Introduction
Calcium silicate-based cements physical properties is influenced by environmental changes.
Aim
Here, we intended to evaluate the effect of storage medium on surface porosity of root Mineral Trioxide Aggregate (MTA) and Biodentine cement.
Materials and Methods
A total of 40 polyethylene tubes were selected and divided into two groups: Group A (MTA) and Group B (Biodentine). Each group was subdivided into two subgroups (n=10). In subgroups A1 and B1, tubes were transferred to Distilled Water (DW), while samples of subgroup A2 and B2 were transferred to Synthetic Tissue Fluid (STF) as storage medium and samples were stored for three days. All specimens were then placed in a desiccator for 24 hours and then subject to surface porosity evaluation by Scanning Electron Microscopy (SEM) at ×500, ×1000, ×2000 and ×5000 magnifications. The number and the surface porosities were determined by Image J analysis. Data were analyzed by ANOVA at level of significance of p<0.05.
Results
The lowest surface porosity was observed in MTA samples stored in STF and the highest was in Biodentine samples stored in DW. Significant differences were noted between groups and subgroups of each group (p< 0.05). MTA samples stored in DW and STF showed significantly lower surface porosities compared to Biodentine samples (p < 0.05).
Conclusion
Storage medium can drastically affect the surface porosity of tested calcium silicate-based cements. However, MTA showed lower surface porosity compared to Biodentine cement, which can result in lower microleakage in applied area.
Introduction
The purpose of endodontic treatment is to remove oral pathogens from the root canal and periapical tissues and maintain a long-term periapical health. Penetration of microorganisms from the oral cavity into the filled root canal system is known as coronal leakage, while infiltration of the apical segment of the root by molecules like peptides that support microbial metabolism is termed apical leakage. In essence, there are three routes of leakage including 1) between material and tooth interface, 2) between core material and sealer interface and 3) within the material itself [1,2].
Calcium silicate-based cements have a wide range of applications in endodontics due to their unique chemical and physical properties [3]. Calcium silicate-based cements have sealing ability against microleakage [4]. The use of these cements has expanded to many applications of root repair including retrograde filling, direct pulp capping, indirect pulp capping, repair of root and furcation perforations, apexification and pulpotomy [4,5]. MTA is the most commonly used calcium silicate-based cements composed of 75% Portland cement, 20% bismuth oxide, 5% gypsum and trace amounts of Na2SO4, K2SO4, SiO2, MgO and CaO. Biodentine is a recently introduced new version of calcium silicate-based cement, which is introduced as a restorative cement [6].
Porosity is a measure of the void spaces in a substance and is a fraction of the volume of voids over the total volume, between 0-1, or as a percentage between 0%-100% [7]. Surface porosity is defined as the void spaces in the surface, which can increase the permeability of set material. Although, porosity of a material may not have a direct influence on its leakage characteristics, it may have an impact on numerous other factors including adsorption, permeability, strength and density [8]. The porosity may act as a portal of entry for microorganisms called microleakage [9]. Porosity is directly related to leakage behavior of root filling material. Porosimetry is the measuring volume, size, density and distribution porosity of subjected material. The porosity of a material affects its physical properties and, subsequently its behavior in its surrounding environment. These will help in understanding the formation, structure and potential use of many substances. Raskin A et al., [6] revealed that Biodentine exhibits better leakage resistance both to enamel and to dentine compared to Fuji II LC. However, the surface porosity of dental material can lead to microleakage and failure of endodontic treatments [8].
It was reported that the environmental changes might affect the physical properties of dental materials such as sealing ability. Previous studies showed that storage temperature or STF could affect the sealing ability and microleakage values of calcium silicate-based cements [10-12]. However, the effect of storage medium on the surface porosity has yet to be determined. Present study intended to evaluate the surface porosities of root MTA and Biodentine in two different storage medium including DW and STF.
Materials and Methods
This scientific study was performed in Dental Faculty of Azad University in Tehran, Iran. The study was performed from 2011-2013.
