Introduction
An ideal face has well coordinated facial structures with the cranium, harmonious relationships of each jaw with their respective dentitions, soft tissue along with underlying hard tissue structures and balanced maxillomandibular relationships. Anterior-posterior skeletal jaw relationships depend on a range of combinations of maxillary and mandibular prognathism or retrognathism [1]. Jaw relationships has been classified into Class I, II, and III with Class I having straight profile; Class II having convex profile resulting from maxillary skeletal excess and/or mandibular skeletal deficiency; and Class III possessing concave profile which may be the outcome of maxillary skeletal deficiency and/or mandibular skeletal excess [2]. Abnormal stomatognathic functions sleep disorders due to mandibular deficiency, impaired dentofacial aesthetics and psychosocial problems result from abnormal sagittal jaw relationships [3].
We know that skeletal dysplasia is caused by genetics and environmental factors and differentiation of contribution of these two factors to skeletal anomalies is difficult [4]. Recent advances in genetic research assist in determining the contributions of genes in the development of craniofacial characteristics and associated anomalies. Determination of hereditary factors help in distinguishing the environmental factors and carry out a more targeted intervention [4].
Primarily, heritability was proved with family [5] and twin studies [6]. Studies have found a stronger genetic component for skeletal pattern than for dental characteristics [7], for vertical parameters than anteroposterior and for mandibular shape than mandibular size [8]. Probability of mandibular prognathism was found to be six times higher in monozygotic than dizygotic twins [9]. Studies have shown the genetic impact in cases with hypoplastic maxilla [10] and hypoplastic mandibles [11].
Racial predominance of sagittal Class III jaw relationship with prognathic mandible is found in Chinese and Malaysian populations while Indian populations show a relatively lower prevalence, as compared to other races [12]. Although twin based study method provided valuable information, its result might be statistically less significant [13].
Allelic association has been proved better than twin study from reports evaluating genetic influence on temporomandibular disorders [14]. Recently SNP’s are most frequently searched as they affect protein expression and functioning and bring about a phenotypic change [15].
Presently genetic loci 1p22.1, 1q32.2, 3q26.2, 11q22, 12q13.13, 12q23, 1p36, 6q25, 19p13.2, 14q24.3-31.2, 4p16.1, 1p22.3 and 1q32.2 have been reported to be linked with mandibular prognathism [16-20]. Moreover, studies have found that genes FGF23 [21], GHR [22], EPB41 [23], MATN1 [24], MYO1H [25] and DUSP6 [26] might be causal variants of Class III malocclusion. SNP’s in CYP19A1 gene, which encodes enzyme aromatase, have been found to influence sagittal jaw growth during puberty [27].
MSX1 gene is located on chromosome 4p16 and is expressed in cranial neural crest cells, thus influencing the development of nasal processes, maxilla and mandible [28-30]. MSX1 gene studies on mice have proved its role in maxillomandibular development [31,32]. MSX1 is expressed in the jaw bones prenatally, postnatally and even during aging [33]. MSX1 expression in early postnatal sagittal suture [34] and localization of Adelaide-type craniosynostosis to 4p16 [35] strengthens its role in sagittal jaw relationships. Mutation in MSX1 results in teeth agenesis [36] and syndromes involving cleft lip and palate [37,38]. MSX1 controls bone metabolism and growth [39], differentiation of cartilage [40] and muscle [41]. Significant association of MSX1 with dentofacial region including jaws and dentition lead to the selection of this gene as the candidate for exploring its role in inter jaw relationship. Although a lot of work has been done to correlate the genetic effect on malocclusion but role of MSX1 gene on malocclusion, yet to be properly characterized. Objectives of present study were to find the association of MSX1 gene with all skeletal sagittal malocclusions and adding to the gene pool responsible for the skeletal anomalies, which will help in orthodontic treatment planning in the future.
Materials and Methods
Case Selection
This cross-sectional study was conducted on 133 subjects at Faculty of Dental Sciences, Banaras Hindu University, Uttar Pardesh, India, between August 2014 and November 2015. Ethical clearance was obtained from the Ethical Committee of the Institute of Medical Sciences, Banaras Hindu University. Subjects were selected by purposive sampling method within this time period.
The case subjects (Class II division 1 = 41, Class I division 2 = 20, Class III = 37) were recruited from the orthodontic outpatient department and the control subjects (Class I = 35) were medical and dental students of university.
Based on case history, only the patients belonging to North India were taken. Further screening was done based on cephalometric examination (ANB angle and wits appraisal), facial profile and intraoral examinations. Cross-examination of subjects was performed to avoid error and biasing. The case subjects who diagnosed with skeletal Class II and Class III maxillomandibular relationships, having full permanent dentition from second molars to incisors in all four quadrants without any prior dental/orthodontic treatment were included. The criteria for the control subjects were similar, except that they were skeletal Class I profile (Normal ANB angle and wits appraisal) and Class I molar relationship with good alignment of all teeth (normal occlusion instead of malocclusion). Written informed consents were obtained from all study participants.
