Introduction
Spinal dysraphism occurs due to failure of fusion of parts along dorsal aspect of midline structures lying along spinal axis from skin to vertebrae and spinal cord. Congenital spinal anomalies may be minimal and asymptomatic like spinal bifida occulta, or severe with marked neurological deficits like Arnold-Chiari malformation or caudal regression syndrome. Magnetic Resonance Imaging (MRI) is the modality of choice to diagnose mild to severe spinal dysraphism.
Aim
To diagnose type and extent of clinically suspected spinal anomalies by MRI scan and to compare various sequences for identifying neural tissue and fatty tissue in anomalies.
Materials and Methods
Fifty paediatric patients referred with clinical suspicion of spinal anomalies for MRI scan to radiodiagnosis department and diagnosed as having spinal dysraphism on 1.5 Tesla MRI Scan, were included in this observational analytic study. Various MRI sequences were taken in multiple planes. MRI findings of spinal dysraphism were compared with detailed clinical examination or surgical findings. Osseous anomalies like spina bifida occulta were confirmed by radiographs or CT scan.
Results
Out of 50 patients, type II Arnold-Chiari Malformation (34%), Spina Bifida Occulta (22%) and Diastematomyelia (18%) were common anomalies. MRI findings were well correlated with surgical findings in 20 operated cases. Nerve roots with/ without neural placode in thecal sac/outpouching were detected in combination of 3D HASTE myelographic sequence with SE/ TSE T1W sequence in 24 cases; which was significantly high as compared to combinations of SE/TSE T1W sequence with TSE T2W, with STIR and with Single Shot Myelographic sequence {p-value 0.002, < 0.001 and 0.008 respectively}. Fatty component was present in dysraphism in five cases, commonly as isolated anomaly; which was detected by combination of STIR and SE/TSE T1W sequences in all five cases.
Conclusion
Paediatric spinal dysraphism and associated malformations are accurately diagnosed on MRI scan. MR myelographic 3D-HASTE and STIR sequences should be a part of protocol to evaluate spinal dysraphism.
Introduction
Column of 33 vertebrae makes central axial skeleton of human body; which are separated by 23 intervertebral discs starting below the C2 vertebral body upto S1 vertebral body. They are associated with 31 pairs of spinal nerves – mainly supplying limbs [1].
Embryological Basis
Spinal dysraphism is defined as developmental abnormalities of the dorsal median region in embryo, variably involving ectoderm, mesoderm and endoderm. Dysraphism signifies incomplete fusion or malformation of a seam or junction [2]. Spinal dysraphisms are congenital defects in vertebral arch/arches; which may have associated malformations of congenital fibrous band, lipoma, congenital cyst or outpouching, nerve roots or spinal cord.
Normally, spine and spinal cord develops between 2-6 gestational weeks; which can be divided in three stages [2-5]. First stage of gastrulation occurs in 2-3 weeks. On 14-15 gestational days, primitive streak grows caudally in dorsal midline and development of mesoderm creates trilaminar disc. Caudal regression of primitive streak forms midline notochordal process; which cavitates to notocordal canal. Flattening and curving of notochordal canal forms definitive notochord by 17th day. Defective gastrulation stage is presumed to cause split cord malformation, neuroenteric cyst or anterior meningocele [5].
Second stage of primary neurulation happens during 3-4 weeks, starting by notochord interacting with ectoderm and forming neural plate. On 18th day, neural plate starts bending and folds into neural tube. Neural tube closure starts at fourth somite level and extends cranio-caudally in zipper-like fashion [4,5]. Rostral and caudal neurospores close last between 24 to 27 days. Meeting of ectoderm folds separates neural tube from cutaneous ectoderm by dysjunction and forms tubular primordium. Placode is part of non-neurulated embryonic neural tissue frozen at the stage of neural plate. Placode may be terminal (at cord’s caudal end) or segmental (at intermediate level along cord). Placode is usually observed in all open spinal dysraphism and several severe closed spinal dysraphism. In primary neurulation, spinal cord is formed only upto second sacral segment. Disturbance in primary neurulation probably causes meningocele or meningomyelocele. Premature dysjunction is thought to cause dorsal type lipoma; while incomplete dysjunction is presumed origin of dorsal dermal sinus [5]. Third stage of secondary neurulation occurs during 5-6 weeks. Caudal pleuripotent cell mass forms solid medullary cord; which cavitates by retrograde differentiation and forms additional secondary neural tube. This tube forms spine and spinal cord caudal to conus medullaris tip and filum terminale. Defective secondary neurulation possibly lead to caudal or transitional lipoma, thickened filum or myelocystocele [5].
Spectrum of Spinal Dysraphism
Spinal dysraphism includes all forms of spina bifida. Closed Spinal Dysraphism (CSD) is covered by skin and is also known as spina bifida occulta; while Open Spinal Dysraphism (OSD) is not covered by skin and is also called spina bifida cystica/aperta [2,4,6].
Spectrum of closed spinal dysraphism occurs with two different subsets: (A) those with subcutaneous mass in lower back like meningocele, lipomeningomyelocele, myelocystocele, and lipomyeloschisis, and (B) those without mass like spina bifida occulta, tight filum terminale, persistent terminal ventricle, dorsal dermal sinus, filar lipoma, intradural lipoma, neuroenteric cyst, dorsal enteric fistula, diastematomyelia, caudal regression syndrome (caudal agenesis) and segmental spinal dysgenesis [4,6]. Spectrum of open spinal dysraphism is meningomyelocele, myelocele, hemimyelocele, hemimeningomyelocele and myeloschisis [4,6].