Specimen Preparation
Root MTA (Baran Darou Parse, Tabriz, Iran) and Biodentine cements (Septodont, Lyon, France) were used in this study. Each cement powder was mixed according to the manufacturer’s instruction and packed into a cylindrical polyethylene tube with an internal diameter of 20 mm and a height of 5 mm using a non surgical manual MTA carrier (Dentsply, TulsaDental, USA) and hand pressure according to the previous studies [13, 14]. The cylindrical tubes were then stored in an incubator for 72 hour; two groups in DW as control groups and two groups in STF. The STF was prepared as follows: 1.7 g of KH2PO4, 11.8 g of Na2HPO4, 80.0 g of NaCl and 2.0 g of KCl in 10 L of H2O (pH=7.2) according to a previous study [4]. The STF was renewed every day. There were four experimental groups:
Group A1: 10 tubes of MTA stored in DW.
Group A2: 10 tubes of MTA stored in STF.
Group B1:10 tubes of Biodentine stored in DW.
Group B2:10 tubes of Biodentine stored in STF.
Finally, all the specimens were stored in a desiccator for 24 hours prior to SEM analysis.
Scanning Electron Microscopy (SEM) Analysis
After drying, specimens were polished and sputter-coated with 10 nm of gold and evaluated under a scanning electron microscope (TESCAN, Tesla, Czech Republic). Secondary Electron (SE) and Back-Scattered Electron (BSE) detectors at ×500,×1000, ×2000 and ×5000 magnifications were selected. EDS color dot map analysis was performed for each sample at each magnification. Photomicrographs were taken and analyzed at desired magnifications. Digital images were recorded using a Microsoft picture manager (Redmond, WA, USA) to standardize each picture at 600×800 pixels. Surface porosity was calculated using the Image J program (Rasband WS, ImageJ; US National Institute of Health, Bethesda, MD, USA) according to the previous studies [12, 15]. Each figure was inverted [Table/Fig-1a-c] by this program and brightness was adjusted to select the orifices of each figure [Table/Fig-1c,d]. Binary images were made considering the orifices of the pores as outlined and the total number of outlines and the total pore surface in each micrograph was calculated in μm2 [Table/Fig-1e-g].
Sequence of image analysis using Image J. a) Micrograph of White Mineral Trioxide Aggregate (WMTA) polished perpendicularly; b) Image is inverted for easy calculation; c) Enhanced contrast. d) Conversion to 8-bit type for better calculation. e) Threshold adjustment for better calculation; f) Calculation of the amount of circles or ellipses in the micrograph in regards to the scale; g) The mean ± standard deviation of the number of outlines of total pores in μm2 in each micrograph were calculated for each sample in experimental groups.
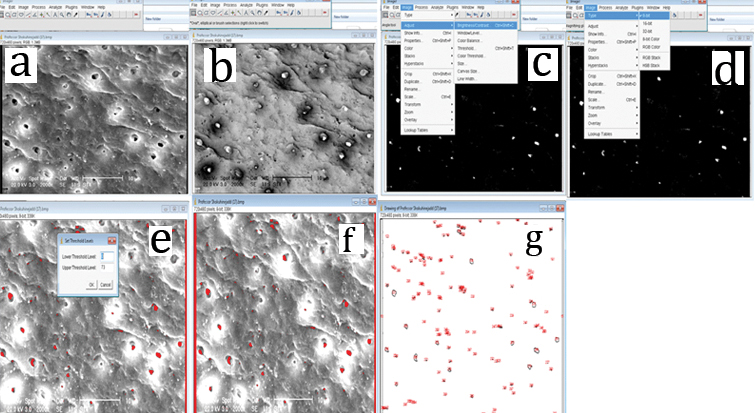
Statistical Analysis
Data means and SD were elaborated by a spreadsheet (Excel; Microsoft Corp, Redmond, Wash). The statistical program of SPSS 9.0 (SPSS Inc., Chicago, IL, USA) was used. The data were analyzed by using one-way analysis of variance (ANOVA) test.