Sample Collection and DNA Isolation
Peripheral venous blood was collected in EDTA vials, which were used for all the further experimental works. DNA was isolated from blood using phenol-chloroform protocol described by Barker in 1998 [42]. About 3-5 ml of heparinised blood was taken in polypropylene tube with 15 ml of autoclaved 0.9% NaCl. These were mixed well for 5 minutes and then centrifuged at 5000 rpm for 5 minutes at room temperature. Supernatant was discarded without disrupting the pellet. Pellet was mixed with 15 ml (3-4 times volume of the blood) of haemolytic solution-A (Sucrose = 109.5 gm in 985 ml mQ, 1M MgCl2 = 5 ml, Triton X100 = 10 ml). Again after performing the established procedure, the precipitated DNA was transferred to a 1.5 ml microcentrifuge tube (Eppendorf tube) and washed two times with 1 ml of 70% ethanol. Pellet after drying in 37°C incubator was dissolved in 100-150 μl of TE. DNA was stored at 4°C. Each DNA sample was checked for purity by quantifying optical density ratio against 260/280 nm ultraviolet light absorbance in a spectrophotometer (NanoDrop 2000, Thermo Scientific) and samples with values greater than 1.7 were selected for quality testing by gel electrophoresis [Table/Fig-1].
PCR product of exon 1 in agarose gel viewed through ultraviolet light.
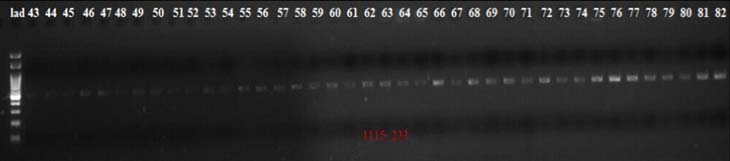
PCR and gene sequencing: MSX1 gene was amplified by PCR using genomic DNA as template with two sets of primers covering 2 exons; 1Pf 5’GCTGGCCAGTGCTGC3’, 1Pr 5’ACGGGGTCCTCTCGGGCTTC3’, 2Pf 5’ACTTGGCGGCACTCAATATC3’ and 2Pr 5’AAGCTATGCAGGAGACATGG3’.
The PCR was done in a reaction mixture of 25 μl for 35 cycles for exon1 and exon2 using PCR conditions in ABI Veriti 96 well thermal cycler machine (Applied Biosystems, CA, USA). Amplified products were eluted from agarose gel using GeneJet gel extraction kit (Thermo Fisher Scientific Inc., USA) [Table/Fig-2].
PCR product of exon 2 in agarose gel viewed through ultraviolet light.
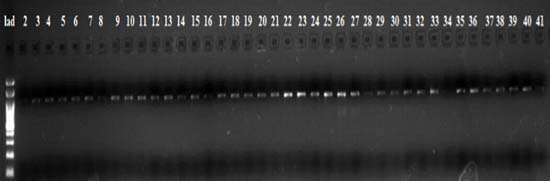
Genotyping was done in 3730XL DNA analyser (Applied Biosystems, USA). SNP’s were identified using multiple sequence alignment of MSX1 gene using Clustal X2.1 software [Table/Fig-3,4].
Multiple sequence alignment for MSX1 exon 1 for rs34165410 using Clustal X 2.1 software.
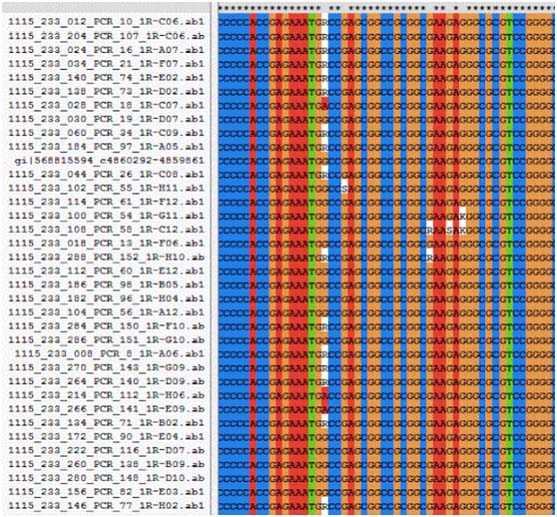
Multiple sequence alignment for MSX1 exon 2 for rs8670 using Clustal X2.1 software.
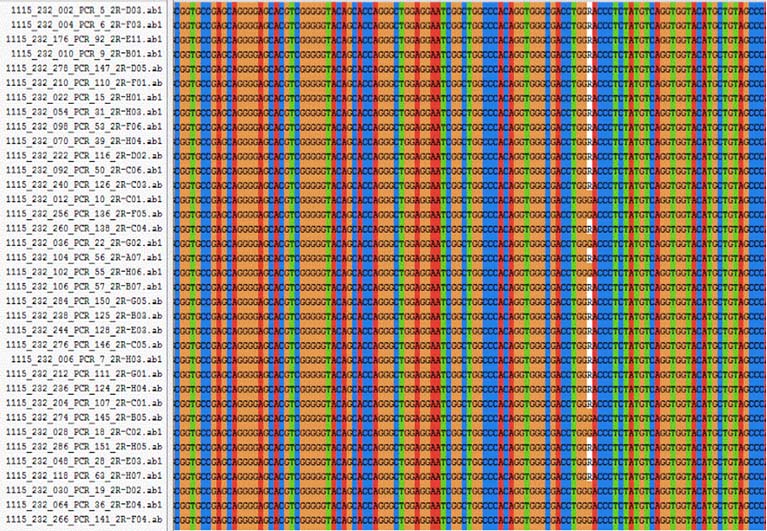
Statistical Analysis
Statistical analysis was performed using Chi square test to compare genotype differences between case and control groups [Table/Fig-5]. Statistical significance was taken at p<0.05. Effect of non-synonymous SNP’s on the structure, stability and activity of corresponding protein was predicted using Predict SNP software (Loschmidt laboratories, Czech Republic).