Role of Radiological Investigations
Plain radiograph is screening modality for bony spinal anomalies. Ultrasound is good modality for evaluation of spinal anomalies in antenatal period; but in young infants, it can be used only as screening modality as it is sensitive to detect dysraphism with cystic component; but is less sensitive to MRI in closed spinal dysraphism [7]. Good ultrasound study also depends on available acoustic window and operator’s skill. Computed Tomography (CT) scan with multi-planar reformations is excellent modality to diagnose vertebral anomalies and bony septum in diastematomyelia; but it has less soft tissue contrast and requires intrathecal injection of non-ionic iodinated contrast to evaluate thecal sac and its content [8]. Ultrasound and CT scan do not show small abnormalities of spinal cord and nerves and do not have good soft tissue contrast as MRI; which makes MRI initial investigation of choice [9]. Magnetic Resonance Imaging (MRI) scan is best modality to diagnose spinal dysraphism and is usually the only investigation required for diagnosis and pre-surgical planning for spinal dysraphism and associated syndrome [10].
Proper protocol is essential for a complex modality like MRI scan; otherwise abnormality/lesion may not be seen [11]. MRI is time consuming and patient has to remain still in scanner gantry for 30-45 minutes for spine study. Many paediatric patients require sedation or short general anaesthesia to get good quality MRI scan without motion artifacts. Long time of MRI scan has potential hazard of complication during general anaesthesia. So, comparison of combinations of sequences was explored to find best pair of sequences for making diagnosis. Most useful sequences should be taken initially for getting essential information in short scan time, so that if patient starts moving during scan and further anaesthesia is risky, most useful part of study is obtained and diagnosis can be made.
The main objective of study was to diagnose clinically suspected congenital spinal anomalies and associated malformations and to confirm their type and extent by MRI scan. Other objective was to compare various sequences and weighting of images for identifying neural tissue in anomalies with confidence. These sequences and image weighting were also compared for finding fatty tissue in anomalies.
Materials and Methods
This observational study was done at Department of Radio-diagnosis, Pramukhswami Medical College and Shree Krishna hospital, Karamsad, Dist. Anand, Gujarat, India, from September 2011 to August 2016.
Paediatric age group was considered as age of 1 day to 18 years for this research study. On PubMed and Google internet searches, no baseline similar study of comparison of different MRI sequences in spinal dysraphism was found; hence, comparison of different MRI sequence in this study was done as an exploratory study. Fifty paediatric patients, who had been referred with clinical suspicion of congenital spinal anomalies to department of radiodiagnosis for MRI scan and were diagnosed as having spinal dysraphism on MRI findings, as well as had follow up for comparison by surgical finding (for complex anomalies), plain radiograph or CT scan (for osseous anomalies) or detailed clinical examination (e.g., dorsal dermal sinus or cystic/fatty swelling) within three months of MRI scan, were included in the study. Post-surgical patients of spinal anomaly referred for MRI scan were excluded from the study. All patients that satisfied inclusion criteria and without any exclusion criteria were included. Research project was approved by Human Research Ethical Committee of the institution.
Informed written consent was taken from the parent or legal guardian before MRI scan. All the patients underwent MRI scan in 1.5 Tesla Siemens Symphony-Vision Maestro Class MRI scanner (Head Quarter: Erlangen, Germany). Heavy sedation or short general anaesthesia was required in 28 patients for doing MRI scan; out of which 20 were given midazolam and ketamine combination; while eight were given fentanyl and propofol combination. For MRI of spine, six element spinal array coil in gantry table and for MRI scan of spine in infant or MRI of brain in Arnold-Chiari Malformation, four channel head coil were used. As per our MRI protocol, all the three planes viz., sagittal, axial and coronal planes were acquired. In cervical spine, 3.0 mm thick images were taken in all the three planes. In thoracic or lumbar spine, 4.0 mm thick images were obtained in all three planes. About 5 mm thick images were obtained for MRI of brain. Main technical parameters of MRI of spine are tabulated [Table/Fig-1].
MRI protocol followed in this study.
Parameter → | Sequence and weighting | TF | FOV (mm) | Matrix (Pixel) | In Plane Resolution (mm x mm) | Number of Slices | TR (ms) | TE (ms) |
---|
Plane ↓ |
---|
Sagittal | TSE T2W | 23 | 320 | 256x256 | 1.3 x 1.3mm | 13 | 4000 | 115 |
TSE T1W | 7 | 320 | 256x256 | 1.3 x 1.3mm | 13 | 535 | 13 |
Single Shot Myelo. | 288 | 300 | 288x320 | 0.1 x 0.8mm | 1 | 8000 | 1200 |
Axial | TSE T2W | 15 | 200 | 205x256 | 0.8 x 0.8mm | 20-29 | 2400-3600 | 105 |
SE T1W | - | 200 | 205x256 | 0.8 x 0.8mm | 20-29 | 525-800 | 13 |
Coronal | TSE T1W | 7 | 320 | 205x256 | 1.5 x 1.3mm | 14 | 500 | 12 |
STIR (TI =150) | 7 | 320 | 205x256 | 1.5 x 1.3mm | 14 | 4000 | 52 |
Single Shot Myelo. | 288 | 320 | 256x256 | 0.1 x 0.8mm | 1 | 8000 | 1200 |
3-D Myelo. With Reforma tions | HASTE -with Fat Sat. | 236 | 320 | 256x256 | 0.8 x 0.8mm | 48-72 | 8000 | 275 |
The T1 weighted (T1W) images were taken by Spin Echo (SE) or Turbo Spin Echo (TSE) sequences; which are best for anatomical depiction. Pathologies are usually seen better on T2 Weighted (T2W) images taken by Turbo Spin Echo (TSE) sequence and Short Tau Inversion Recovery (STIR) fat suppression sequence. For Single Shot MR Myelography, 40 mm to 60 mm thick slabs were taken in sagittal and coronal planes. In 3 dimensional (3D) Myelographic acquisition by Half-Fourier Acquisition Single-shot Turbo-spin Echo (HASTE) with fat saturation sequence, 0.8 mm thin sagittal images were taken and were reformatted in oblique, coronal and/or curved planes of thickness between 15 mm to 60 mm.
