. Similarly, naturally neurotropic viruses such as herpes simplex based vectors or lipid encapsulated plasmids coding for anti-inflammatory interleutin-10 have been employed to selectively inoculate neural and glial cells and inhibit allodynic neurotransmitters [24]. Researchers have also attempted direct gene therapy to the articular surface of the temporomandibular joint [25].
Gene Therapy for DNA Vaccinations
An effective caries vaccine or periodontal vaccine has been an elusive dream, with many researchers only achieving mixed success. Instead of delivering an attenuated microbe or isolated protein to the immune system, as was attempted earlier, DNA transfer of specific genes of selected microbes to salivary tissue has been experimentally attempted. In the study, Porphyromonas gingivalis fimbriae antigen was expressed by salivary cells after gene transfer resulting in the formation of antigens of s-IgA and IgG classes against the microbe, which would target the periodontal pathogen [26]. Additionally, the antigen would compete with the live organisms for attachment in dental plaque. Similar DNA vaccine attempts have been made for Mutans Streptococci as well [27].
Gene Therapy for Implant Osseointegration
Since alveolar bone loss is the primary reason for implant failure, and since the implant is a biologically passive structure, there is a strong interest in controlling the interaction of the bone implant interface by adjunctive means. Adenovirus-vectors encoding human Platelet Derived Growth Factor-β (PDGF-β) have been used to accelerate bone fill and improve bone density thereby improving implant osseointegration [28]. Recently, calcium phosphate nano-particles have been used as a non viral vector to transduce PDGF-gene plasmids to human fibroblasts [29].
Gene Therapy for Head, Neck, Oral Cancer
Gene therapy in cancer treatment broadly aims at expressing a gene product that will result in cancer cell death. This may be accomplished by various strategies: (1) addition of a tumour suppressor gene (gene addition therapy) e.g. p53 in cancer cells, (2) deletion of a defective tumour gene (gene excision therapy), (3) down regulation of expression of genes that control tumour growth, (4) enhancement of immune surveillance, (5) activation of pro drugs that have a chemotherapeutic effect and cause toxicity only to the tumour cells (‘suicide gene’ therapy), (6) antiangiogenic therapy to inhibit tumou angiogenesis, (7) “cancer vaccination” with genes for tumour antigens [30].
Gene addition therapy: The p53-expression alteration has been widely implicated in a variety of cancers as an early event in carcinogenesis, and p53 tumour suppressor gene addition has been attempted using a variety of viral vectors in various animal and clinical trials to trigger a pro-apoptotic anti-tumour effect [Table/Fig-2]. Retroviral, adeno-associated viral, herpesviral and adenoviral vectors have been tried, and of these adenoviral vectors have proven more successful than others, as they are capable of gene addition in both dividing as well as resting cells, without any permanent additions to the host genome. Even more successful has been the use of oncolytic viruses, which are designed to replicate only in cancerous cells lacking p53, to produce tumour regression in refractory cancers [31] or to reduce or produce precancerous dysplastic lesion regression [32].
Addition of gene p53 into the tumor cells and removal of the mutated p53 gene of the cell thus suppressing further multiplication of cancer cells.
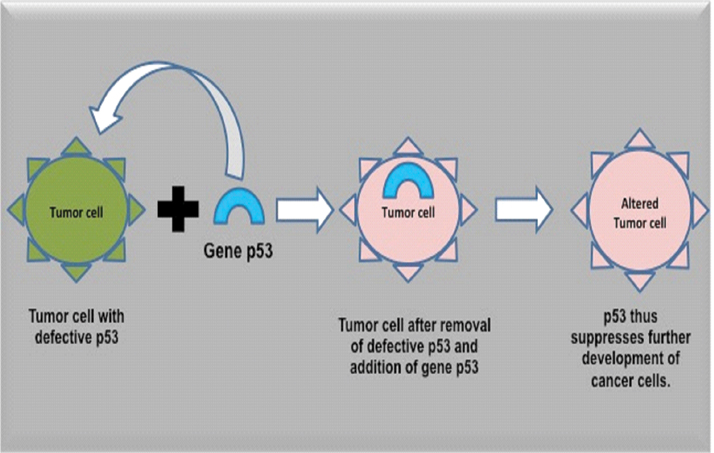
Mutation of the p27 gene has been associated with the inhibition of cell apoptosis in various cancers including Oral Squamous Cell Carcinoma (OSCC) and the effect of delivery of genetically engineered p27, which is resistant to the usual enzymatic degradation has been tested significantly inhibit tumour growth [33]. Other tumour suppressor gene addition candidates have been the retinoblastoma-1 gene and p16 gene.