Case control association analysis for the variants identified.
For variant 1 (g.4861745C>G) |
g.4861745C>G | Case (class II Div 1) | Control (class I) |
CC | 36 | 32 |
CG | 5 | 3 |
GG | 0 | 0 |
X2p-value | 0.020.9 | ReferenceReference |
g.4861745C>G | Case (class II Div 2) | Control (class I) |
CC | 14 | 32 |
CG | 6 | 3 |
GG | 0 | 0 |
X2p-value | 2.840.09 | ReferenceReference |
g.4861745C>G | Case (class III) | Control (class I) |
CC | 29 | 32 |
CG | 8 | 3 |
GG | 0 | 0 |
X2p-value | 1.50.23 | ReferenceReference |
For variant 2 (g. 4861974 C>T) |
g.4861974 C>T | Case (class II Div 1) | Control (class I) |
CC | 30 | 25 |
CT | 10 | 8 |
TT | 1 | 2 |
X2p-value | 0.0080.9 | ReferenceReference |
g.4861974 C>T | Case (class II Div 2) | Control (class I) |
CC | 13 | 25 |
CT | 6 | 8 |
TT | 1 | 2 |
X2p-value | 0.040.85 | ReferenceReference |
g.4861974 C>T | Case (class III) | Control (class I) |
CC | 22 | 25 |
CT | 13 | 8 |
TT | 2 | 2 |
X2p-value | 0.70.43 | ReferenceReference |
For variant 3 (g.4861753A>C) |
g.4861753A>C | Case (class II Div 1) | Control (class I) |
AA | 40 | 34 |
AC | 1 | 1 |
CC | 0 | 0 |
p-value (Fisher’s exact test) | 0.7 | Reference |
g.4861753A>C | Case (class II Div 2) | Control (class I) |
AA | 19 | 34 |
AC | 1 | 1 |
CC | 0 | 0 |
p-value (Fisher’s-exact test) | 0.6 | Reference |
For variant 4 (g.4861721C>T) |
g.4861721C>T | Case (class II Div 2) | Control (class I) |
CC | 19 | 35 |
CT | 1 | 0 |
TT | 0 | 0 |
p-value (Fisher’s exact test) | 0.4 | Reference |
For variant 5 (g.4861609G>A) |
g.4861609G>A | Case (class II Div 1) | Control (class I) |
GG | 39 | 26 |
GA | 2 | 9 |
AA | 0 | 0 |
X2p-value | 5.0460.025 | ReferenceReference |
Odd’s Ratio (GG vs GA)95% Confidence Interval | 6.751.3485 to 33.7879 | Reference |
g.4861609G>A | Case (class II Div 2) | Control (class I) |
GG | 16 | 26 |
GA | 4 | 9 |
AA | 0 | 0 |
X2p-value | 0.220.9 | ReferenceReference |
g.4861609G>A | Case (class III) | Control (class I) |
GG | 32 | 26 |
GA | 4 | 9 |
AA | 1 | 0 |
X2p-value | 1.020.31 | ReferenceReference |
For variant 6 (g. 4861712C>G) |
g.4861712C>G | Case (class II Div 1) | Control (class I) |
CC | 39 | 34 |
CG | 2 | 1 |
GG | 0 | 0 |
p-value (Fisher’s-exact test) | 0.9 | Reference |
For variant 7 (g.4861912C>G) |
g.4861912C>G | Case (class II Div 1) | Control (class I) |
CC | 40 | 35 |
CG | 1 | 0 |
GG | 0 | 0 |
p-value (Fisher’s-exact test) | 0.54 | Reference |
For variant 8 (g. 4864876C>T) |
g.4864876C>T | Case (class II Div 1) | Control (class I) |
CC | 33 | 22 |
CT | 7 | 11 |
TT | 1 | 2 |
X2p-value | 2.120.14 | ReferenceReference |
g.4864876C>T | Case (class II Div 2) | Control (class I) |
CC | 13 | 22 |
CT | 5 | 11 |
TT | 2 | 2 |
X2p-value | 0.020.9 | ReferenceReference |
g.4864876C>T | Case (class III) | Control (class I) |
CC | 25 | 22 |
CT | 10 | 11 |
TT | 2 | 2 |
X2p-value | 0.030.9 | ReferenceReference |
For variant 9 (g. 4864938C>T) |
g.4864938C>T | Case (class II Div 1) | Control (class I) |
CC | 40 | 35 |
CT | 1 | 0 |
TT | 0 | 0 |
p-value (Fisher’s-exact test) | 0.54 | Reference |
g.4864938C>T | Case (class II Div 2) | Control (class I) |
CC | 19 | 35 |
CT | 1 | 0 |
TT | 0 | 0 |
p-value (Fisher’s-exact test) | 0.4 | Reference |
g.4864938C>T | Case (class III) | Control (class I) |
CC | 36 | 35 |
CT | 1 | 0 |
TT | 0 | 0 |
p-value (Fisher’s-exact test) | 0.5 | Reference |
Results
A total of 133 subjects with 35 Class I, 41 Class II Div 1, 20 Class II Div 2 and 37 class III patients were enrolled in this study [Table/Fig-6].