CT scan of spine was done after taking informed consent from parent/gaurdian in four patients to confirm small bony abnormality and in one patient for spinal anomalies with Sprengel shoulder. These were done 64 detector row General Electric Optima CT 660 CT scanner (Head Quarter: Chicago, USA). CT protocol used is 1.25 mm thin acquisition using 120 kV, 330 mA with 1.0 to 1.2 pitch. For cervical spine, 3 mm thick axial images were reconstructed; while for thoraco-lumbar spine 4 mm thick axial images were reconstructed with 3 or 4 mm thick sagittal and coronal reconstruction.
MRI findings of open spinal dysraphism or closed spinal dysraphism with subcutaneous mass were confirmed by surgery or detailed clinical examination. Open spinal dysraphism were diagnosed by finding neural tissue/placode in meningomyelocele or by diagnosing Arnod-Chiari type II malformation (always associated with lumbar meningomyelocele). Lack of subcutaneous fat over lumbar cystic lesion was supportive finding. Occult bony spinal anomalies were confirmed by antero-posterior and lateral radiographs with additional help of CT scan in five patients.
Review of these MRI scans were done again at least after three months of MRI and observation of neural tissue (nerves/placode) and fatty tissue with confidence were tabulated by seeing four pairs of sequences viz., SE/TSE T1W and TSE T2W sequences, SE/TSE T1W and STIR sequences, SE/TSE T1W and Single Shot Myelographic sequences, SE/TSE T1W and 3D HASTE with Fat Saturation Myelographic sequences.
Statistical Analysis
Patient’s data as well as comparison of pair of sequences of different spinal dysraphism were tabulated. The images obtained were compared as four pairs of sequences viz., SE/TSE T1W and TSE T2W sequences, SE/TSE T1W and STIR sequences, SE/TSE T1W and Single Shot Myelographic sequences, SE/TSE T1W and 3D HASTE with fat saturation myelographic sequences in terms of characterization of nerves/nerve roots with or without placode as well as fatty tissue for the ease and confidence.
Neural tissue (nerves/placode) detection rate was calculated as proportion (in %) for observations made by four pairs of sequences. Statistical method used was test of difference between proportion to calculate probability (p) values of best neural tissue detection rate and other pairs of sequences; which were calculated by statistician using Stata software (Version 14.2). The p-value < 0.05 was considered statistically significant.
Results
In this study of MRI of spine of 50 paediatric patients; minimal age of patient was 7 days and maximum age was 15 years 3 months with 25 patients being male and 25 patients being female. Patient’s data of different spinal dysraphism were tabulated with their number, age and gender [Table/Fig-2].
Types of different paediatric spinal dysraphism found in study with frequency, mean age (with range) and gender.
No. | Anomaly | No. of Patients (with %) | Mean Age (Range) | Gender |
---|
Male | Female |
---|
1 | Arnold-Chiari Type II Malformation | 17(34%) | 6 Months (14 Days -16 Months) | 9 | 8 |
2 | Spina Bifida Occulta alone | 11(22%) | 13 Years (8-15 Years) | 5 | 6 |
3 | Diastematomyelia (1 with small dorsal dermal sinus) | 9(18%) | 10 Years (3 -15 Years) | 4 | 5 |
4 | Spina bifida with other bony deformities | 4(8%) | 8 Years (6-10 Years) | 1 | 3 |
5 | Dorsal Dermal Sinus | 4(8%) | 10 Years (3-15 Years) | 3 | 1 |
6 | Intraspinal Lipoma | 3(6%) | 7 Years (7 days- 14 Years) | 2 | 1 |
7 | Lipomeningocele | 1(2%) | 15 Years | - | 1 |
8 | Caudal Regression Syndrome | 1(2%) | 7 Years | 1 | - |
| TOTAL PATIENTS | 50 [100%] | 7 Years 1 Month &16Days (7 Days - 15 Years) | 25 [50%] | 25 [50%] |
Most common anomalies were type II Arnold-Chiari malformation with lumbar meningomyelocele (17 patients, 34%), spina bifida occulta (11 patients, 22%) and diastematomyelia (9 patients, 18%). Occult bony anomalies were found in 15 patients (30%). MRI observations were well correlated with surgical findings in 20 operated cases; which were lumbar meningomyelocele of Arnold-Chiari malformation (14 patients), cord tethering in diastematomyelia (3), dorsal dermal sinus with diastematomyelia (1), intraspinal lipoma (1) and lipomeningocele (1). Bony anomalies in 15 patients were confirmed by plain radiographs; among which five patients underwent CT scan for very small slit-like spina bifida occulta in cervical/thoracic regions (3 patients with 1 patient also having Sprengel shoulder), to differentiate thin bony spur from fibrous band in diastematomyelia (1 patient) and to confirm bony cortical pressure erosion in patient with intraspinal lipoma (1 patient). Rest 15 patients were correlating with detailed clinical examination.