Oncolytic viruses: Oncolytic viruses are DNA or RNA viruses that replicate only in tumour cells. The adenovirus ONYX-015 virus is a replication defective cytomegalovirus lacking the E1B viral protein, preventing replication in cells with a normal p53 pathway. This permits selective targeting of cancers with p53 mutations. ONYX-015 has been tested successfully in a Phase II human trial on subjects with head and neck carcinomas to produce highly selective tumour cell lysis and significant tumour regression [32] and in several Phase II and Phase III trials in refractory head and neck cancers [34]. In the form of Advexin© (Introgen Therapeutics, USA), ONYX-015 has been incorporated into a mouthwash in a clinical trial to administer p53 to premalignant cells, selectively resolving the dysplastic changes in up to 37% of cases [35].
Gene-excision therapy: Gene-excision therapy is another strategy to inhibit the growth of cancer cells. Here, early growth factor response factor 1, which controls cell growth and cell cycle progression including the expression of pro-neoplastic molecules such as TGF-β1, PDGF-α, is inhibited from phosphorylation by the action of Okadaic acid, a toxic polyether molecule [36].
Down-regulation of oncogene expression: Oncogene expression can be down regulated by the delivery of short chains of antisense-RNA complimentary to oncogenic DNA nucleotides. The resultant DNA/RNA complex is identified as a foreign message and is cleaved out enzymatically reducing the expression of genes such as the oncogenes of the bcl-family, myc, fos, and ras. This ‘antisense RNA’ strategy can be used not only to affect tumour growth but also the oncogenic viruses such as human papilloma virus, human t-lymphotropic virus and cytomegalovirus. The degree of down regulation is directly proportionate to the quantum of antisense RNA nucleotides that can be delivered to the tumour, and delivering such amounts is often a challenge [37]. However, clinical trials testing the ability of epidermal growth factor receptor delivered by liposome to affect oral non-Hodgkin lymphoma tumour growth have reported low toxicity, high efficacy and high titres [38].
Immunotherapy: This strategy involves administering antigens or adjuvants to the body’s own immune system or modifying the immunogenicity of cancer cells to increase their detection and destruction. Patients with carcinomas often present with numerous deficits in the functioning of immune cells such as NK cells, T-lymphocytes and dendritic cells. These deficits may involve generalized host immune deficits or they may be the result of localized cancer tumour evasion [39].
General passive immune activation, by administering cytokines such as Granulocyte- Macrophage Colony-Stimulating Factor (GM-CSF), alpha-Interferon (α-IFN), gamma-Interferon (γ-IFN), Interleukin 2 (IL-2) and Interleukin 12 (IL-12) act by increasing the anti-tumour response [39, 40]. General active immunity can also be stimulated by co-administering either whole replication defective cancer cells, or, purified tumour antigens as isolates or mixtures, with cytokines, or lab grown antigen presenting-cells, along with cytokines as adjuvants [39].
Tumour evasion happens when cancer cells express surface proteins that inactivate activated T-cells on binding. By developing monoclonal antibodies targeting the binding sites of activated T-cells such as anti-PD-1 (anti-Programmed cell Death Receptor-1) and anti-CTLA-4 (anti-Cytotoxic T-Lymphocyte-Associated protein-4), these immune checkpoints are inhibited from being turned off by the cancer cells, permitting destructive immunity to clear the neoplastic cells. Checkpoint blockers such as ipilimumab, pembrolizumab, nivolumab have been approved for human use and another antibody MPDL3280A is currently undergoing clinical trials [41].
