Introduction
Connective Tissue Growth Factor (CTGF/CCN2) is one of the six members of cysteine-rich, heparin-binding proteins, divreted as modular protein and recognised to play a major function in cell processes such as adhesion, migration, proliferation and differentiation as well as chondrogenesis, skeletogenesis, angiogenesis and wound healing. The capacity of CTGF to interact with different growth factors lends an important role during early and late development, especially in the anterior region of the embryo. CTGF Knockout (KO) mice have several craniofacial defects and bone miss shaped due to an impairment of the vascular system development during chondrogenesis.
Aim
The aim of the study was to establish an association between multiple modular functions of CTGF and the phenotype and cardiovascular functions in transgenic mouse.
Materials and Methods
Bicistronic cassette was constructed using pIRES expressing vector (Clontech, Palo Alto, CA). The construct harbours mouse cDNA in tandem with LacZ cDNA as a reporter gene under the control of Cytomegalovirus (CMV) promoter. The plasmid was linearised with NotI restriction enzyme, and 50 ng of linearised plasmid was injected into mouse pronucleus for the chimaera production. Immunohistochemical methods were used to assess the colocalisation renin and CTGF as well as morphology and rheology of the cardiovascular system.
Results
The chimeric mice were backcrossed against the wild-type C57BL/6 to generate hemizygous (F1) mouse. Most of the offsprings died as a result of respiratory distress and those that survived have low CTGF gene copy number, approximately 40 molecules per mouse genome. The copy number assessment on the dead pups showed 5×103 molecules per mouse genome explaining the threshold of the gene in terms of toxicity. Interestingly, the result of this cross showed 85% of the progenies to be positive deviating from Mendelian first law. All F2 progenies died excluding the possibility of establishing the CTGF transgenic mouse line, situation that compelled us to work at the level of hemizygosity. The histological characterisation of left ventricle shows cardiac hypertrophy together with decrease in body mass and alopecia, this compared to the wild type. The immunohistochemical staining of aorta root showed hyperplasia with increased expression and colocalisation of renin and CTGF demonstrating that CTGF may be involved in vascular tone control.
Conclusion
Genetic engineering is a noble avenue to investigate the function of new or existing genes. Our data have shown that CTGF transgenic mouse has cardiac and aorta root hypertrophy and abnormal renin accumulation in aorta root as compared to the wild-type animals. The transgenic animals developed alopecia and lean body mass adding two new functions on pre-existing CTGF multiple functions.
Introduction
CCN2 or CTGF is a matricellular protein of the CCN family of Extracellular Matrix (ECM)-associated heparin-binding proteins [1]. CTGF fulfils several crucial functions in biological arena including angiogenesis, cell adhesion, proliferation, migration, tissue and wound repair. CTGF is also involved in bone development and seriously implicated in different types of cancers and fibrotic function [1-3]. CTGF exerts its functions by binding to various cell surface receptors in a context-dependent manner including integrin receptors [4], cell surface heparan sulphate proteoglycans [5], Low-density lipoprotein receptor-related proteins [6] and TrkA [7]. In addition, CTGF also binds growth factors and ECM proteins. The N-terminal half of CTGF interacts with aggrecan [8], Thrombospondin Type 1 repeat domain interacts with Vascular Endothelial Growth Factor (VEGF) [9], and the C-terminal domain interacts with members of the Transforming Growth Factor-β (TGF-β) superfamily, fibronectin, perlecan, fibulin-1, slit and mucins [1,2]. CTGF is also known as an important role player in the developmental biology. Indeed, CTGF KO mice die at birth due to respiratory distress as a result of severe chondrodysplasia [10]. CTGF-null mice also show defects in angiogenesis, with impaired interaction between endothelial cells and pericytes and collagen IV deficiency in the endothelial basement membrane [2]. CTGF is also important for pancreatic beta cell development [11] and is critical for normal ovarian follicle development and ovulation [12]. CTGF plays an important role in wound repair and has been suggested to be involved in all pathologies dealing with fibrosis process [13]. Most interestingly, CTGF acts as a strong inducer of ECM proteins such as integrin receptors, fibronectin and type-I collagen [14] and acts in mediation with TGF-β1 in these processes. Besides fibrosis, CTGF expression correlates with many types of diseases such as cardiovascular diseases, arthritis, diabetic nephropathy and retinopathy [15].
In the light of all these multiple functions, CTGF may still have unknown functions that have not been biologically vetted, and genetic modified animals are the unique models of choice to elucidate new functions for known genes. To explore these functions, a transgenic mouse model harbouring the CTGF expression cassette under the control of ubiquitous promoter CMV was generated. We demonstrated a development of vascular complication indicating a topical dysregulation of CTGF expression inside this tissue.