Among these cases of type II Arnold-Chiari malformation and diastomatomyelia show abnormality related to neural tissue in 24 patients (48% of total patients) [Table/Fig-3]. Nerve roots with or without neural placode in thecal sac or outpouching were detected by 3D-MR Myelographic HASTE sequence with SE/TSE T1W sequence in all 24 cases having neural tissue in malformation (48% of total patients); which suggested this combination extremely accurate to diagnose neural tissue (100% identification). Neural tissue detection rate with combination of other three pair of sequences viz. SE/TSE T1W and TSE T2W Sequences, SE/TSE T1W and STIR sequences as well as SE/TSE T1W sequence with single shot myelographic sequences were 66.7%, 54.2% and 75%; which are significantly less as compared to combination of 3D-HASTE with SE/TSE T1W sequences having p-value of 0.002, <0.001 and 0.008 respectively. Presence of neural placode and nerves in dysraphism suggest more complex malformation, having guarded prognosis.
Comparison of different sequences paired with SE and TSE T1W images for detecting neural tissue in spinal dysraphism with ease and confidence with proportion of neural tissue detection rate and test of difference between proportion for significance.
Pair of images/ Diagnosis | Total patients | SE/TSE T1W and TSE T2W | SE/ TSE T1W and STiR | SE/TSE T1W and Single Shot MR Myelography | SE/TSE T1W and 3D HASTE MR myelography |
---|
Arnold-Chiari Malformation (type II)•Neural placode•Nerves/roots alone or with placode | 171017 | 811 | 79 | 813 | 1017 |
Diastematomyelia• Nerves/roots | 97 | 5 | 4 | 5 | 7 |
Neural Tissue Detection: Number of Cases | 17 + 7 = 24 | 11+ 5 = 16 | 9 + 4 = 13 | 13 + 5 = 18 | 17 + 7 = 24 |
Neural Tissue Detection Rate (Percent) | 100 % | 66.7% | 54.2 % | 75.0 % | 100 % |
Test of Difference Between Proportion | p-value Between | | 0.008 |
| < 0.001 |
0.002 |
Fatty lesions or fatty components were found in five patients (10 percent) [Table/Fig-3].
Fatty tissue is seen commonly in an isolated anomaly of transitional type of intraspinal lipoma (3 cases) or lipomeningomyelocele (1 case) and have more favourable prognosis. Prominent fatty filum terminale was also diagnosed in patient of caudal regression syndrome. The STIR sequence in combination with T1W images had shown fatty component in all the five cases [Table/Fig-4].
Comparison of different sequences paired with SE and TSE T1W images for detecting fatty tissue in spinal dysraphism.
Pair of images/ Diagnosis | Total patients | SE/TSE T1W and TSE T2W | SE/TSE T1W and STiR | SE/TSE T1W and Single Shot MR myelog- raphy | SE/TSE T1W and 3D HASTE MR Myelography |
---|
Intraspinal Lipoma(All transitional type) | 3 | 2 | 3 | 2 | 2 |
Lipomeningo- myelocele | 1 | 0 | 1 | 0 | 0 |
Caudal Regression Syndrome (1)• Fatty Filum (Filar Lipoma) | 1 | 1 | 1 | 0 | 0 |
Fatty Tissue Detection: No. of Cases | 5 [Total] | 3 | 5 (All) | 2 | 2 |
Clinical and radiological features of few complex spinal dysraphism like Arnold-Chiari Type II Malformation [Table/Fig-5], diastematomyelia with dorsal dermal sinus [Table/Fig-6], multiple spina bifida with Sprengel shoulder [Table/Fig-7], dorso-lumbar epidural lipoma with dural ectasia, multiple spina bifida and hemivertebrae [Table/Fig-8] are shown.
Images of Arnold-Chiari type II malformation. * † C/F = Clinical Features ‡ R/F = Radiological Findings † C/F: 14 days old girl having congenital lumbo-sacral swelling and big head size.‡ R/F: L3, L4, L5 and S1 Spina bifida with lumbar menigomyelocele having horizontally oriented nerve roots and neural placode with cerebellar tonsilar ectopia upto C5 level and mild hydro-cephalus.