Distribution of patients in different classes of malocclusion.
Age Gr-oup(ye-ars) | Class I(P1-P35) | Class II Div 1(P36-P76) | Class II Div 2(P77-P96) | Class III(P97-P133) | Total No. of individuals |
---|
10-21 | | M | F | | M | F | | M | F | | M | F | | M | F |
4 | 3 | 1 | 25 | 8 | 17 | 13 | 4 | 9 | 20 | 10 | 10 | 62 | 25 | 37 |
21-32 | 29 | 22 | 7 | 15 | 4 | 11 | 7 | 3 | 4 | 13 | 8 | 5 | 64 | 36 | 28 |
32-43 | 2 | 2 | 0 | 0 | 0 | 0 | 0 | 0 | 0 | 2 | 2 | 0 | 4 | 4 | 0 |
43-54 | 0 | 0 | 0 | 1 | 0 | 1 | 0 | 0 | 0 | 2 | 2 | 0 | 3 | 2 | 1 |
| 35 | 27 | 8 | 41 | 12 | 29 | 20 | 7 | 13 | 37 | 22 | 15 | 133 | 67 | 66 |
* P = Patient
In exon 1; 5 reported substitutions and 2 novel substitutions and in exon 2; 2 reported substitutions were found [Table/Fig-7]. One synonymous variant (g.4861974C>T), 5 non-synonymous missense variant (g.4861745C>G, g.4861753A>C, g.4861721C>T, g.4861712C>G, g.4861912C>G) and 1 non-coding variant (g.4861609G>A) in 5’ UTR position were observed in exon1. The g.4861974C>T was observed in 43 subjects; 37 being heterozygote for the wild type and six homozygote for the rare allele. Even though twenty-two subjects had g.4861745C>Gmissense variant, g.4861753A>Cand g.4861712C>G variants were observed in 3 subjects each, g.4861721C>Tand g.4861912C>G variants were observed in only 1 case each, all heterozygous for the wild type allele. The non-coding variant (g.4861609G>A) was observed in 20 subjects, 19 heterozygote for the wild type allele and 1 homozygote for the rare allele.
Distribution of patients based on genetic variation.
Genomic position | Patient Id | Aminoacid change | Database status |
---|
Class I | Class II Div 1 | Class II Div 2 | Class III |
---|
Exon1 |
4861745C>G | P14, P32, P35 | P37, P57, P61, P68, P74 | P77, P78, P86, P89, P91, P95 | P105, P107, P109, P112, P121, P127, P129, P132 | A40G | rs36059701 |
4861974C>T | P1, P2, P3, P13, P16, P20, P22(TT), P24, P25(TT), P31, | P40(TT), P43, P44, P45, P46, P48, P64, P65, P66, P67, P75 | P80, P82(TT), P83, P84, P85, P89, P95 | P97, P99, P100, P101, P103, P106, P109, P110, P114, P119, P123, P124(TT), P126, P128(TT), P132 | CDS | rs34165410 |
4861753A>C | P21 | P39 | P84 | | M43L | rs565664559HGMD198263 |
4861721C>T | | | P78 | | A32V | reported |
4861609G>A | P5, P6, P19, P23, P24, P27, P29, P33, P35, | P36, P60 | P79, P86, P90, P91 | P98, P102, P112, P117, P125(TT), | 5’UTR | rs186861426 |
4861712C>G | P7, | P56, P60 | | | A29G | Novel polymorphic |
4861912C>G | | P54 | | | L96V | Novel Disease causing |
Exon2 |
4864876C>T | P4, P5, P6, P14, P15 (TT), P19, P23, P24, P27, P29, P32, P33, P35 (TT), | P36, P37, P49, P57, P61, P68, P70 (TT), P74, | P77, P78, P86 (TT), P89, P90, P91 (TT), P95 | P98, P102, P105, P107, P109, P112 (TT), P117, P121, P125 (TT), P127, P129, P132, | 3’UTR | rs8670 |
4864938C>T | | P62 | P92 | P104, | 3’UTR | rs1095 |
In exon 2, two non-coding variants (g.4864876C>T and g.4864938C>T) in 3’UTR position were found. The g.4864876C>T variantwas found in 40 subjects, 33 heterozygous for the wild type allele and 7 homozygous for the rare allele. The g.4864938C>T variant was observed in 3 subjects, all heterozygous for the wild type allele [Table/Fig-8].
Representative sequence electropherograms showing g.4861974C>T, g.4861745C>G, g.4861753A>C, g.4861712C>G, g.4861721C>T, g.4861912C>G, g.4861609G>A substitutions in exon1 and g.4864876C>T, g.4864938C>T substitutions in exon2 of MSX1 gene.