Materials and Methods
The study was conducted in the Laboratory of Molecular and Diagnostics Personalized Therapeutic Unit, University of Hail School of Medicine, Hail, Kingdom of Saudi Arabia during span of over four years and has been approved by Ethical Committee of the School of Medicine, University of Hail.
Study Design
Vector construction: The pIRES 6.1, bicistronic vector was obtained from Clontech (Palo Alto, CA) and a plasmid βgeo-harboring LacZ obtained from Felipe Soriano Laboratory (Seattle, Washington State). The pIRES is a mammalian expression vector that allows high-level expression of two genes of interest from the same bicistronic mRNA transcript. The vector contains the Encephalomyocarditis Virus (ECMV) Internal Ribosome Entry Site (IRES) flanked by two Multiple Cloning Sites (MCS A and B), an arrangement that allows cap-independent translation of the gene cloned into MCS B (Rees S et al., 1996, Jackson RJ et al., 1990 and Jang SK et al., 1988) [16-18]. The pIRES plasmid utilises a partially disabled IRES sequence (Rees S et al., 1996) [16] that reduces the rate at which the gene cloned into MCS B is translated relative to that of MCS A. The constitutively active Cytomegalovirus Immediate Early Promoter (PCMV IE) drives the expression of the bicistronic transcript located between PCMV IE and MCS A and efficiently spliced out the following transcription. SV40 polyadenylation signals downstream of MCS B direct proper processing of the 3’ end of the mRNA, a condition that generates a stable transcript. Two bacteriophage promoters T7 and T3, respectively, located upstream and downstream flank the two multiples cloning sites MCS A and MCS B. The vector harbours a neomycin resistance gene (Neor) under the control of the SV40 enhancer/promoter destined for the selection of transfected cells. Appended at the 3’ end is a synthetic polyadenylation signal that will control for the stabilisation of Neor mRNA. The SV40 is endowed with an origin of replication that allows the SV40 T antigen replication inside the mammalian cells. Upstream the CMV promoter is ColE1 origin of replication and ampicillin resistance gene (Ampr) for the propagation and the selection in Escherichia coli and an f1 origin for the production of single-stranded DNA. The cassettes cloned into either MCS should start with methionine codons. The pIRES cassette thus engineered can be electroporated into the mammalian cells and was grown in G418 medium. To engineer the transgenic vector, a pair of primers (Fwd: 5’-GGTTTGATGATCTGTGACATGG-3’ and Rev: 5’-CTGCAGCCCGGGGGATCCCCTGAC-3’) were designed in βgeo plasmid, little bit upstream the methionine start point of lacZ cDNA. The forward primer was flanked by NheI, and the reverse primer was flanked with SalI which ultimately is religazable into XhoI site on pIRES 6.1 leading to the abrogation of both sites (SalI and XhoI) on the construct. The amplified Polymerase Chain Reaction (PCR) product of 3 Kb was subcloned onto pIRES 6.1. The resulting vector of approximately 9 Kb was digested with SalI and NotI. The CTGF cDNA subcloning onto the pIRES vector was proceeded as follows: Mouse plasmid cDNA was obtained from (OriGene Technologies, Rockville, MD). Forward and reverse primers (Fwd: 5’-GCATCCTCCTACCGCGTCCCGATC-3’, Rev: 5’-GAGTTCGTGTCCCTTACTTCCTGG-3’) for mouse and, respectively, flanked with SalI and NotI. We amplified the mouse CTGF cDNA and subcloned it onto the pIRES and βgeo plasmid [Table/Fig-1]. This gives approximately 10 Kb plasmid. The resulting plasmid was transformed into E. coli bacteria, propagated and purified with Qiagen kit (QIA Amp Qiagen, Hilden, Germany). This plasmid was linearised with NotI and 50 ng was injected into mouse pronucleus to generate the chimaera. These chimeric of two months were backcrossed C57B6 wild type to propagate the line.
TBV bicistronic targeting vector made from pIRES plasmid, a mammalian expression vector that allows high-level expression of two genes of interest from the same bicistronic mRNA transcript. The vector contains the Encephalomyocarditis Virus (ECMV) Internal Ribosome Entry Site (IRES) flanked by two Multiple Cloning Sites (MCS A and B), an arrangement that allows cap-independent translation of the gene cloned into MCS B. The plasmid utilises a partially disabled IRES sequence that reduces the rate at which the gene cloned into MCS B is translated relative to that of MCS A. The constitutively active Cytomegalovirus Immediate Early Promoter (PCMV IE) drives the expression of the bicistronic transcript located between PCMV IE and MCS A and efficiently spliced out the following transcription. The SV40 origin allows for replication in mammalian cells expressing the SV40 T antigen. The vector also contains an ampicillin resistance gene (Ampr), and a ColE1 origin of replication for selection and propagation in Escherichia coli, and an F1 origin for single-stranded DNA production. Genes cloned into either MCS must contain ATG start codons.