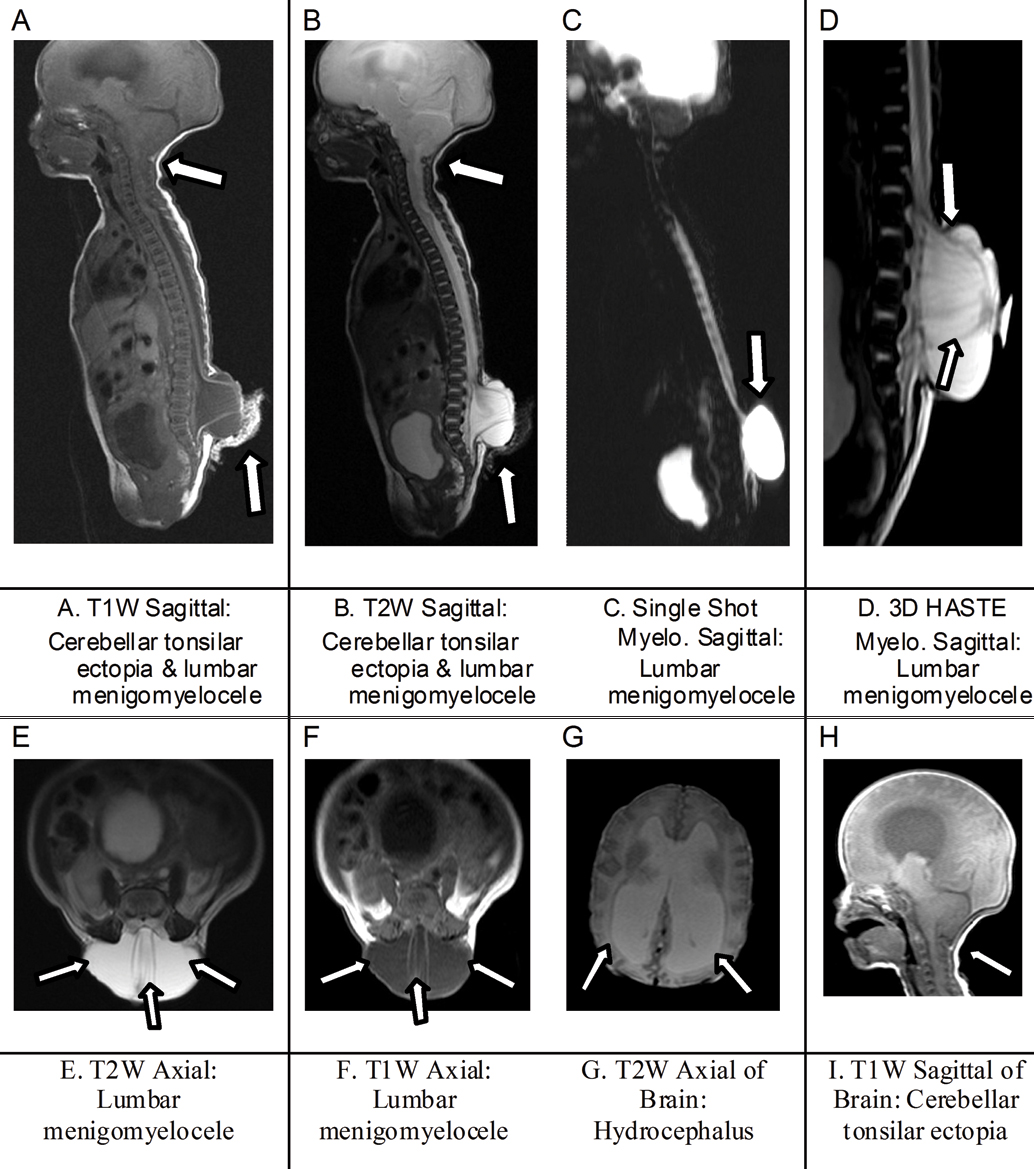
Diastematomyelia with small dorsal dermal sinus. C/F:10 Years old boy with history of small sinus in mid-lumbar region with mild lower limb weak-ness.‡ R/F: Bony spur at upper L3 level, tethered split hemi-cords with syrinx in upper lumbar region and small posterior dermal sinus at lower L3 level.
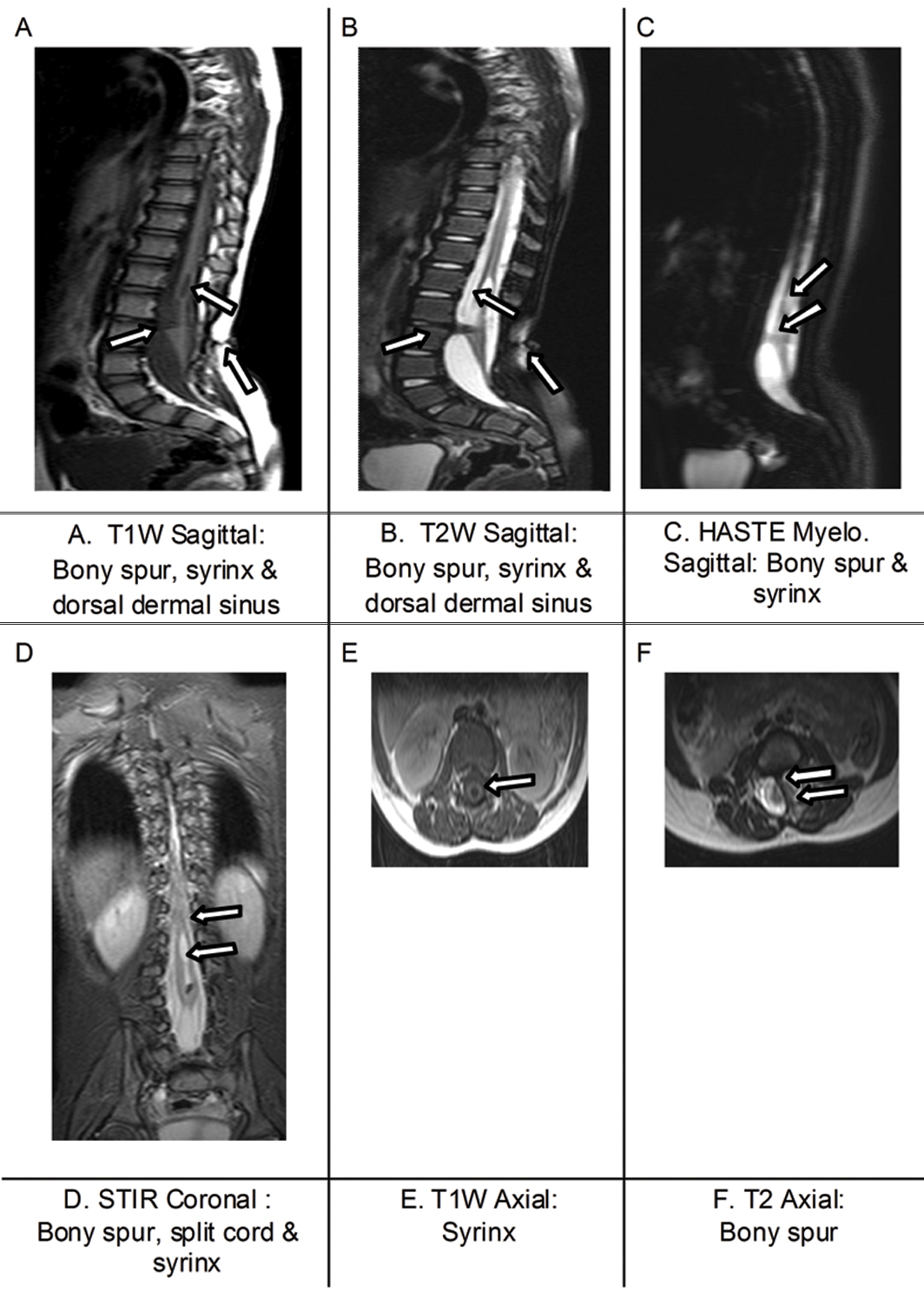
Multiple spina bifida with Sprengel shoulder deformity. † C/F:6 Years old girl with elevated shoulders and mild