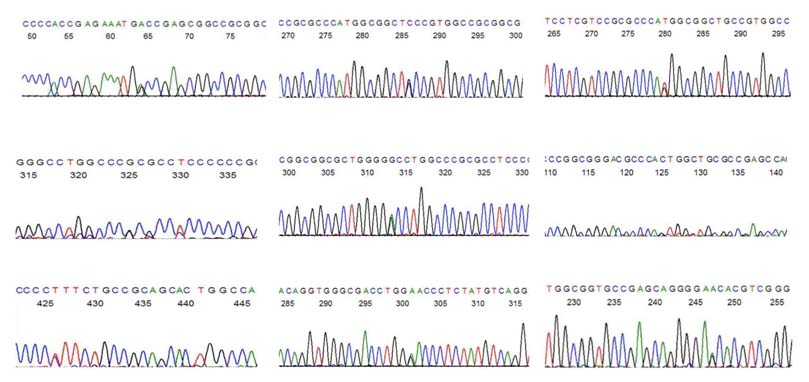
Statistical analysis revealed significant difference between Class II and Class I subjects with respect to g.4861609G>A variant (p<0.05). Odd’s ratio provided 6.75 times stronger association of this variant with class I subjects as compared to class II subjects [Table/Fig-5].
Further, analysis of non-synonymous variants using Predict SNP software was not found to have any deleterious effect on the MSX1 protein structure [Table/Fig-9].
Prediction of effect of non-synonymous SNP’s using Predict SNP software.
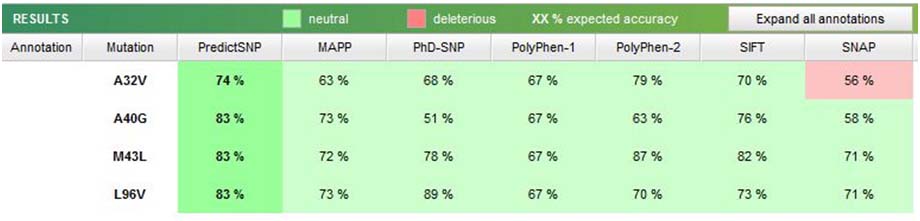
Discussion
Genetics is becoming an essential aid in enhancing diagnostic armamentarium and will be dominating our treatment priorities/decisions. Understanding of molecular genetics is vital to explain the underlying pathogenic mechanisms of human malformations [4]. Concept of aetiological heterogeneity complicates the understanding of genetic association [43]. Chromosomal aberrations, transpositions, deletions, or additions are usual causes of abnormalities in first branchial arch derivatives [4]. Point mutations affect single nucleotide base by transition or transversion. Elimination or retention of mutations by natural selection produces variations in phenotype [43].
SNP genotyping of EDA and XEDAR genes revealed significant association with Class I crowding in Hong Kong population [13]. Class II malocclusion was shown to have association with ACTN3 R577X genotype by Zebrick B et al., CYP19A1 gene SNP’s rs2470144 and rs2445761 were reported to be associated with average differences in annual sagittal jaw growth in males [44]. In our study, it was intended to find association of different sagittal jaw relationships with MSX1 gene [27].
MSX1 is a homeoboxgene that was found to have an association with craniofacial and jaw development [34,43,45]. Homeobox genes are master genes of craniofacial region; control induction, patterning, molecular interactions, and apoptosis during development. They produce transcription factors, which control the expression of other genes [43]. Pleiotropically expressing MSX1 will magnify its harmful effects with any change in its nucleotide sequence. MSX1 mutations have been associated with orofacial clefts [46] and ectodermal dysplasias having tooth agenesis and nail malformation [47].
SNP (g.4861609G>A, rs186861426) having significant association was found in 5’ UTR on exon1 of MSX1 gene. This non coding variant in 5’ UTR position is listed in the pubmed database but there is no frequency data for this population [48]. In the present study, g.4861609G>A variant was found in two Class II division 1 cases and nine Class I cases and showed significant association (p-value=0.02). ‘A’ allele seems to be protective against Class II division 1 malocclusion (Odd’s ratio (GG Vs GA) = 6.75). It means that individuals having ‘GA’ genotype have ≈ 7 times low risk for developing Class II division 1 malocclusion as compared to those having ‘GG’ genotype.
The UTRs are sections of mRNA next to the start and the end codons, due to their potential to bind to some miRNAs; are able to interfere with mRNA translation efficiency, stability, localization, and hence protein reproduction [49]. A 5’ upstream region of murine MSX1 has many enhancer components comprising NFκB- binding sites and one MSX1 consensus binding site [50]. These regulations in mice may also have similar MSX1 regulations in humans affecting its expression in multiple ways. Thus, the SNP in 5′UTR might have affected the regulatory factors and ultimately interfered with the gene expression and functioning.
The localization of MSX1 to distal of first branchial arch with its expression in developing anterior region influencing anterior palate and incisor development [51]. Postnatal expression in growing mandibular basal bone may be the association factor of MSX1 gene to skeletal jaw relationships [52]. MSX1-Tg mice with increased MSX1 expression had accentuated mandibular curve, rounded skull and limited facial outgrowth than WT ones while msx1−/− mice had lost mandibular curvature [45]. This indicated MSX1 driven bone growth direction. With applications of these facts to humans, it was suggested that deprival of the MSX1 expression gradient would cause a shift of craniofacial form from various skeletal head form e.g., dolichocephalic to a normocephalic or brachycephalic type [39].