Suicide gene therapy: The addition of suicide genes by viral vectors is another strategy for treating carcinomas where genes coding for a pro-drug are transfected to cells, via herpes virus or adenovirus. When these enzymes are synthesized intracellularly, they metabolize drugs which trigger cell death. The Herpes Simplex Virus-Thymidine Kinase (HSV-TK) triggers metabolism of ganciclovir to ultimately terminate DNA-synthesis [Table/Fig-3].
How introduction of gene HSV-TK helps in coding for enzyme that converts ganciclovir to triphosphate compound which is toxic to cancer cells.
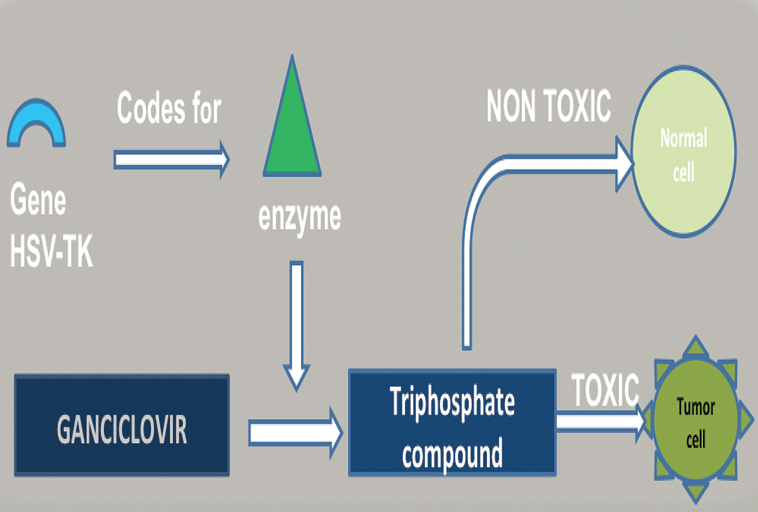
Gene Therapy to Keratinocytes
Keratinocytes are already used within medicine predictably, and protocols to graft, proliferate, differentiate or dedifferentiate and transplant sheets of keratinocytes already exist. These cultured keratinocytes are grafted to defects where they persist and maintain normal epithelial morphology. To these existing protocols, gene therapy can be added thereby allowing certain therapeutic genes to be added to a structure easily accessible to vectors and can easily be monitored. In the worst case, the epithelium can be excised.
Such keratinocytes transduced with genes to secrete biologically active molecules which can then be grafted to sites of interest. Precisely this approach was employed by a research group to express Factor IX in oral mucosal keratinocyte cultures [42], while others have attempted to transduce BMP-7 in an attempt to induce repair at bony defects [43]. Another area of research is the persistence of expression of molecules by the genetically modified tissue.
Gene Therapy for Orthodontic Tooth Movement
The activity of osteoclasts which are necessary for tooth remodelling during orthodontic therapy is mediated by the RANK-RANKL-OPG interaction. Here, RANKL refers to Receptor Activator of NF-kappa β-Ligand (RANKL) which is a receptor that binds to the RANK molecule and permits osteoclast precursor activation. Thus, inducing RANK-RANKL binding results in accelerated osteoclastogenesis and hastens orthodontic tooth movement by up to 150% and it may also be employed to overcome orthodontic challenges in moving ankylosed teeth [44].
On the other hand, Osteoprotegerin (OPG) is a competing receptor that also binds with RANK. However, OPG-RANK binding has an opposite effect to RANKL-RANK binding whereby osteoclast activation is blocked. Hence, tooth remodelling is slowed and orthodontic tooth movement is delayed up to 50% [45].
Control of the OPG gene transfer and RANKL gene transfer to periodontal tissues is being examined with interest by various research groups as a means of modulating orthodontic tooth movement.