Results
Analysis of Number and Surface Area Covered by Porosities
The means and standard deviations of the number of total porosities and the surface area covered by porosities in μm2 of experimental groups are shown in [Table/Fig-2]. In ×500, ×1000 and ×2000 magnification micrographs, significant differences were noted for the number of total porosities and the surface area covered by porosities in both DW and STF storage medium inside each experimental group and between groups (p<0.001). However, in ×5000 magnification micrographs, the number of total porosities and the surface area covered by porosities of Biodentine and MTA samples stored in DW and storage medium did not show any significant differences (p<0.2). While at ×5000 magnification, the number of total porosities and the surface area covered by porosities of Biodentine and MTA samples stored in STF, showed significant differences (p<0.01).
The number of total porosities and the surface area covered by porosities in μm2 for MTA and Biodentine cements in each magnification.
Variables | Magnification | ×500 | ×500 | ×1000 | ×1000 | ×2000 | ×2000 | ×5000 | ×5000 |
---|
Substance | Media | Number | Surface | Number | Surface | Number | Surface | Number | Surface |
MTA | Distilled water | 23.6±7.69 | 48±11.02 μm2 | 24.6±9.46 | 20.4±4.47 μm2 | 10.5±3.44 | 5.7±2.1 μm2 | 7.2±2.22 | 5.9±1.81 μm2 |
STF | 11.4±4.65 | 24±5.09 μm2 | 14.1±5.18 | 8.6±2.65 μm2 | 5.7±2.6 | 2.5±1.02 μm2 | 2.6±0.66 | 2.1±0.94 μm2 |
Biodentine | Distilled water | 41±9.96 | 52.3±7.18 μm2 | 23.3±2.6 | 32.2±8.38 μm2 | 14.8±5.4 | 10.8±2.71 μm2 | 9.5±3.32 | 5.5±2.61 μm2 |
STF | 21.1±5.94 | 26.3±3.92 μm2 | 14.6±9.14 | 15.7±4.47 μm2 | 14.6±21.59 | 8.2±2.89 μm2 | 4.2±1.93 | 3.2±0.87 μm2 |
*Numbers were multiplied by 10-3
SEM Analysis
The SEM images of the samples at ×1000 are presented in [Table/Fig-3]. As shown in this figure, samples stored in DW indicate more irregularities and porosities at the surface of cement [Table/Fig-3a, c]; please note arrows). However, the SEM images of surface of samples stored in STF medium showed more homogenous and regular contribution of cement material and lesser porosities [Table/Fig-3b,d]; please note arrows). The comparison of SEM images of experimental groups showed that MTA samples stored in DW and STF storage medium showed lesser irregularities and surface porosities compared to Biodentine samples [Table/Fig-3a,b]. The SEM images of Biodentine samples stored in DW showed large irregularities and porosities with large amount to unmixed cement powder, while the images of sample stored in STF showed better hydration and mixture of powder with lesser porosities [Table/Fig-3c,d].
The SEM images of MTA and Biodentine samples at ×1000 magnification (200 μm scale). a) SEM image of MTA sample stored in DW, Please note the pores formed at the surface of cement due to storage in DW (green arrows). b) SEM image of MTA sample stored in STF. Please note the uniform distribution of cement materials on the surface, which presents lesser irregularities and porosities (red arrows).c) SEM image of the Biodentine sample stored in DW. Please note the large amount of irregularities and unmixed powder particles at the surface due to storage in DW (orange arrows).d) SEM image of the Biodentine sample stored in STF. Please note the large size and also cleft like irregularities and porosities shown on the surface (blue arrows).