restriction of shoulder movements. ‡ R/F:Spina bifida of C5, C6, C7, T1 and T2 vertebrae with asymmetric omo-vertebral bones on both sides and winging of scapulae.
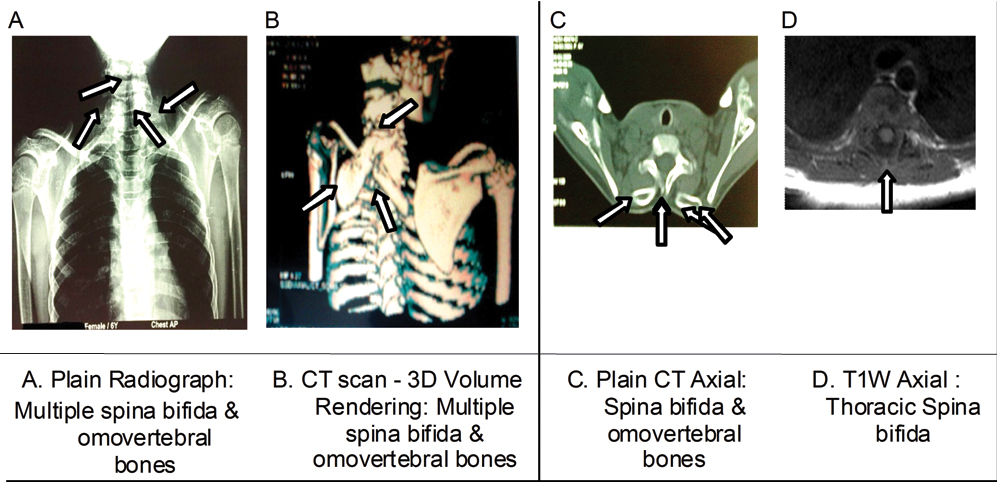
Dorso-lumbar epidural lipoma with mild dural ectasia, multiple spina bifida and hemivertebrae. C/F: 7 days old girl having history of dorso-lumbar congenital swelling and kyphoscoliosis.‡ R/F: Dorso-lumbar intraspinal lipoma, mildly dilated thecal sac with multiple spina bifida and hemivertebrae.