Dentin and Periodontal Regeneration
Dentinal or pulp injury secondary to carious, restorative or traumatic process is traditionally treated by means of a pulp capping procedure, which induces dentin bridge formation. Apart from calcium hydroxide, biomolecules such as porcine enamel matrix derivative (Emdogain) [46] have been made commercially available and biomolecules expressed during dentinogenesis such as Odontogenic Ameloblast Associated Protein (ODAM) [47], Amelogenin, OP-1, TGF-β, BMP-4 and BMP-2 have been shown to work experimentally by direct instillation [48]. However, a matter of concern is that several of these biomolecules favour osseous healing which may predispose to ankylotic healing of periodontal tissues.
Dentist as a Teeth Bioengineer
Knowledge of the entire gamut of genes that control tooth formation is presently inconceivable, but in the distant future advances in research may permit the discovery and manipulation of the various genes and gene products that form the teeth and their supporting structures. In such a scenario, bioreactors may permit the engineering of tooth structures in vitro or in vivo. Already the discovery of ‘master genes’ that control morphogenesis and regionalization such as FGF-8 (Fibroblast Growth Factors, BMP-4, PAX-9, WNTs (Wingless Integrated) and SHHs (Sonic Hedge Hog), offer a road to explore ahead [49, 50].
Dentist as Gene Therapist
As explained earlier, salivary glands offer convenient access for gene therapy, which may elevate the role of the oral healthcare professional to that of a gene therapist [51]. Via gene therapy to the salivary glands, a window to the other parts of the body may be realized. Apart from modulating their usual exocrine expression through gene therapy, tissue engineering can manipulate the glands to have an endocrine role as well, permitting various distant therapeutic targets or systemic actions.
Future Challenges and Directions
While single nucelotide polymorphisms and single gene disorders are amenable to detection, analysis and genetic manipulation, the vast majority of traits and disorders are polygenic. In addition to involving numerous genes acting in concert, these genes are also situated at different loci. Further, susceptibility to such traits amongst individuals is dissimilar due to environmental factors exerting varying degrees of influence [52]. Also, genetic etiology for particular trait may be associated not only with disease causing genes but even with unrelated genes. Unravelling these complex interactions between genes is the biggest hurdle to making personalised medicine realisable.
To counter, these are several disruptive technologies that have the potential to greatly impact genetic research. Clustered regularly interspaced short palindromic repeats sequences enable rapid genetic editing [53]; gene-sequencers and computer processing power are scaling rapidly enabling whole genome sequencing to be done quickly and cost-effectively [54]; and algorithmic deep learning is helping to analyse gene expression and regulation [55].
Conclusion
Gene therapy has the potential to disrupt existing therapies or create therapies where none previously exist and undoubtedly, will revolutionize dentistry in the years to come. This review has presented some of the current approaches in gene therapy vis-à-vis dentistry. Clinical success is already apparent in several human trials and each success inches towards a future of predictive, preventive, personalised medicine.