Though SNP’s exist normally in the genome of every person [53]. Some of these SNP’s may contribute risk for multifactorial traits including malocclusion or may be protective as in the present study where individuals bearing minor allele A at chromosome 4 position 4861609 might be less susceptible to development of Class II division 1 malocclusion than others who do not have it.
Although synonymous or silent SNPs alter mRNA splicing and stability, protein structure, folding and function yet non synonymous SNPs and SNPs within regulatory regions have a greater probability to affect gene function relative to their synonymous SNP counterparts [54].
Non synonymous SNPs (g.4861745C>G, g.4861753A>C, g.4861721C>T, g.4861912C>G) using Predict SNP software were not found to have a deleterious effect. This might be due to neutral amino acid substituting other non polar amino acids and hence helix was unaffected as a result of undisturbed bonds [Table/Fig-9].
In the present study, two novel SNP’s (g.4861712C>G and g.4861912C>G) were found with g.4861712C>G in two Class II division 1 cases (p-value=0.9) and one control subject whereas g.4861912C>G was found in one Class II division 1 case (p-value=0.54) and both were not associated with malocclusion.
In the present study, g.4861745C>G variant always co-segregated with g.4864876C>T variant showing 100% linkage disequilibrium between the two variants. Significance of this is needed to be investigated further [Table/Fig-10].
Total linkage disequilibrium of g.4861745C>G with g.4864876C>T and frequency in different classes.
Genomic position | Patient ID | Class I | Class II Div 1 | Class II Div 2 | Class III |
---|
4861745C>G 4864876C>T | P14, P32, P35, P37, P57, P61, P68, P74, P77, P78, P86, P89, P91, P95, P105, P107, P109, P112, P121, P127, P129, P132 | 3 | 5 | 6 | 8 |
Results obtained in the present study, highlighted the MSX1 gene significance in the developmental process of skeletal sagittal jaw relationships. Two novel SNP’s obtained in present study appears to have contribution in development of jaws. As the associated SNP is already reported in other populations, conducting similar study in other populations with larger sample size using this SNP genotyping would be required to corroborate these findings. Moreover, in vitro investigation is required to be done by overexpressing this SNP in human embryonic kidney 293T cells to elucidate the biological impacts of this SNP.
Future prospects of this study and similar and more extensive studies are possibilities of extraction of exact genetic information from the biological parents and/or the newborns and determination of likelihood of skeletal anomalies that may occur in the offspring and thereby take appropriate correctional measures earliest as possible.
Conclusion
The present unique study is an attempt to find genetic association with all the three Classes of skeletal malocclusion in sagittal relation, carried out in Indian population. MSX1 plays important role in maxillomandibular development. The study unfolds an important relationship between MSX1 gene and Class II division 1 malocclusion and Class I normal skeletal relationships.
The role of human MSX1 extended in the gene pool information responsible for the skeletal anomalies related to development of abnormal upper and lower jaws. Similar studies with larger sample size and in varying populations are required to be conducted to establish this finding. Its predicted role should be validated by in vitro investigation involving overexpressing the candidate SNP’s in living cells and realizing the phenotypic change.
* P = Patient
[1]. Dale JG, Dale HC, Interceptive guidance of occlusion with emphasis on diagnosis. In: Graber, LW, Vanarsdall Jr, RL, Vig, KWL. (Eds.) Orthodontics: Current principles and techniques 2011 5th edPAMosby:423-76. [Google Scholar]
[2]. Ackerman JL, Nguyen T, Proffit WR, The decision making process in orthodontics. In: Graber, LW, Vanarsdall, RL Jr, Vig, KWL. (Eds.) Orthodontics: Current principles and techniques 2011 5th edPAMosby:01-58. [Google Scholar]
[3]. Proffit W, Fields H, Sarver D, Orthodontic diagnosis: the problem oriented approach. In: Proffit, W, Fields, H, Sarver, D. (Eds.) Contemporary orthodontics 2013 4th edSt LouisMosby:150-219. [Google Scholar]
[4]. Cakan DG, Ulkur F, Taner T, The genetic basis of facial skeletal characteristics and its relation with orthodontics Eur J dent 2012 6:340-45. [Google Scholar]