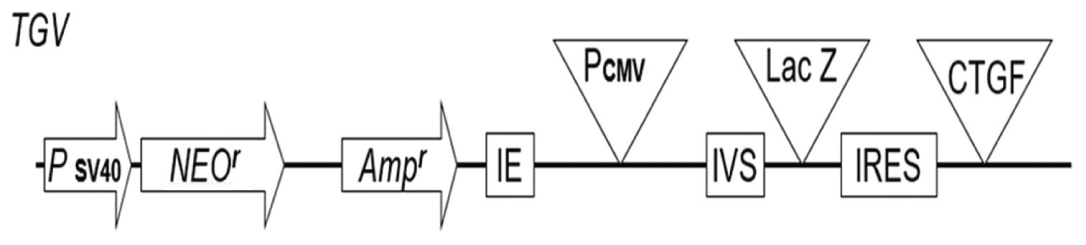
Animal Breeding
Four chimeric CTGF transgenic mice were backcrossed to four C57BL/6 mice. Most of the progenies resulting from this backcross die at birth, and those that survive have a low CTGF gene copy number as low as 40 ng per mouse genome. An analysis on cadaver showed gene copy number as high as 5000 molecules of CTGF per mouse genome, which means that high CTGF gene copy number is lethal to the animals, some of the pups having green or red eyes colour. All progenies resulting from this intercross die as resultant of gene toxicity and this challenging our capability of establishing this transgenic line compelling us to conduct all experiments at the state of hemizygosity.
Determination of Heart Shape by Histochemical Studies
Left ventricle frozen sections were cut at 10 μm onto gelatin-coated slides and air dried. Soak the slides in Eosin Y, Sigma for 30 seconds. Rinse two times one min each in 95% ETOH and two times one min each in 100% EtOH. Soak again the slides in 50:50 in EtOH/xylene for one minute and two times in xylene for one minute each. During dehydration periods, the previous liquid was let to drain off for few seconds but not completely so as to transfer a minimum of the previous liquid into the next liquid.
CTGF Immunohistochemistry Staining of the Aorta Root from Transgenic and Control Mice
CTGF immunochemically was conducted in aorta root as previously described [19]. Aorta root and connective tissues were deep frozen quickly at −70°C and cut with cryostat at 6 μm thick. The tissue slices were let thaw in room temperature, air dried and equilibrate for 20 minutes in phosphate-buffered saline (pH 7.4). The tissues were blocked for 15 minutes in normal 5% goat serum. The slices were incubated for 60 minutes in room temperature with anti-CTGF polyclonal antibody (Fibro Gen, Inc., South San Francisco, California). The sections were subsequently treated with a biotinylated secondary anti-rabbit antibody (1:400; Vector Laboratories, Burlingame, California). The red colour was revealed using an aminoethyl carbazole kit (Zymed Laboratories, Inc., South San Francisco). Between each step, sections were washed intermittently for five minutes with phosphate-buffered saline and haematoxylin was used to counterstain the slides and mounted with glycerin-gelatin medium.
Renin Immunohistochemistry Staining of the Aorta Root from Transgenic and Control Mice
Animals (adults, eight to nine-months-old) were anaesthetised with ether. The aorta root was removed fixed in Bouin’s fixative or 10% formalin. The fixed tissue was dehydrated and embedded in paraffin, and 5 μm sections were cut. Tissues were processed for immunohistochemistry and stained with haematoxylin and eosin to assess morphology, with Periodic Acid-Schiff (PAS) reagent to assess basement membrane integrity and with Masson’s trichrome stain to assess collagen deposition.
Immunohistochemistry - Bouin’s fixed kidney tissue sections (5 μm thick) were deparaffinised in xylenes and graded alcohols, and immunostaining was performed for renin (using a 1:10,000 dilution of goat anti-rat-renin polyclonal antibody (obtained from Santa Cruz Biotechnology, Santa Cruz, CA).
Statistical Analysis
Body weight gain between transgenic mice and littermate control were analysed using statistical software package SPSS 18.0 (SPSS Inc., Chicago, IL, USA). Data were presented as a mean ± standard deviation. Descriptive data between the two groups were generated in forms of mean.
Results
[Table/Fig-1] depicts the targeting bicistronic vector. The vector harbours two cDNA in tandem: β-geo LacZ as a reporter gene and CTGF cDNA as a transgene. Both cDNAs are separated by IRES to obviate the production of fusion genes in the mouse genome during genome integration. The β-geo was appended upstream to the CTGF cDNA to allow easy PCR genotyping of the pups during breeding. [Table/Fig-2] represents the average body weight of transgenic mice as compared to control mice. Transgenic mice (39.75 gm) versus control mice (67.62 gm), standard deviation: Transgenic mice (21.52) versus control mice (19.69) with 95% confidence interval for mean. F factor (7.302) and (p<0.01**) values were accessed using ANOVA program. These results were highly significant as ascertained by p value (p<0.01**).