[1]. Mercola KE, Cline MJ, Sounding boards. The potentials of inserting new genetic information N Engl J Med 1980 303(22):1297-300. [Google Scholar]
[2]. Misra S, Human gene therapy: a brief overview of the genetic revolution J Assoc Physicians India 2013 61(2):127-33. [Google Scholar]
[3]. Winn SR, Hu Y, Sfeir C, Hollinger JO, Gene therapy approaches for modulating bone regeneration Adv Drug Deliv Rev 2000 42(1-2):121-38. [Google Scholar]
[4]. Prabhakar A, Paul JM, Basappa N, Gene therapy and its implications in dentistry Int J Clin Pediatr Dent 2011 4(2):85-92. [Google Scholar]
[5]. Siddique N, Raza H, Ahmed S, Khurshid Z, Zafar MS, Gene therapy: A paradigm shift in dentistry Genes (Basel) 2016 7(11):98 [Google Scholar]
[6]. Porter JR, Ruckh TT, Popat KC, Bone tissue engineering: A review in bone biomimetics and drug delivery strategies Biotechnol Prog 2009 25(6):1539-60. [Google Scholar]
[7]. Reddi AH, Morphogenesis and tissue engineering of bone and cartilage: inductive signals, stem cells and biomimetic biomaterials Tissue Eng 2000 6(4):351-59. [Google Scholar]
[8]. Alden TD, Beres EJ, Laurent JS, Engh JA, Das S, London SD, The use of bone morphogenetic protein gene therapy in craniofacial bone repair J Craniofac Surg 2000 11(1):24-30. [Google Scholar]
[9]. Franceschi RT, Wang D, Krebsbach PH, Rutherford RB, Gene therapy for bone formation: in vitro and in vivo osteogenic activity of an adenovirus expressing BMP7 J Cell Biochem 2000 78(3):476-86. [Google Scholar]
[10]. Franceschi RT, Biological approaches to bone regeneration by gene therapy J Dent Res 2005 84(12):1093-103. [Google Scholar]
[11]. Gazit D, Turgeman G, Kelley P, Wang E, Jalenak M, Zilberman Y, Engineered pluripotent mesenchymal cells integrate and differentiate in regenerating bone: a novel cell-mediated gene therapy J Gene Med 1999 1(2):121-33. [Google Scholar]
[12]. Moutsatsos IK, Turgeman G, Zhou S, Kurkalli BG, Pelled G, Tzur L, Exogenously regulated stem cell-mediated gene therapy for bone regeneration Mol Ther 2001 3(4):449-61. [Google Scholar]
[13]. Zhu Z, Lee CS, Tejeda KM, Giannobile WV, Gene transfer and expression of platelet-derived growth factors modulate periodontal cellular activity J Dent Res 2001 80(3):892-97. [Google Scholar]
[14]. Peng H, Wright V, Usas A, Gearhart B, Shen HC, Cummins J, Synergistic enhancement of bone formation and healing by stem cell-expressed VEGF and bone morphogenetic protein-4 J Clin Invest 2002 110(6):751-59. [Google Scholar]
[15]. Baum BJ, Kok M, Tran SD, Yamano S, The impact of gene therapy on dentistry: a revisiting after six years J Am Dent Assoc 2002 133(1):35-44. [Google Scholar]
[16]. Goldfine ID, German MS, Tseng HC, Wang J, Bolaffi JL, Chen JW, The endocrine secretion of human insulin and growth hormone by exocrine glands of the gastrointestinal tract Nat Biotechnol 1997 15(13):1378-82. [Google Scholar]
[17]. He X, Goldsmith CM, Marmary Y, Wellner RB, Parlow AF, Nieman LK, Systemic action of human growth hormone following adenovirus-mediated gene transfer to rat submandibular glands Gene Ther 1998 5(4):537-41. [Google Scholar]
[18]. O’Connell BC, Xu T, Walsh TJ, Sein T, Mastrangeli A, Crystal RG, Transfer of a gene encoding the anticandidal protein histatin 3 to salivary glands Hum Gene Ther 1996 7(18):2255-61. [Google Scholar]
[19]. Delporte C, O’Connell BC, He X, Lancaster HE, O’Connell AC, Agre P, Increased fluid secretion after adenoviral-mediated transfer of the aquaporin-1 cDNA to irradiated rat salivary glands Proc Natl Acad Sci U S A 1997 94(7):3268-73. [Google Scholar]