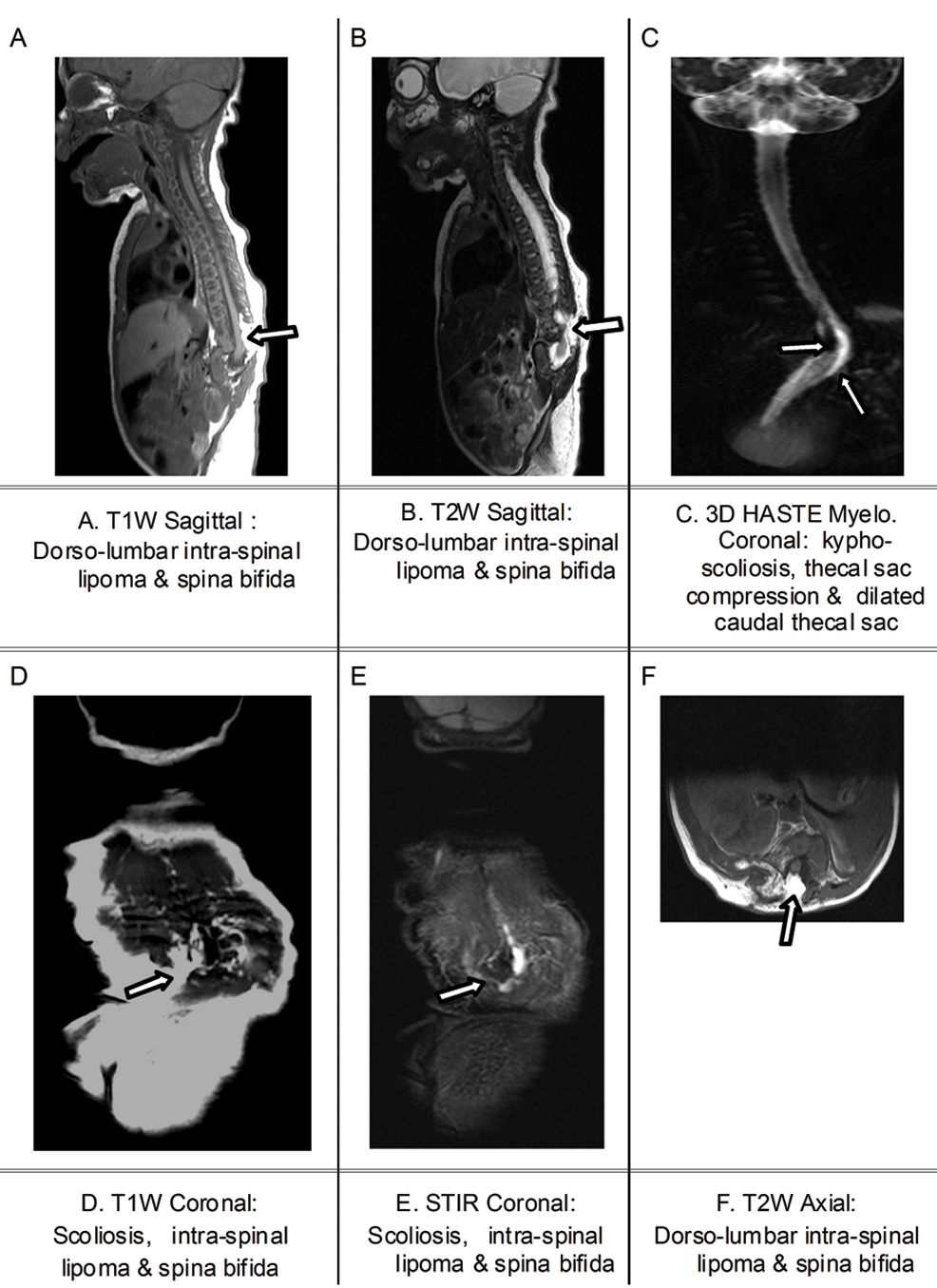
Discussion
Clinical features of spinal anomaly are hair tuft, hypertrichosis, Port-wine stain, visible swelling, haemangioma, dimpling, sinus tract in back, visibly short back, abnormal spinal curvature, deviation of gluteal furrow, human or faun tail, asymmetry of legs/feet, subcutaneous lipoma, sensory loss in limb/limbs, decreased movements in limb/ limbs, dribbling of urine, recurrent urinary tract infection [5,12]. Combination of two or more cutaneous midline lesions in back is the strongest marker of occult or closed spinal dysraphism [12].
Main objective of study was to evaluate clinically suspected spinal dysraphism by MRI scan; which were accurately diagnosed with their type and extent. MRI findings are well correlated on surgery (20 patients), radiographs/CT (15 patients with 5 having CT) and detailed clinical examination (15 patient). Ramacharya et al., in their study of 50 patients in India were reported nearly similar kinds of spinal dysraphism with range of presenting age of two months to 16 years as well as 26 female and 24 male [13]. Kumar R et al., in their study of 155 patients done in Northern India reported mean age of presentation of 5.7 years with 1.5:1 female to male ratio [14]. Meningomyelocele is seen in Arnold-Chiari malformation in all 17 cases; which constitutes 100% open spinal dysraphism and 34 % of all patients. Meningomyelocele was reported to constitute 98.8 % of open spinal dysraphism in west [3,4].
Another objective of study was to explore possibility of finding best sequence pair by comparing different sequence pairs to detect neural tissue and fatty tissue in different spinal anomalies. Similar study of MRI sequence comparisons for spinal dysraphism was not found in different internet searches. The only sequence comparison study in MRI of spine was done by Campi A et al., who had compared T2-weighted Fast SE, Conventional Echo (CSE) and STIR-FSE in 57 patients of MRI of cervical spine; in which they found STIR-FSE sequence better than other two sequences to find small demyelination plaques and recommended their inclusion in suspected demyelinating disease [15].
MR Three dimensional gradient steady state sequence- Constructive Interference with Steady State (CISS) with its reformations in multiple planes and at various angles is mainly used to assess intracranial ventricles and cisternal spaces. Even this 3D CISS sequences was investigated by various investigators like Ramli N et al., Hingwala D et al., and Azad R et al., in MRI of spine; who have found the 3D CISS sequence and its reformations useful even in spinal region [16-18].
Predisposing factors of spinal dysraphism are nutritional deficiency of folic acid and mutations in genes involving folic acid metabolism [19]. Recurrence risk after birth of an infant with isolated spina bifida is 3%-5% with recurrence in sibling may occur as spina bifida or other spinal anomaly. Chromosome abnormalities, single gene mutation, maternal diabetes mellitus, prenatal exposure to certain anti-convulsants given to mother are other causative factors; which have different recurrence risks [19]. Few Northern provinces in China have highest prevalence rates of 1 in 200 pregnancies for spina dysraphism; while european countries have lower prevalence rate of < 1 in 2,000 pregnancies. Overall estimated incidence of spinal dysraphism is 1-3/1000 live births [6,20]. In India, as in world over; steady decrease is observed in incidence of spinal dysraphism in the decade of 2001-2010 due to folic acid fortification in pregnancy, antenatal detection by ultrasound and elevated maternal Alpha Feto Protein (AFP) (found in upto 80% cases of spina bifida) as well as medical termination of pregnancy [20].