Body weight gain of the transgenic mice compared to the control littermate.
*WT=Wild type, TG= Transgenic
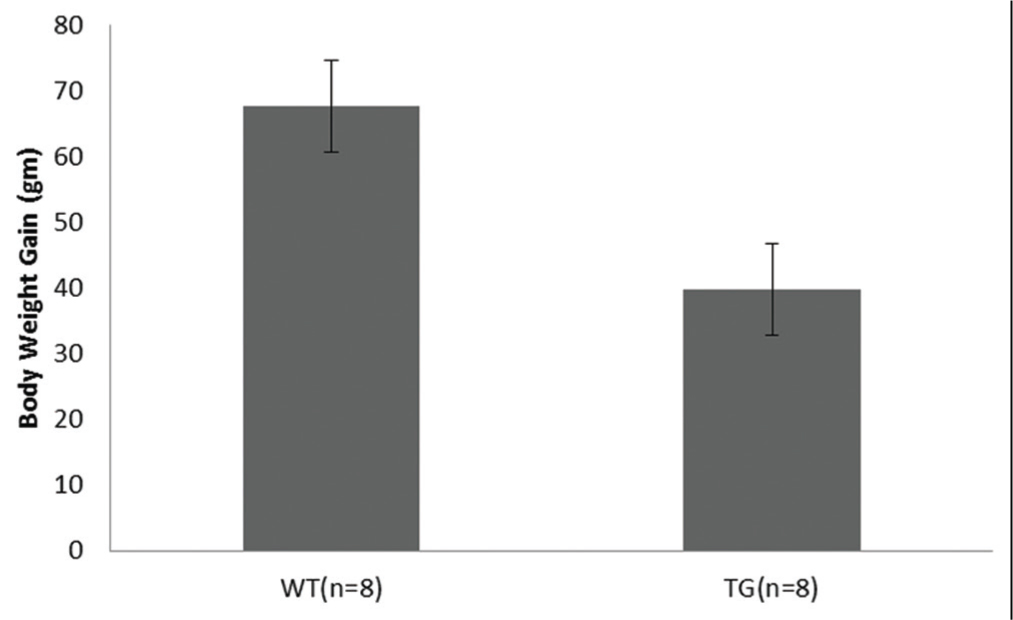
[Table/Fig-3] shows the picture of the transgenic mice at the age of 8-9 months. The transgenic mice lost weight and developed alopecia. [Table/Fig-4] shows the histological characterisation of left ventricle of CMV-CTGF transgenic mouse with an overt cardiac hypertrophy as compared to control mouse. [Table/Fig-5,6] show aorta root hyperplasia with colocalisation of CTGF and renin in aorta root as revealed with renin and CTGF antibodies in the transgenic mouse as compared to control and with an marked increase of renin and CTGF in transgenic mouse.
Transgenic mice picture at the age of 8-9 months. Note the fur coat of the transgenic mice is characterised hair loss.
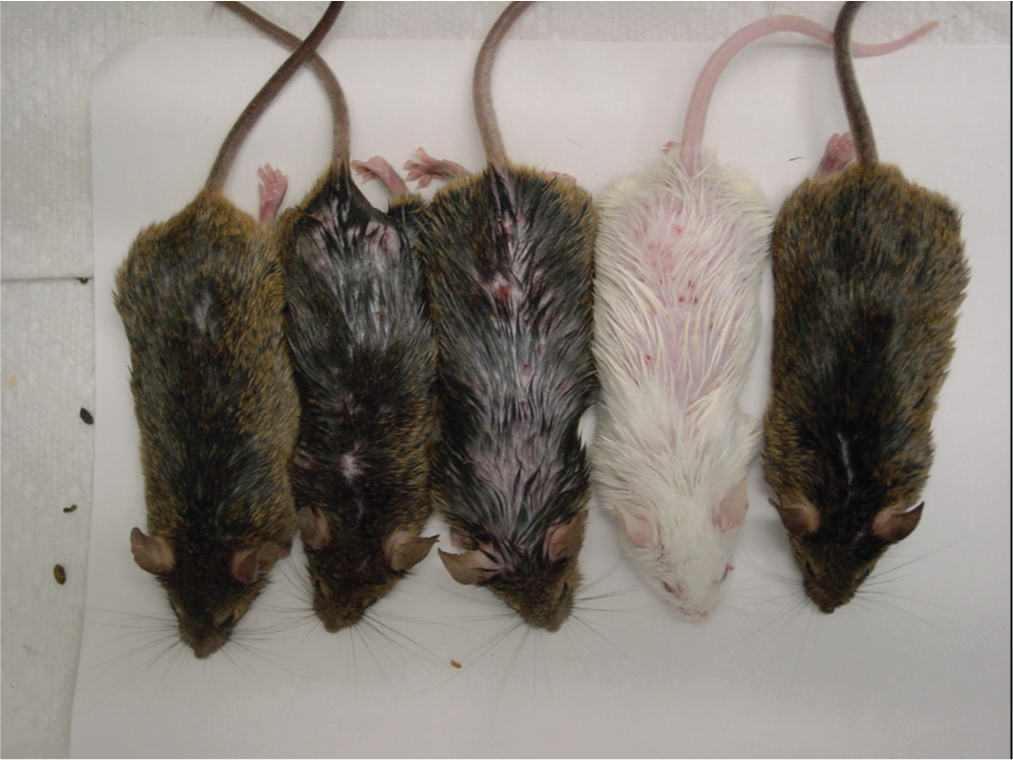
Histological characterisation of the left ventricle of Cytomegalovirus-Connective Tissue Growth Factor (CTGF) transgenic mouse as compared to wild type mouse. Eosin Y staining of heart cross section: a) littermate Wild-Type (WT) mouse; b) TG-CTGF