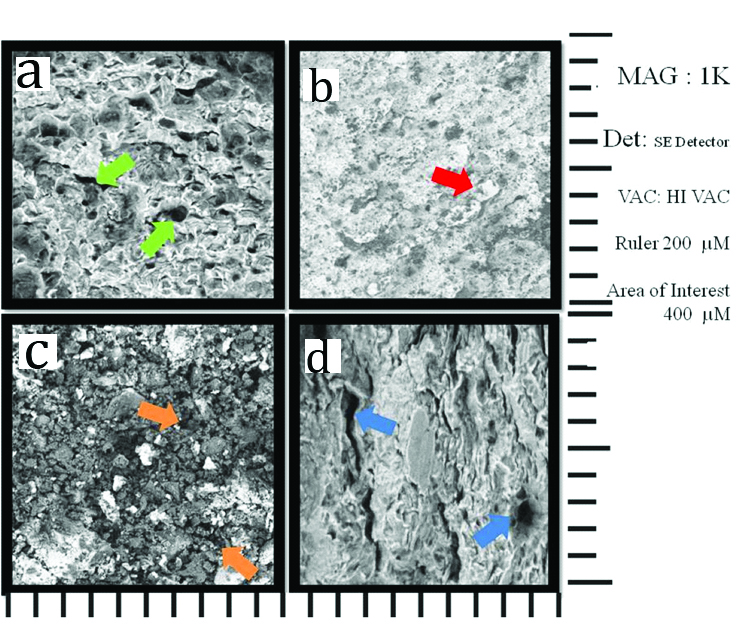
Discussion
Present study intended to evaluate the effect of two storage medium including STF and DW on surface porosity of MTA and Biodentine cements. The result of this study showed that the number of porosities and the surface covered by porosities in Biodentine samples were higher than MTA in STF and DW. Infact, the presence of more porosity in Biodentine might be related to the different components [5,6] procedure of mixing and powder to liquid ratio compared to MTA [16]. The main component of the Biodentine powder is tricalcium silicate and dicalcium silicate, with the addition of CaCO3 as filler and ZrO2 as a radiopacifier. The liquid is a solution of CaCl2 (accelerator of setting) with a water reducing agent [5,6]. The addition of zirconium oxide doesn’t react with base of calcium silicate cements so does not affect the hydration of the cements. In other words, the zirconium oxide is an inert filler in the matrix of Biodentine causes porosity [17]. CaCl2 accelerates the setting reaction [5,6]. If a setting reaction occurs very fast, it causes more porosity. Also, one of the other strategies for reducing the setting time is decreasing of the liquid content in the system [5]. This results in incomplete hydration of powder that produces more porosity [18]. This issue was also detected in SEM images of Biodentine sample stored in DW [Table/Fig-3c] In relation to the powder to liquid ratio, as we know, MTA is hand-mixed using a 3:1 powder to liquid ratio.
For Biodentine, liquid from the single-dose container is added into the powder-containing capsule and triturated with an amalgamator [16]. These amounts show more liquid in Biodentine can also affect more porosity for this cement [18]. In comparison of hand-mixing preparation of MTA, Biodentine is mixed by trituration [16]. Mechanical mixing like trituration could increase compressive strength [19], but this factor reduces the setting time [16], which can affect the porosity indirectly. In some previous investigations, normal saline or tap water was used as storage medium for MTA cement [20-22]. However, in other studies, authors have used phosphate containing solution for this purpose to mimic the clinical situation [11,23-25]. It was indicated that STF as storage medium can reduce the microleakage value in MTA cement [11]. Other investigators showed that phosphate buffer solutions can decrease the bacterial penetration or microleakage of calcium silicate cements due to formation of crystals over these cements [25]. Similar results were reported by Taddei P et al., where these authors reported that superficial biocoating layer can be formed on the surface of these cements when they were soaked in phosphate-rich solution during a time period of one-six months. It was explained that when calcium silicate cements are soaked in lower concentration phosphate solution, the amount of apatite precipitation is decreased and the time for apatite formation is prolonged [26,27].