[20]. Lodde BM, Mineshiba F, Kok MR, Wang J, Zheng C, Schmidt M, NOD mouse model for Sjogren’s syndrome: lack of longitudinal stability Oral Dis 2006 12(6):566-72. [Google Scholar]
[21]. Lodde BM, Mineshiba F, Wang J, Cotrim AP, Afione S, Tak PP, Effect of human vasoactive intestinal peptide gene transfer in a murine model of Sjogren’s syndrome Ann Rheum Dis 2006 65(2):195-200. [Google Scholar]
[22]. Kok MR, Yamano S, Lodde BM, Wang J, Couwenhoven RI, Yakar S, Local adeno-associated virus-mediated interleukin 10 gene transfer has disease-modifying effects in a murine model of Sjogren’s syndrome Hum Gene Ther 2003 14(17):1605-18. [Google Scholar]
[23]. Shilpashree HS, Sarapur S, Gene therapy in dentistry: a review N Y State Dent J 2013 79(5):60-64. [Google Scholar]
[24]. Pohl M, Fink DJ, A new player in gene therapy for pain? Gene Ther 2008 15(13):953-54. [Google Scholar]
[25]. Kuboki T, Nakanishi T, Kanyama M, Sonoyama W, Fujisawa T, Kobayashi K, Direct adenovirus-mediated gene delivery to the temporomandibular joint in guinea-pigs Arch Oral Biol 1999 44(9):701-09. [Google Scholar]
[26]. Kawabata S, Terao Y, Fujiwara T, Nakagawa I, Hamada S, Targeted salivary gland immunization with plasmid DNA elicits specific salivary immunoglobulin A and G antibodies and serum immunoglobulin G antibodies in mice Infect Immun 1999 67(11):5863-68. [Google Scholar]
[27]. Fan M, Bian Z, Peng Z, Guo J, Jia R, Chen Z, DNA vaccine encoding Streptococcus mutans surface protein protected gnotobiotic rats from caries Zhonghua Kou Qiang Yi Xue Za Zhi 2002 37(1):04-07. [Google Scholar]
[28]. Chang PC, Cirelli JA, Jin Q, Seol YJ, Sugai JV, D’Silva NJ, Adenovirus encoding human platelet-derived growth factor-B delivered to alveolar bone defects exhibits safety and biodistribution profiles favorable for clinical use Hum Gene Ther 2009 20(5):486-96. [Google Scholar]
[29]. Elangovan S, Jain S, Tsai PC, Margolis HC, Amiji M, Nano-sized calcium phosphate particles for periodontal gene therapy J Periodontol 2013 84(1):117-25. [Google Scholar]
[30]. Ayllon Barbellido S, Campo Trapero J, Cano Sanchez J, Perea Garcia MA, Escudero Castano N, Bascones Martinez A, Gene therapy in the management of oral cancer: review of the literature Med Oral Patol Oral Cir Bucal 2008 13(1):E15-21. [Google Scholar]
[31]. Nemunaitis J, Cunningham C, Tong A, Post L, Netto G, Paulson AS, Pilot trial of intravenous infusion of a replication-selective adenovirus (ONYX-015) in combination with chemotherapy or IL-2 treatment in refractory cancer patients Cancer Gene Ther 2003 10(5):341-52. [Google Scholar]
[32]. Nemunaitis J, Ganly I, Khuri F, Arseneau J, Kuhn J, McCarty T, Selective replication and oncolysis in p53 mutant tumors with ONYX-015, an E1B-55kD gene-deleted adenovirus, in patients with advanced head and neck cancer: a phase II trial Cancer Res 2000 60(22):6359-66. [Google Scholar]
[33]. Supriatno Harada K, Kawaguchi S, Onoue T, Yoshida H, Sato M, Characteristics of antitumor activity of mutant type p27Kip1 gene in an oral cancer cell line Oral Oncol 2004 40(7):679-87. [Google Scholar]
[34]. INGN 201: Ad-p53, Ad5CMV-p53, adenoviral p53, p53 gene therapy-introgen, RPR/INGN 201 Drugs R D 2007 8(3):176-87. [Google Scholar]
[35]. Rudin CM, Cohen EE, Papadimitrakopoulou VA, Silverman S Jr, Recant W, El-Naggar AK, An attenuated adenovirus, ONYX-015, as mouthwash therapy for premalignant oral dysplasia J Clin Oncol 2003 21(24):4546-52. [Google Scholar]