[5]. Cruz RM, Kreiger H, Ferreira R, Mah J, Hartsfield JJr, Oliveira S, Major gene and multifactorial inheritance of mandibular prognathism Am J Med Genet A 2008 146A:71-77. [Google Scholar]
[6]. Schulze C, Wiese W, On the heredity of prognathism Fortschr Kiefer Orthop 1965 126:213-29. [Google Scholar]
[7]. Lundström A, Nature vs nurture in dentofacial variation Eur J Orthod 1984 6:77-91. [Google Scholar]
[8]. Manfredi C, Martina R, Grossi GB, Giuliani M, Heritability of 39 orthodontic cephalometric parameters on MZ, DZ twins and MN-paired singletons Am J Orthod Dentofacial Orthop 1997 111:44-51. [Google Scholar]
[9]. Baker C, Similarity of malocclusion in families Int J Orthod 1924 10:459-62. [Google Scholar]
[10]. Schoenebeck JJ, Hutchinson SA, Byers A, Beale HC, Carrington B, Faden DL, Variation of BMP3 contributes to dog breed skull diversity PLoS Genet 2012 8:e1002849 [Google Scholar]
[11]. Gutierrez SJ, Gomez M, Rey JA, Ochoa M, Gutierrez SM, Prieto JC, Polymorphisms of the noggin gene and mandibular micrognathia: A first approximation Acta Odontol Latinoam 2010 23:13-19. [Google Scholar]
[12]. Hardy DK, Cubas YP, Orellana MF, Prevalence of angle class III malocclusion: A systematic review and meta-analysis Open J Epidemiol 2012 2:75-82. [Google Scholar]
[13]. Ting TY, Wong RW, Rabie AB, Analysis of genetic polymorphisms in skeletal Class I crowding Am J Orthod Dentofacial Orthop 2011 140:e9-15. [Google Scholar]
[14]. Slade G, Diatchenko L, Ohrbach R, Maixner W, Orthodontic treatment, genetic factors and risk of temporomandibular disorder NIH Public Access 2008 :146 [Google Scholar]
[15]. Wang Z, Moult J, SNPs, protein structure, and disease Hum Mutat 2001 17:263-70. [Google Scholar]
[16]. Yamaguchi T, Park SB, Narita A, Maki K, Inoue I, Genome-wide linkage analysis of mandibular prognathism in Korean and Japanese patients J Dent Res 2005 84:255-59. [Google Scholar]
[17]. Frazier-Bowers S, Rincon-Rodriguez R, Zhou J, Alexander K, Lange E, Evidence of linkage in a Hispanic cohort with a Class III dentofacial phenotype J Dent Res 2009 88:56-60. [Google Scholar]
[18]. Li Q, Li X, Zhang F, Chen F, The identification of a novel locus for mandibular prognathism in the hanchinese population J Dent Res 2011 90:53-57. [Google Scholar]
[19]. Li Q, Zhang F, Li X, Chen F, Genome scan for locus involved in mandibular prognathism in pedigrees from China PLoS One 2010 5:e12678 [Google Scholar]
[20]. Ikuno K, Kajii TS, Oka A, Inoko H, Ishikawa H, Iida J, Microsatellite genome-wide association study for mandibular prognathism Am J Orthod Dentofacial Orthop 2014 145:757-62. [Google Scholar]
[21]. Chen F, Li Q, Gu M, Li X, Yu J, Zhang YB, Identification of a mutation in FGF23 involved in mandibular prognathism Sci Rep 2015 5:11250 [Google Scholar]
[22]. Sasaki Y, Satoh K, Hayasaki H, Fukumoto S, Fujiwara T, Nonaka K, The P561T polymorphism of the growth hormone receptor gene has an inhibitory effect on mandibular growth in young children Eur J Orthod 2009 31:536-41. [Google Scholar]
[23]. Xue F, Wong R, Rabie AB, Identification of SNP markers on 1p36 and association analysis of EPB41 with mandibular prognathism in a Chinese population Arch Oral Biol 2010 55:867-72. [Google Scholar]
[24]. Jang JY, Park EK, Ryoo HM, Shin HI, Kim TH, Jang JS, Polymorphisms in the Matrilin-1 gene and risk of mandibular prognathism in Koreans J Dent Res 2010 89:1203-07. [Google Scholar]
[25]. Tassopoulou-Fishell M, Deeley K, Harvey EM, Sciote J, Vieira AR, Genetic variation in myosin 1H contributes to mandibular prognathism Am J Orthod Dentofacial Orthop 2012 141:51-59. [Google Scholar]
[26]. Nikopensius T, Saag M, Jagomägi T, Annilo T, Kals M, Kivistik PA, A missense mutation in DUSP6 is associated with Class III malocclusion J Dent Res 2013 92:893-98. [Google Scholar]
[27]. He S, Hartsfield JKJr, Guo Y, Cao Y, Wang S, Chen S, Association between CYP19A1 genotype and pubertal sagittal jaw growth Am J Orthod Dentofacial Orthop 2012 142:662-70. [Google Scholar]
[28]. Robert B, Lyons G, Simandl BK, Kuroiwa A, Buckingham M, The apical ectodermal ridge regulates Hox-7 and Hox-8 gene expression in developing chick limb buds Genes Dev 1991 5:2363-74. [Google Scholar]
[29]. Nishikawa K, Nakanishi T, Aoki C, Hattori T, Takahashi K, Taniguchi S, Differential expression of homeobox-containing genes Msx-1 and Msx-2 and homeoprotein Msx-2 expression during chick craniofacial development Mol Biol Int 1994 32:763-71. [Google Scholar]