The high amount of phosphate represents the continuous replenishment of phosphate ions from tissue fluids [28,29] and the absence of calcium causes the release of calcium ions from the cements, the limiting parameter in the precipitation reaction [21]. The studies have reported that Portland cements release calcium and when soak in phosphate solutions, induce apatite formation and produces high alkalinity in surrounding environment [4]. The hydration reaction of calcium silicate cement produces calcium silicate (C–S–H) gel and a large amount of calcium hydroxide. Calcium-silicate cements like MTA aged in physiological-like phosphate solutions such as STF produce a superficial biocoating formed by carbonated apatite and calcite (calcium carbonate) deposits can affect surface porosity [30, 31]. Infact, the reaction between these cements and STF is as following: Calcium ions supplied by the rapid dissolution of calcium hydroxide can react with environmental carbonate ions to form a calcium carbonate (calcite) superficial layer. Calcium carbonate may precipitate at the surface and in the cement paste porosity forming a protective layer of calcium carbonate on the outer zone of cement discs [32]. This calcium rich layer may absorb phosphate from the STF allowing the crystallization of apatite crystals [30]. These reactions are as follow:
2(3CaO.SiO2) + 6 H2O→3CaO.2SiO2 .3H2O + 3Ca (OH) 2
Ca (OH) 2→Ca2+ + H2O
Ca2+ + CO3-→CaCO3 (Calcite)
CaCO3 + PO43-→3 CaCO3. PO4 (Apatite)
The apatite content in the coating layer increases over soaking time [33]. This layer decreases microleakage by filling gaps in dentine or at the dentine-material interface [25,34]. In other words, presence of solution containing ion like phosphate can affect calcium silicate based cements and produce a superficial layer on the substance and therefore formation of this layer can affect chemical and physical properties of cement. Similar outcomes were noticed in present study as the SEM analysis of MTA samples showed better distribution of powder particles and uniform cement surface with lesser porosities specially in samples stored in STF medium [Table/Fig-3 a,b].
Goldberg M et al., showed the apatite formation after soaking in phosphate solution for Biodentine [35]. Han L and Okiji T demonstrated that both Biodentine and MTA caused the uptake of Ca and Si in the adjacent root canal dentine in the presence of phosphate buffered saline. The dentine ion uptake was more prominent for Biodentine than MTA at 30 and 90 days [36]. This result showed that more Ca can be released from Biodentine, which causes more porosity in this cement. These outcomes are consistent with present study results. Although, biological compounds, particularly the STF effect on bone metabolism has been proved [30], In our study, STF provided a significant positive effect in reducing the porosity, which can be regarded as a capacity to improve properties such as solubility and setting time in general. We recommend the immersion of calcium silicate cement in biological environment like STF in clinical scenario in order to reduce the surface porosity and leakage of set cement.
As an eye to the future, it will be beneficial to design more investigations, especially in vivo studies, in order to testify the outcomes of present study in clinical situation.
Conclusion
According to the present study results, it can be concluded that storage medium is an effective factor that can influence the porosity of tested calcium silicate-based cements. However, MTA showed lower surface porosity compared to Biodentine cement, which can result in lower microleakage in applied areas. Future studies in this area are needed to assess the storage medium may affect the bulk porosity of calcium silicate-based cements.