Limitation
Limitations are single institution study and not having any previous baseline study of similar type of sequence pair comparisons to determine sample size and robust study design. This study is done to explore the possibility of better choices of sequences in protocol, if study need to be done in short time. This kind of study should be done at multiple institutions to determine best sequence or pair of sequences for accurate tissue characterisation; especially for neural tissue identification, which affect the prognosis of spinal dysraphism.
Conclusion
Congenital spinal dysraphism and associated malformations can be accurately diagnosed on MRI in paediatric patients with their type and extent. The 3D-MR myelographic sequence with SE/FSE T1W images is very useful to demonstrate abnormalities of the thecal sac, nerve roots/placode in abnormal thecal outpouching. Fatty lesion or fatty component is easily seen on STIR sequence with SE/ FSE T1W images; which are commonly seen as isolated anomalies. MR myelographic 3D-HASTE sequence and STIR sequence should always be the part of protocol to evaluate clinically suspected spinal dysraphism.
[1]. Drake RL, Vogl AW, Mitchell Adam WM, Gray’s Anatomy for Students 2015 3rd international edPhiladelphiaElsevier Churchill Livingston:32-33.59-61 & 74-77 [Google Scholar]
[2]. Singh I, Human Embryology 2015 10th edJaypee Brothers Medical Publishers (P.) Ltd:58-70.141-52 [Google Scholar]
[3]. Rossi A, Cama A, Piatelli G, Ravegnani M, Biancheri R, Tortori-Donat Pi, Spinal Dysraphism: MR Imaging Rationale J. Neuroradiol 2004 31(1):3-24. [Google Scholar]
[4]. Stephanie LR, Mohanned I, Raybaud CA, Parmar HA, Congenital spine and spinal cord malformations- pictorial review AJR 2010 194:S26-S37. [Google Scholar]
[5]. Dominic NP Thompson, Spinal dysraphic anomalies, classification, presentation and management Paed and Child Health 2010 20(9):397-403. [Google Scholar]
[6]. Tortori-Donati P, Rossi A, Rishu B, Cama A, Magnetic resonance imaging of spinal dysraphism Top in Magn Reson Imaging 2001 12(6):375-409. [Google Scholar]
[7]. Dhingani DD, Boruah DK, Dutta HK, Gogoi RK, Ultrasonography and magnetic resonance imaging evaluation of pediatric spinal anomalies J Pediatr Neurosci 2016 11:206-12. [Google Scholar]
[8]. Kumaran SK, Chirtrarasan P, Role of helical CT and MRI in the evaluation of spinal dysraphism Int J Adv Med 2017 4:124-32. [Google Scholar]
[9]. Altman NR, Altman DH, MR imaging of spinal dysraphism AJNR 1987 8:533-38. [Google Scholar]
[10]. Khan AN, Naul LG, Turnbull I, Sabih D, Al-Okaili R. Imaging in spinal dysraphism and myelomeningocele: magenetic resonance imaging. Available from http://emedicine.medscape.com/article/413899-overview#a4 [Assessed on 6-10-2016] [Google Scholar]
[11]. Osborn AG, Hendrick RE, Emanuel K, Introduction to magnetic resonance imaging: a basic primer 1992 Oslo NorwayNycomed; March [Google Scholar]
[12]. Guggisberg D, Hadj-Rabia S, Viney C, Bodemer C, Brunelle F, Zerah M, Skin markers of occult spinal dysraphism in children Arch Dermatol 2004 140:1109-15. [Google Scholar]
[13]. Ramacharya Desai KS, Spinal dysraphism: MRI evaluation Int J Res Med Sci 2015 3(8):1937-41. [Google Scholar]
[14]. Kumar R, Singh SN, Spinal dysraphism: trends in northern India Pediatr Neurosurg 2003 38(3):133-45. [Google Scholar]
[15]. Campi A, Pontesilli S, Gerevini S, Scotti G, Comparison of MRI pulse sequences for investigation of lesions of the cervical spinal cord Neuroradiology 2000 42(9):669-75. [Google Scholar]
[16]. Ramli N, Cooper A, Jaspal T, High resolution CISS imaging of the spine British Journal of Radiology 2001 74:862-73. [Google Scholar]
[17]. Hingwala D, Chatterjee S, Chandrasekharan K, Bejoy T, Kapilamoorthy TR, Applications of 3D CISS sequence for problem solving in neuroimaging Indian J Radiol Imaging 2011 21(2):90-97. [Google Scholar]
[18]. Azad R, Azad S, Shukla AK, Arora P, Role of screening of whole spine with sagittal MRI with MR myelography in early detection and management of occult intrasacral meningocele Asian Journ of Neurosurg 2013 8(4):174-78. [Google Scholar]
[19]. Spina Bifida in Encyclopedia of Children’s health: available at http://www.healthofchildren.com/S/Spina-Bifida.html [Assessed on 17-6-2017] [Google Scholar]
[20]. Venkataramana NK, Spinal Dysraphism J Pediatr Neurosci 2011 6(suppl 1):S31-S40. [Google Scholar]