[36]. Okamura H, Morimoto H, Fujita M, Nasu F, Sasakia E, Haneji T, Suppression of Egr-1 expression in human oral squamous carcinoma cells by okadaic acid Oral Oncol 2002 38(8):779-84. [Google Scholar]
[37]. Gavrilov K, Saltzman WM, Therapeutic siRNA: principles, challenges, and strategies Yale J Biol Med 2012 85(2):187-200. [Google Scholar]
[38]. Waters JS, Webb A, Cunningham D, Clarke PA, Raynaud F, Di Stefano F, Phase I clinical and pharmacokinetic study of bcl-2 antisense oligonucleotide therapy in patients with non-Hodgkin’s lymphoma J Clin Oncol 2000 18(9):1812-23. [Google Scholar]
[39]. Disis ML, Mechanism of action of immunotherapy Semin Oncol 2014 41(Suppl 5):S3-13. [Google Scholar]
[40]. Rosenberg SA, IL-2: the first effective immunotherapy for human cancer J Immunol 2014 192(12):5451-58. [Google Scholar]
[41]. La-Beck NM, Jean GW, Huynh C, Alzghari SK, Lowe DB, Immune checkpoint inhibitors: new insights and current place in cancer therapy Pharmacotherapy 2015 35(10):963-76. [Google Scholar]
[42]. Nanba D, Matsushita N, Toki F, Higashiyama S, Efficient expansion of human keratinocyte stem/progenitor cells carrying a transgene with lentiviral vector Stem Cell Res Ther 2013 4(5):127 [Google Scholar]
[43]. Rutherford RB, Racenis P, Fatherazi S, Izutsu K, Bone formation by BMP-7-transduced human gingival keratinocytes J Dent Res 2003 82(4):293-97. [Google Scholar]
[44]. Kanzaki H, Chiba M, Arai K, Takahashi I, Haruyama N, Nishimura M, Local RANKL gene transfer to the periodontal tissue accelerates orthodontic tooth movement Gene Ther 2006 13(8):678-85. [Google Scholar]
[45]. Kanzaki H, Chiba M, Shimizu Y, Mitani H, Dual regulation of osteoclast differentiation by periodontal ligament cells through RANKL stimulation and OPG inhibition J Dent Res 2001 80(3):887-91. [Google Scholar]
[46]. Nakamura Y, Hammarstrom L, Lundberg E, Ekdahl H, Matsumoto K, Gestrelius S, Enamel matrix derivative promotes reparative processes in the dental pulp Adv Dent Res 2001 15:105-07. [Google Scholar]
[47]. Yang IS, Lee DS, Park JT, Kim HJ, Son HH, Park JC, Tertiary dentin formation after direct pulp capping with odontogenic ameloblast-associated protein in rat teeth J Endod 2010 36(12):1956-62. [Google Scholar]
[48]. Goldberg M, Six N, Decup F, Buch D, Majd ES, Lasfargues J-J, Application of bioactive molecules in pulp-capping situations Adv Dent Res 2001 15(1):91-95. [Google Scholar]
[49]. Peters H, Neubuser A, Balling R, Pax genes and organogenesis: Pax9 meets tooth development Eur J Oral Sci 1998 106(Suppl 1):38-43. [Google Scholar]
[50]. Koussoulakou DS, Margaritis LH, Koussoulakos SL, A curriculum vitae of teeth: evolution, generation, regeneration Int J Biol Sci 2009 5(3):226-43. [Google Scholar]
[51]. Baum BJ, Alevizos I, Chiorini JA, Cotrim AP, Zheng C, Advances in saliveary gland gene therapy-oral and systemic implications Expert Opinion on Biological Therapy 2015 15(10):1443-54. [Google Scholar]
[52]. Boyle EA, Li YI, Pritchard JK, An expanded view of complex traits: from polygenic to omnigenic Cell 169(7):1177-86. [Google Scholar]
[53]. Wang T, Wei JJ, Sabatini DM, Lander ES, Genetic screens in human cells using the CRISPR-Cas9 system Science 2014 343(6166):80-84. [Google Scholar]
[54]. Zhang J, Chiodini R, Badr A, Zhang G, The impact of next-generation sequencing on genomics J Genet Genomics 2011 38(3):95-109. [Google Scholar]
[55]. Chen Y, Li Y, Narayan R, Subramanian A, Xie X, Gene expression inference with deep learning Bioinformatics 2016 32(12):1832-39. [Google Scholar]