[1]. Veríssimo DM, do Vale MS, Methodologies for assessment of apical and coronal leakage of endodontic filling materials: a critical review Journal of oral science 2006 48(3):93-8. [Google Scholar]
[2]. Saghiri MA, Lotfi M, Saghiri AM, Vosoughhosseini S, Fatemi A, Shiezadeh V, Effect of pH on sealing ability of white mineral trioxide aggregate as a root-end filling material J Endod 2008 34(10):1226-29. [Google Scholar]
[3]. Milutinović-Nikolić AD, Medić VB, Vuković ZM, Porosity of different dental luting cements Dental Materials 2007 23(6):674-78. [Google Scholar]
[4]. Sarkar N, Caicedo R, Ritwik P, Moiseyeva R, Kawashima I, Physicochemical basis of the biologic properties of mineral trioxide aggregate J Endod 2005 31(2):97-100. [Google Scholar]
[5]. Laurent P, Camps J, De Méo M, Déjou J, About I, Induction of specific cell responses to a Ca(3)SiO(5)-based posterior restorative material Dent Mater 2008 24(11):1486-94. [Google Scholar]
[6]. Raskin A, Eschrich G, Dejou J, In vitro microleakage of Biodentine as a dentin substitute compared to Fuji II LC in cervical lining restorations Journal of Adhesive Dentistry 2012 14(6) [Google Scholar]
[7]. Li S, Roy DM, Investigation of relations between porosity, pore structure, and C1− diffusion of fly ash and blended cement pastes Cement and Concrete Research 1986 16(5):749-59. [Google Scholar]
[8]. Saghiri MA, Asgar K, Lotfi M, Karamifar K, Neelakantan P, Ricci JL, Application of mercury intrusion porosimetry for studying the porosity of mineral trioxide aggregate at two different pH Acta Odontol Scand 2012 70(1):78-82. [Google Scholar]
[9]. Dammaschke T, A new bioactive cement for direct pulp capping International Dentistry, African Edition. 2012 2012 2(2) [Google Scholar]
[10]. Saghiri MA, Asgar K, Lotfi M, Nazari A, Karamifar K, Neelakantan P, Effect of storage temperature on sealing ability and solubility of White Mineral Trioxide Aggregate Acta Odontol Scand 2012 70(6):536-40. [Google Scholar]
[11]. Lotfi M, Vosoughhosseini S, Saghiri MA, Rahimi S, Zand V, Reyhani MF, Effect of synthetic tissue fluid on microleakage of grey and white mineral trioxide aggregate as root-end filling materials: an in vitro study Sultan Qaboos University Medical Journal 2012 12(3):323 [Google Scholar]
[12]. Saghiri MA, Asgar K, Lotfi M, Garcia-Godoy F, Nanomodification of mineral trioxide aggregate for enhanced physiochemical properties Int Endod J 2012 45(11):979-88. [Google Scholar]
[13]. Saghiri MA, Lotfi M, Saghiri AM, Vosoughhosseini S, Aeinehchi M, Ranjkesh B, Scanning electron micrograph and surface hardness of mineral trioxide aggregate in the presence of alkaline pH J Endod 2009 35(5):706-10. [Google Scholar]
[14]. Aminoshariae A, Hartwell GR, Moon PC, Placement of mineral trioxide aggregate using two different techniques J Endod 2003 29(10):679-82. [Google Scholar]
[15]. Saghiri MA, García-Godoy F, Asgar K, Lotfi M, The effect of Morinda Citrifolia juice as an endodontic irrigant on smear layer and microhardness of root canal dentin Oral Science International 2013 10(2):53-57. [Google Scholar]
[16]. Leiendecker AP, Qi Y-P, Sawyer AN, Niu L-N, Agee KA, Loushine RJ, Effects of calcium silicate–based materials on collagen matrix integrity of mineralized dentin Journal of Endodontics 2012 38(6):829-33. [Google Scholar]
[17]. Camilleri J, Cutajar A, Mallia B, Hydration characteristics of zirconium oxide replaced Portland cement for use as a root-end filling material Dental Materials 2011 27(8):845-54. [Google Scholar]
[18]. Živica V, Effects of the very low water/cement ratio Construction and building materials 2009 23(12):3579-82. [Google Scholar]
[19]. Basturk FB, Nekoofar MH, Günday M, Dummer PM, The effect of various mixing and placement techniques on the compressive strength of mineral trioxide aggregate Journal of Endodontics 2013 39(1):111-14. [Google Scholar]