showing left ventricle and right ventricle. The cavity of the left ventricle of TG-CTGF mice is enlarged, and the wall is thicker than that of the littermate.
*TG= Transgenic, RV= Right ventricle, LV- Left ventricle
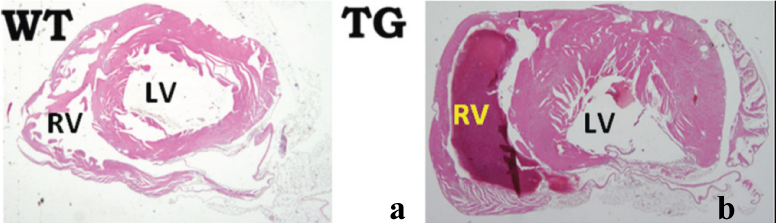
Renin staining; a) Aorta root cross section TG-Connective Tissue Growth Factor (CTGF) 10X; b) Aorta root cross section TG-CTGF 40X. Purple colour represents renin staining; c) Aorta root cross section 10X wild type mouse. Note vascular lumen remodelling in TG-CTGF mouse as compared to the wild-type mouse with a remarkable renin overexpression in aorta root in a transgenic mouse as compared to the wild-type mouse.
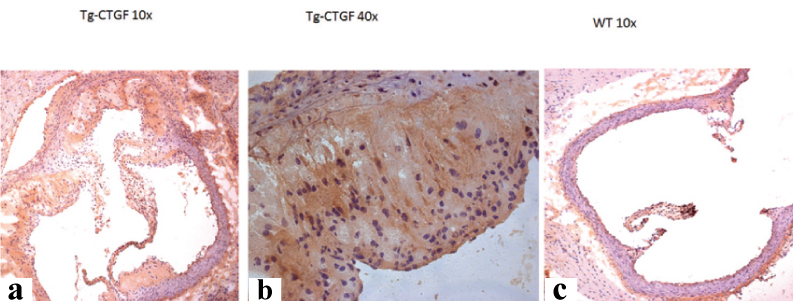
Connective Tissue Growth Factor (CTGF) staining; a) Aorta root cross section TG-CTGF 10X; b) Aorta root cross section TG-CTGF 40X. Purple colour represents CTGF staining; c) Aorta root cross section wild type mouse. Note vascular lumen remodelling as exemplified by walls thickening and CTGF overexpression in aorta root in TG-CTGF mouse as compared to the wild-type mouse.