[30]. Trıbulo C, Aybar MJ, Nguyen VH, Mullins MC, Mayor R, Regulation of Msx genes by a Bmp gradient is essential for neural crest specification Development 2003 130:6441-52. [Google Scholar]
[31]. Satokata I, Maas R, Msx1 deficient mice exhibit cleft palate and abnormalities of craniofacial and tooth development Nat Genet 1994 6:348-56. [Google Scholar]
[32]. Satokata I, Ma L, Ohshima H, Bei M, Woo I, Nishizawa K, Msx2 deficiency in mice causes pleiotropic defects in bone growth and ectodermal organ formation Nat Genet 2000 24:391-95. [Google Scholar]
[33]. Petit S, Meary F, Pibouin L, Jeanny JC, Fernandes I, Poliard A, Autoregulatory loop of Msx1 expression involving its antisense transcripts J Cell Physiol 2009 220:303-10. [Google Scholar]
[34]. Orestes-Cardoso SM, Nefussi JR, Hotton D, Mesbah M, Orestes-Cardoso MD, Robert B, Postnatal Msx1 expression pattern in craniofacial, axial, and appendicular skeleton of transgenic mice from the first week until the second year Dev Dyn 2001 221:01-13. [Google Scholar]
[35]. Hollway GE, Phillips HA, Adès LC, Haan EA, Mulley JC, Localization of craniosynostosis Adelaide type to 4p16 Hum Mol Genet 1995 4:681-83. [Google Scholar]
[36]. Boeira Junior BR, Echeverrigaray S, Polymorphism in the MSX1 gene in a family with upper lateral incisor agenesis Arch Oral Biol 2012 57:1423-28. [Google Scholar]
[37]. Cobourne MT, Familial human hypodontia — is it all in the genes? Br Dent J 2007 203:203-08. [Google Scholar]
[38]. Otero L, Gutierrez S, Chaves M, Vargas C, Bermudez L, Association of MSX1 With nonsyndromic cleft lip and palate in a Colombian population Cleft Palate Craniofac J 2007 44:653-56. [Google Scholar]
[39]. Nassif A, Senussi I, Meary F, Loiodice S, Hotton D, Robert B, Msx1 role in craniofacial bone morphogenesis Bone 2014 66:96-104. [Google Scholar]
[40]. Mina M, Gluhak J, Upholt WB, Kollar EJ, Rogers B, Experimental analysis of Msx-1 and Msx-2 gene expression during chick mandibular morphogenesis Dev Dyn 1995 202:195-214. [Google Scholar]
[41]. Odelberg SJ, Kollhoff A, Keating MT, Dedifferentiation of mammalian myotubes induced by msx1 Cell 2000 103:1099-1109. [Google Scholar]
[42]. Barker. Phenol-Chloroform Isoamyl Alcohol (PCI) DNA Extraction;1998 [Google Scholar]
[43]. Mossey PA, The heritability of malocclusion: Part 1-Genetics, principles and terminology Br J Orthod 1999 26:103-13. [Google Scholar]
[44]. Zebrick B, Teeramongkolgul T, Nicot R, Horton MJ, Raoul G, Ferri J, ACTN3 R577X genotypes associate with class II and deepbite malocclusions Am J Orthod Dentofacial Orthop 2014 146:603-11. [Google Scholar]
[45]. Orestes-Cardoso S, Nefussi JR, Lezot F, Oboeuf M, Pereira M, Mesbah M, Msx1 is a regulator of bone formation during development and postnatal growth: in vivo investigations in a transgenic mouse model Connect Tissue Res 2002 43:153-60. [Google Scholar]
[46]. Tongkobpetch S, Siriwan P, Shotelersuk V, MSX1 mutations contribute to nonsyndromic cleft lip in a Thai population J Hum Genet 2006 51:671-76. [Google Scholar]
[47]. De Muynck S, Schollen E, Matthijs G, Verdonck A, Devriendt K, Carels C, A novel MSX1 mutation in hypodontia Am J Med Genet A 2004 128A:401-03. [Google Scholar]
[48]. Database of Single Nucleotide Polymorphisms (dbSNP). Bethesda (MD). National Center for Biotechnology Information, National Library of Medicine. dbSNP accession: {rs186861426} Available from: http://www.ncbi.nlm.nih.gov/snp/snp_viewtable.cgi?method_id=13256 [Google Scholar]
[49]. Pesole G, Mignone F, Gissi C, Grillo G, Licciulli F, Liuni S, Structural and functional features of eukaryotic mRNA untranslated regions Gene 2001 276:73-81. [Google Scholar]
[50]. Mehra-Chaudhary R, Matsui H, Raghow R, Msx3 protein recruits histone deacetylase to down-regulate the Msx1 promoter Biochem J 2001 353:13-22. [Google Scholar]
[51]. Welsh IC, O’Brien TP, Signaling intergration in the rugae zone directs sequential SHH signaling center formation during rostral outgrowth of the palate Dev Biol 2009 336:53-67. [Google Scholar]
[52]. Berdal A, Molla M, Hotton D, Aioub M, Lezot F, Nefussi JR, Differential impact of MSX1 and MSX2 homeogenes on mouse maxillofacial skeleton Cells Tissues Organs 2009 189:126-32. [Google Scholar]
[53]. Carlson DS, Evolving concepts of heredity and genetics in orthodontics Am J Orthod Dentofacial Orthop 2015 148:922-38. [Google Scholar]
[54]. Hunt R, Sauna ZE, Ambudkar SV, Gottesman MM, Kimchi-Sarfaty C, Silent (synonymous) SNPs: should we care about them? Methods Mol Biol 2009 578:23-39. [Google Scholar]