[20]. Fischer EJ, Arens DE, Miller CH, Bacterial leakage of mineral trioxide aggregate as compared with zinc-free amalgam, intermediate restorative material, and Super-EBA as a root-end filling material J Endod 1998 24(3):176-79. [Google Scholar]
[21]. De-Deus G, Petruccelli V, Gurgel-Filho E, Coutinho-Filho T, MTA versus Portland cement as repair material for furcal perforations: a laboratory study using a polymicrobial leakage model International Endodontic Journal 2006 39(4):293-98. [Google Scholar]
[22]. Torabinejad M, Rastegar AF, Kettering JD, Pitt Ford TR, Bacterial leakage of mineral trioxide aggregate as a root-end filling material Journal of Endodontics 1995 21(3):109-12. [Google Scholar]
[23]. Rahimi S, Shahi S, Lotfi M, Yavari HR, Charehjoo ME, Comparison of microleakage with three different thicknesses of mineral trioxide aggregate as root-end filling material Journal of Oral Science 2008 50(3):273-77. [Google Scholar]
[24]. Lotfi M, Vosoughhosseini S, Saghiri M, Zand V, Yavari HR, Kimyai S, Effect of alkaline ph on sealing ability of white mineral trioxide aggregate Med Oral Patol Oral Cir Bucal 2011 16(7):e1014-16. [Google Scholar]
[25]. Parirokh M, Askarifard S, Mansouri S, Haghdoost AA, Raoof M, Torabinejad M, Effect of phosphate buffer saline on coronal leakage of mineral trioxide aggregate Journal of Oral Science 2009 51(2):187-91. [Google Scholar]
[26]. Taddei P, Tinti A, Gandolfi M, Rossi PL, Prati C, Vibrational study on the bioactivity of Portland cement-based materials for endodontic use Journal of Molecular Structure 2009 924:548-54. [Google Scholar]
[27]. Taddei P, Tinti A, Gandolfi MG, Rossi PL, Prati C, Ageing of calcium silicate cements for endodontic use in simulated body fluids: a micro-Raman study Journal of Raman Spectroscopy 2009 40(12):1858-66. [Google Scholar]
[28]. Tay FR, Pashley DH, Rueggeberg FA, Loushine RJ, Weller RN, Calcium phosphate phase transformation produced by the interaction of the Portland cement component of white mineral trioxide aggregate with a phosphate-containing fluid Journal of Endodontics 2007 33(11):1347-51. [Google Scholar]
[29]. Gallego D, Higuita N, Garcia F, Ferrell N, Hansford DJ, Bioactive coatings on Portland cement substrates: Surface precipitation of apatite-like crystals Materials Science and Engineering: C 2008 28(3):347-52. [Google Scholar]
[30]. Gandolfi MG, Taddei P, Tinti A, Dorigo EDS, Rossi PL, Prati C, Kinetics of apatite formation on a calcium-silicate cement for root-end filling during ageing in physiological-like phosphate solutions Clinical Oral Investigations 2010 14(6):659-68. [Google Scholar]
[31]. Camilleri J, Characterization of hydration products of mineral trioxide aggregate Int Endod J 2008 41(5):408-17. [Google Scholar]
[32]. Kamali S, Moranville M, Leclercq S, Material and environmental parameter effects on the leaching of cement pastes: experiments and modelling Cement and Concrete Research 2008 38(4):575-85. [Google Scholar]
[33]. Gandolfi MG, Ciapetti G, Taddei P, Perut F, Tinti A, Cardoso MV, Apatite formation on bioactive calcium-silicate cements for dentistry affects surface topography and human marrow stromal cells proliferation Dental Materials 2010 26(10):974-92. [Google Scholar]
[34]. Martin RL, Monticelli F, Brackett WW, Loushine RJ, Rockman RA, Ferrari M, Sealing properties of mineral trioxide aggregate orthograde apical plugs and root fillings in an in vitro apexification model Journal of Endodontics 2007 33(3):272-75. [Google Scholar]
[35]. Goldberg M, Pradelle-Plasse N, Tran X, Colon P, Laurent P, Aubut V, Emerging trends in (bio) material researches. Biocompatibility or cytotoxic effects of dental composites 2009 Oxford, UKCoxmoor Publishing:181-203. [Google Scholar]
[36]. Han L, Okiji T, Uptake of calcium and silicon released from calcium silicate–based endodontic materials into root canal dentine International endodontic journal 2011 44(12):1081-87. [Google Scholar]
[37]. Saghiri MA, Saghiri AM, In Memoriam: Dr. Hajar Afsar Lajevardi MD, MSc, MS (1955-2015) Iran J Pediatr 2017 27:e8093 [Google Scholar]