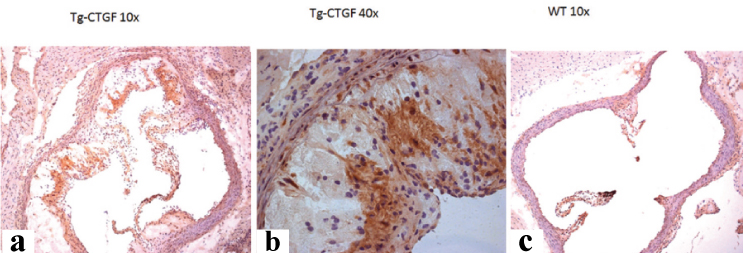
Discussion
In this study, the generation of CTGF transgenic mouse using pIRES bicistronic vector was reported. Our data suggest a potential role for CTGF the body mass control of the transgenic mouse compared with the littermate as shown in [Table/Fig-3]. CTGF gene KO mouse (CTGF-/-) die soon after birth because of multiple skeletal defects [10,20]. Fibroblasts derived from CTGF-/- KO mouse embryos have a substantially reduced expression in pro-inflammatory, pro-adhesive and pro-angiogenic genes because of diminished expression of the proteoheparansulphate subtype syndecan 4 [21]. To the best of our knowledge, this is the first time we are observing an association of CTGF regulation with the body weight control. When we opened the mouse to take other organs, we noticed that the transgenic mouse was lean with the absence of abdominal fat accumulation compared to the littermate of the same age. This study was not intended to investigate the regulation of body weight in this transgenic mouse, but interestingly, the CTGF seems have a role to play in body weight control. Immunohistochemistry analysis using littermate mouse heart as well as the transgenic mouse heart revealed that transgenic mouse undergoes cardiac hypertrophy as compared with control [Table/Fig-4]. Other authors simultaneously also reported similar findings in CTGF transgenic mouse. These studies dealt with four different tissue-specific transgenic animals with the transgenes expressed in cardiomyocytes [22], podocytes [23], hepatocytes [24] and respiratory epithelial cells [25]. Albeit the absence of glomerular abnormalities proteinuria in kidney transgenic mouse, heart tissue-specific transgenic mouse [23] showed cardiomyocyte hypertrophy and with age-dependent ventricular hypertrophy and systolic heart failure [22]. These observations confirmed our findings where the left ventricle underwent remarkable hypertrophy as compared to the wild-type mouse. The molecular mechanisms underlying this hypertrophy observed in former studies as well as in ours remained unknown [22]. A dysfunctional heart with no fibrotic process were observed in CTGF transgenic mouse where the transgene was overexpressed in cardiomyocytes. The plausible explanation of this mechanism of hypertrophy would be due to CTGF multiple trophic properties. As stated above, CTGF presents a modular structure with multiple trophic properties. Although not confirmed, it is possible that some of these modules endowed with growth factor properties will be responsible in this feature of cardiac hypertrophy. This can be elegantly demonstrated by sequential knocking out these modules and evaluate their biological properties in the induction of cardiac hypertrophy. [Table/Fig-5,6] reveal a colocalisation of CTGF and renin in aorta root, with an increased expression of renin and CTGF in the transgenic mouse compared to the control. The two figures also demonstrated that the aorta root hypertrophies in the transgenic mouse compared to the wild type. The blood vessel wall is composed of three tunicae: the intima, media and adventitia. The influence of the adventitia on vascular functions was recently recognised [26]. The structural changes in the adventitial layer, termed adventitial remodeling, have been observed in vascular disease settings such as hypertension [27], atherosclerosis [28], restenosis after balloon angioplasty arteritis and thoracic aortic dissection. Interestingly, adventitia remodelling has been shown to proceed to intima or media remodeling. Adventitial remodeling and thickening is characterised by an increase in Extracellur Matrix (ECM)protein deposition. The previous study revealed the adventitia to be the main site of collagen deposition in the aortic wall [29]. CTGF is known to mediate aortic adventitial fibroblast collagen synthesis stimulated by Angiotensin-II (Ang II) [30] and it is conceivable that, in our study, the hypertrophy and remodeling that we observed in aorta root is directly the result of collagen accumulation in adventitia and the increase in renin synthesis in the aorta root. Ang II, a potent mediator of vascular remodeling, can stimulate the synthesis of ECM in vascular cells. Ang II increased CTGF mRNA and protein expression in a time- and dose-dependent manner [30]. Since renin is involved in the production of Ang II through Ang-converting enzyme, it is obvious to say that renin is involved in the production of CTGF. Recent studies indicate that CTGF is involved in collagen synthesis stimulated by Ang II. We can see here an evolution of renin-angiotensin-aldosterone system with remarkable integration of CTGF into this axis, contributing thereby to the control of vascular tone. Besides, our model of CMV-tg-CTGF, mouse led us to an observation that CTGF can contribute positively and negatively to the biological system. In our study, overexpression of CTGF driven by CMV promoter led to cardiac and vascular hypertrophy and lean body mass production. Indeed, CTGF can be a friend and a foe, depending on the site of expression. For example, CTGF may be necessary for normal wound repair in the skin [30]. In patients with systemic sclerosis, however, overexpression of CTGF in skin fibroblasts may be disastrous [31]. Similarly, overexpression of CTGF in other organs, such as kidney, lung, or liver, may trigger pathological processes such as glomerulosclerosis, lung fibrosis, or liver cirrhosis. In atherosclerosis, high-level expression of CTGF may be responsible for ECM accumulation and thus progression of atherosclerotic lesions [32]. However, increased CTGF expression along the fibrous cap may be advantageous, i.e., increased ECM production in this area may stabilise the fibrous cap and reduce the risk of plaque rupture.
Limitation
The study was limited by the gene toxicity of the CTGF itself limiting our capability to backcross the progenies to F2 level compelling us to limit the cross at F1 level. Even at F1 level, some pups died at birth as a result of CTGF toxicity due to the presence of genome of high gene copy number. The only way to have a proper CTGF animal model is to establish a partial KO that uses a specific cassette designed through mutation of some responsive elements in the promoter region.
Conclusion
In summary, the study reveals that the overexpression of CTGF under the control of CMV promoter in mouse impacts the cardiovascular systems as well as the phenotype and the body weight of the animals. CTGF and renin colocalised in aorta root and both are overexpressed in the transgenic mouse compare to the wild type demonstrating an indirect coregulation between CTGF and renin in the vascular tone. Future work needs to be conducted aimed at developing a better strategy to backcross, these transgenic mice to develop a line with proper gene copy number/genome that can help to explore more functions.
[1]. Jun JI, Lau LF, Taking aim at the extracellular matrix: CCN proteins as emerging therapeutic targets Nat Rev Drug Discov 2011 10(12):945-63. [Google Scholar]
[2]. Hall-Glenn F, De Young RA, Huang BL, van Handel B, Hofmann JJ, Chen TT, CCN2/connective tissue growth factor is essential for pericyte adhesion and endothelial basement membrane formation during angiogenesis PLoS One 2012 7(2):1-11. [Google Scholar]
[3]. Kubota S, Takigawa M, The role of CCN2 in cartilage and bone development J Cell Commun Signal 2011 15(3):209-17. [Google Scholar]
[4]. Schober JM, Chen N, Grzeszkiewicz TM, Jovanovic I, Emeson EE, Ugarova TP, Identification of integrin alpha(M)beta(2) as an adhesion receptor on peripheral blood monocytes for Cyr61 (CCN1) and connective tissue growth factor (CCN2): Immediate-early gene products expressed in atherosclerotic lesions Blood 2002 99(12):4457-65. [Google Scholar]
[5]. Gao R, Brigstock DR, Connective tissue growth factor (CCN2) induces adhesion of rat activated hepatic stellate cells by binding of its C-terminal domain to integrin alpha(v)beta(3) and heparan sulfate proteoglycan J Biol Chem 2004 279(10):8848-55. [Google Scholar]
[6]. Segarini PR, Nesbitt JE, Li D, Hays LG, Yates JR, Carmichael DF, The low density lipoprotein receptor-related protein/alpha2-macroglobulin receptor is a receptor for connective tissue growth factor J Biol Chem 2001 276(44):40659-67. [Google Scholar]
[7]. Wahab NA, Weston BS, Mason RM, Connective tissue growth factor CCN2 interacts with and activates the tyrosine kinase receptor TrkA J Am Soc Nephrol 2005 16(2):340-51. [Google Scholar]
[8]. Aoyama E, Hattori T, Hoshijima M, Araki D, Nishida T, Kubota S, N-terminal domains of CCN family 2/connective tissue growth factor bind to aggrecan Biochem J 2009 420(3):413-20. [Google Scholar]
[9]. Hashimoto G, Inoki I, Fujii Y, Aoki T, Ikeda E, Okada Y, Matrix metalloproteinases cleave connective tissue growth factor and reactivate angiogenic activity of vascular endothelial growth factor 165 J Biol Chem 2002 277(39):36288-95. [Google Scholar]
[10]. Ivkovic S, Yoon BS, Popoff SN, Safadi FF, Libuda DE, Stephenson RC, Connective tissue growth factor coordinates chondrogenesis and angiogenesis during skeletal development Development 2003 130(12):2779-91. [Google Scholar]
[11]. Crawford LA, Guney MA, Oh YA, Deyoung RA, Valenzuela DM, Murphy AJ, Connective tissue growth factor (CTGF) inactivation leads to defects in islet cell lineage allocation and beta-cell proliferation during embryogenesis Mol Endocrinol 2009 23(3):324-36. [Google Scholar]
[12]. Nagashima T, Kim J, Li Q, Lydon JP, DeMayo FJ, Lyons KM, Connective tissue growth factor is required for normal follicle development and ovulation Mol Endocrinol 2011 25(10):1740-59. [Google Scholar]
[13]. Brigstock DR, Connective tissue growth factor (CCN2, CTGF) and organ fibrosis: Lessons from transgenic animals J Cell Commun Signal 2010 4(1):1-4. [Google Scholar]
[14]. Frazier K, Williams S, Kothapalli D, Klapper H, Grotendorst GR, Stimulation of fibroblast cell growth, matrix production, and granulation tissue formation by connective tissue growth factor J Invest Dermatol 1996 107(3):404-11. [Google Scholar]
[15]. Ellina O, Chatzigeorgiou A, Kouyanou S, Lymberi M, Mylona-Karagianni C, Tsouvalas E, Extracellular matrix-associated (GAGs, CTGF), angiogenic (VEGF) and inflammatory factors (MCP-1, CD40, IFN-γ) in Type 1 diabetes mellitus nephropathy Clin Chem Lab Med 2012 50(1):167-74. [Google Scholar]
[16]. Rees S, Coote J, Goodson S, Harris S, Lee MG, Bicistronic vector for the creation of stable mammalian cell lines that predisposes all antibiotic-resistant cells to express recombinant protein Biotechniques 1996 20(1):102-4.:106:108-10. [Google Scholar]
[17]. Jackson RJ, Howell MT, Kaminski A, The novel mechanism of initiation of picornavirus RNA translation Trends Biochem Sci 1990 15(12):477-83. [Google Scholar]
[18]. Jang SK, Krausslich HG, Nicklin MJ, Duke GM, Palmenberg AC, Wimmer E, A segment of the 5 nontranslated region of encephalomyocarditis virus RNA directs internal entry of ribosomes during in vitro translation J Virol 1988 62(8):2636-43. [Google Scholar]
[19]. He S, Jin ML, Worpel V, Hinton DR, A role for connective tissue growth factor in the pathogenesis of choroidal neovascularization Arch Ophthalmol 2003 121(9):1283-8. [Google Scholar]
[20]. Murawaki Y, Ikuta Y, Koda M, Nishimura Y, Kawasaki H, Clinical significance of serum hyaluronan in patients with chronic viral liver disease J Gastroenterol Hepatol 1996 11(5):459-65. [Google Scholar]
[21]. Kennedy L, Liu S, Shi-Wen X, Chen Y, Eastwood M, Sabetkar M, CCN2 is necessary for the function of mouse embryonic fibroblasts Exp Cell Res 2007 313(5):952-64. [Google Scholar]
[22]. Panek AN, Posch MG, Alenina N, Ghadge SK, Erdmann B, Popova E, Connective tissue growth factor overexpression in cardiomyocytes promotes cardiac hypertrophy and protection against pressure overload PLoS One 2009 4(8):e6743 [Google Scholar]
[23]. Yokoi H, Mukoyama M, Mori K, Kasahara M, Suganami T, Sawai K, Overexpression of connective tissue growth factor in podocytes worsens diabetic nephropathy in mice Kidney Int 2008 73(4):446-55. [Google Scholar]
[24]. Tong Z, Chen R, Alt DS, Kemper S, Perbal B, Brigstock DR, Susceptibility to liver fibrosis in mice expressing a connective tissue growth factor transgene in hepatocytes Hepatology 2009 50(3):939-47. [Google Scholar]
[25]. Wu S, Platteau A, Chen S, McNamara G, Whitsett J, Bancalari E, Conditional over-expression of connective tissue growth factor disrupts postnatal lung development Am J Respir Cell Mol Biol 2010 42(5):552-63. [Google Scholar]
[26]. Gutterman DD, Adventitia-dependent influences on vascular function Am J Physiol 1999 277:H1265-72. [Google Scholar]
[27]. Arribas SM, Hillier C, Gonzalez C, McGrory D, Dominiczak AF, McGrath JC, Cellular aspects of vascular remodeling in hypertension revealed by confocal microscopy Hypertension 1997 30:1455-64. [Google Scholar]
[28]. Xu C, Zaring CK, Pannaraj PS, Bassiouny HS, Glagov S, Hypercholesterolemia superimposed by experimental hypertension induces differential distribution of collagen and elastin Arterioscler Thromb Vasc Biol 2000 20(12):2566-72. [Google Scholar]
[29]. Wang X, LeMaire SA, Chen L, Shen YH, Gan Y, Bartsch H, Increased collagen deposition and elevated expression of connective tissue growth factor in human thoracic aortic dissection Circulation 2006 114(1Suppl):I200-05. [Google Scholar]
[30]. Che ZQ, Gao PJ, Shen WL, Fan CL, Liu JJ, Zhu DL, Angiotensin II-stimulated collagen synthesis in aortic adventitial fibroblasts is mediated by connective tissue growth factor Hypertens Res 2008 31(6):1233-40. [Google Scholar]
[31]. Igarashi A, Okochi H, Bradham DM, Grotendorst GR, Regulation of connective tissue growth factor gene expression in human skin fibroblasts and during wound repair Mol Biol Cell 1993 4(6):637-45. [Google Scholar]
[32]. Igarashi A, Nashiro K, Kikuchi K, Sato S, Ihn H, Grotendorst GR, Significant correlation between connective tissue growth factor gene expression and skin sclerosis in tissue sections from patients with systemic sclerosis J Invest Dermatol 1995 105(2):280-84. [Google Scholar]